22.3 Reactions of Alkenes and Alkynes
Learning Objectives
By the end of this section, you will be able to:
- Write equations for the addition reactions of alkenes and alkynes with hydrogen, halogens, and water
- Describe Markovnikov’s Rule as it applies to addition reactions
- Describe chemical tests to test for the presence of unsaturated hydrocarbons.
Addition Reactions
Alkenes are valued mainly for addition reactions, in which one of the bonds in the double bond is broken. Each of the carbon atoms in the bond can then attach another atom or group while remaining joined to each other by a single bond. Examples of addition reactions include hydrogenation, halogenation and hydration.
Hydrogenation of Alkenes
Perhaps the simplest addition reaction is hydrogenation—a reaction with hydrogen (H2) in the presence of a catalyst such as nickel (Ni) or platinum (Pt). An example of the addition reaction between ethylene and hydrogen is shown in Figure 22.3a.
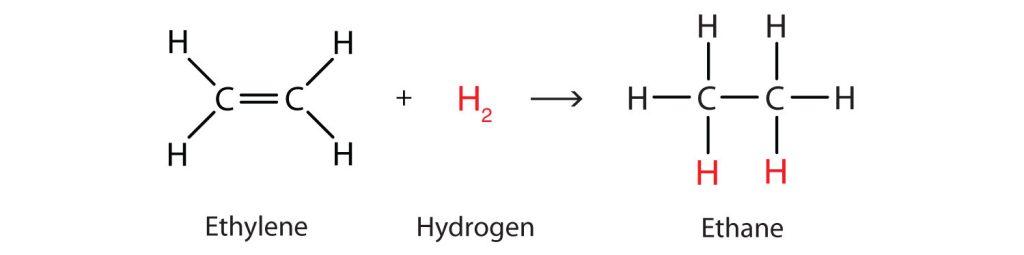
The product is an alkane having the same carbon skeleton as the alkene.
Halogenation of Alkenes
Alkenes also readily undergo halogenation—the addition of halogens. Indeed, the reaction with bromine (Br2) can be used to test for alkenes. Bromine solutions are brownish red. When we add a Br2 solution to an alkene, the colour of the solution disappears because the alkene reacts with the bromine as shown in Figure 22.3b.
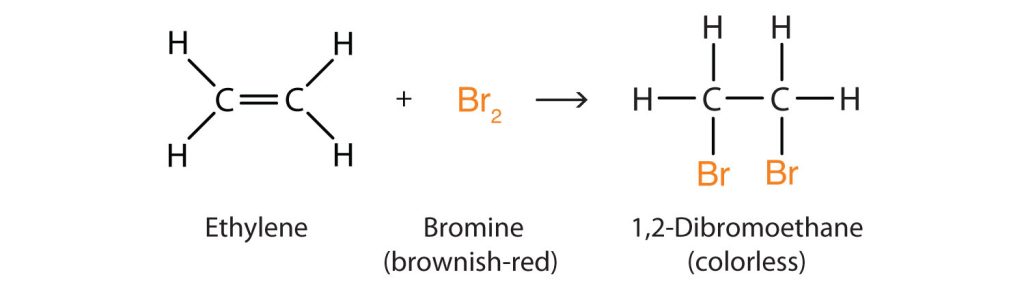
Hydration of Alkenes
Another important addition reaction is that between an alkene and water to form an alcohol. This reaction, called hydration as shown in Figure 22.3c., requires a catalyst—usually a strong acid, such as sulfuric acid (H2SO4).
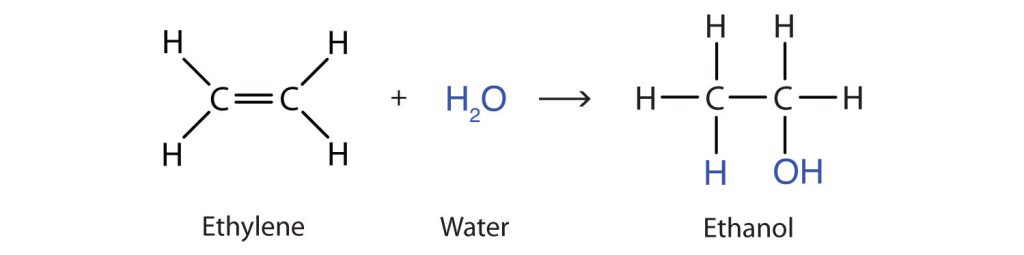
The hydration reaction is discussed in a later chapter, where we deal with this reaction in the synthesis of alcohols.
Write the equation for the reaction between CH3CH=CHCH3 and each substance.
- H2 (Ni catalyst)
- Br2
- H2O (H2SO4 catalyst)
Solution
In each reaction, the reagent adds across the double bond.
a.
b.
c.
Image credit: Intro Chemistry: GOB (V. 1.0)., CC BY-NC-SA 3.0.
Write the equation for each reaction.
- CH3CH2CH=CH2 with H2 (Ni catalyst)
- CH3CH=CH2 with Cl2
- CH3CH2CH=CHCH2CH3 with H2O (H2SO4 catalyst)
Check Your Answers:[1]
Source: Exercise 22.3a is adapted from Fundamentals of GOB Chem, CC BY-NC-SA 4.0 with answer images drawn by Samantha Sullivan Sauer / Biovia Draw, CC BY-NC 4.0
Markovnikov’s Rule
In the addition any unsymmetrical hydrogen-based molecule (e.g. HX or H2O) to an alkene, the H attaches to the carbon with fewer alkyl substituents and the X attaches to the carbon with more alkyl substituents. Examples of the rule is demonstrated in Figure 22.3d.
When both double-bonded carbon atoms have the same degree of substitution, a mixture of addition products results as demonstrated in Figure 22.3e.
Because carbocations are involved as intermediates in these electrophilic addition reactions, Markovnikov’s rule can be restated in the following way:
Markovnikov’s rule restated In the addition of HX or H2O to an alkene, the more highly substituted carbocation is formed as the intermediate rather than the less highly substituted one.
For example, in Figure 22.3f., addition of H+ to 2-methylpropene yields the intermediate tertiary carbocation rather than the alternative primary carbocation, and addition to 1-methylcyclohexene yields a tertiary cation rather than a secondary one. Why should this be?
Example 22.3b
What product would you expect from reaction of HCl with 1-ethylcyclopentene?
Strategy
When solving a problem that asks you to predict a reaction product, begin by looking at the functional group(s) in the reactants and deciding what kind of reaction is likely to occur. In the present instance, the reactant is an alkene that will probably undergo an electrophilic addition reaction with HCl. Next, recall what you know about electrophilic addition reactions to predict the product. You know that electrophilic addition reactions follow Markovnikov’s rule, so H+ will add to the double-bond carbon that has one alkyl group (C2 on the ring) and the Cl will add to the double-bond carbon that has two alkyl groups (C1 on the ring).
Solution
The expected product is 1-chloro-1-ethylcyclopentane.
Example 22.3c
Synthesizing a Specific Compound
What alkene would you start with to prepare the following alkyl halide? There may be more than one possibility.
Strategy
When solving a problem that asks how to prepare a given product, always work backward. Look at the product, identify the functional group(s) it contains, and ask yourself, “How can I prepare that functional group?” In the present instance, the product is a tertiary alkyl chloride, which can be prepared by reaction of an alkene with HCl. The carbon atom bearing the −Cl atom in the product must be one of the double-bond carbons in the reactant. Draw and evaluate all possibilities.
Solution
There are three possibilities, all of which could give the desired product according to Markovnikov’s rule.
Exercise 22.3b
Draw the major product formed from these reactions.
Check Your Answers:[2]
Activity source: Exercise 22.3b is created by Samantha Sullivan Sauer, using images from Biovia Draw, licensed under CC BY-NC 4.0
For more details on alkene addition reactions including the concept of Markonikov’s rule, watch Alkene Addition Reactions below.
Watch Alkene Addition Reactions: Crash Course Organic Chemistry #16 (youtube.com) (13 min).
Video Source: Crash Course. (2020, Nov 11). Alkene Addition Reactions: Crash Course Organic Chemistry #16 (youtube.com) [Video]. YouTube.
Polymerization
The most important commercial reactions of alkenes are polymerizations, reactions in which small molecules, referred to in general as monomers (from the Greek monos, meaning “one,” and meros, meaning “parts”), are assembled into giant molecules referred to as polymers (from the Greek poly, meaning “many,” and meros, meaning “parts”). For more information on polymerization see Chapter 27: Polymers.
Sourcing of Alkenes
In summary, recall that organic functional groups can be converted into other functional groups through reactions. To look at the sourcing of alkenes, refer to the map of some of the more common reactions to convert functional groups can be found in Section 19.6 – General Reactions of Carbon in Infographic 19.6a.
Reactions of Alkynes
Halogenation of Alkynes

Acetylene and the other alkynes also burn readily. An acetylene torch takes advantage of the high heat of combustion for acetylene.
As a general rule, electrophiles undergo addition reactions with alkynes much as they do with alkenes. Take the reaction of alkynes with HX, for instance. The reaction often can be stopped with the addition of 1 equivalent of HX, but reaction with an excess of HX leads to a dihalide product. For example, reaction of 1-hexyne with 2 equivalents of HBr yields 2,2-dibromohexane. As the following examples indicate, the regiochemistry of addition follows Markovnikov’s rule, with halogen adding to the more highly substituted side of the alkyne bond and hydrogen adding to the less highly substituted side. Trans stereochemistry of H and X normally, although not always, occurs in the product.
Bromine and chlorine also add to alkynes to give addition products, and trans stereochemistry again results as demonstrated in Figure 22.3i. below.
The mechanism of alkyne addition is similar but not identical to that of alkene addition. When an electrophile such as HBr adds to an alkene, the reaction takes place in two steps and involves an alkyl carbocation intermediate. If HBr were to add by the same mechanism to an alkyne, an analogous vinylic carbocation would be formed as the intermediate as shown in Figure 22.3j.
A vinylic carbocation has an sp-hybridized carbon and generally forms less readily than an alkyl carbocation (Figure 22.3j.). As a rule, a secondary vinylic carbocation forms about as readily as a primary alkyl carbocation, but a primary vinylic carbocation is so difficult to form that there is no clear evidence it even exists. Thus, many alkyne additions occur through more complex mechanistic pathways.
Hydration of Alkynes
Hydration of alkynes also can take place. Alkynes don’t react directly with aqueous acid but will undergo hydration readily in the presence of mercury(II) sulfate as a Lewis acid catalyst. The reaction occurs with Markovnikov regiochemistry, so the −OH group adds to the more highly substituted carbon and the −H attaches to the less highly substituted one as demonstrated in Figure 22.3k.
Interestingly, the actual product isolated from alkyne hydration is not a vinylic alcohol, or enol (ene + ol), but is instead a ketone.
Hydrogenation of Alkynes
Lastly, hydrogenation (reduction) of alkynes is another chemical reaction that can take place. Alkynes are reduced to alkanes by addition of H2 over a metal catalyst. The reaction in Figure 22.3l., occurs in two steps through an alkene intermediate, and measurements show that the first step in the reaction is more exothermic than the second.
Complete reduction to the alkane occurs when palladium on carbon (Pd/C) is used as catalyst, but hydrogenation can be stopped at the alkene stage if the less active Lindlar catalyst is used. The Lindlar catalyst is a finely divided palladium metal that has been precipitated onto a calcium carbonate support and then deactivated by treatment with lead acetate and quinoline, an aromatic amine. The hydrogenation occurs with syn stereochemistry, giving a cis alkene product.
The alkyne hydrogenation reaction has been explored extensively by the Hoffmann–LaRoche pharmaceutical company, where it is used in the commercial synthesis of vitamin A. The cis isomer of vitamin A produced initially on hydrogenation is converted to the trans isomer by heating as shown in Figure 22.3n.
An alternative method for the conversion of an alkyne to an alkene uses sodium or lithium metal as the reducing agent in liquid ammonia as solvent. This method is complementary to the Lindlar reduction because it produces trans rather than cis alkenes. For example, in Figure 22.3o., 5-decyne gives trans-5-decene on treatment with lithium in liquid ammonia.
Spotlight on Everyday Chemistry: 2022 Nobel Prize in Chemistry
Alkynes were involved in the concept of “click” chemistry where an azide is added to an alkyne with a copper catalyst allowing the two molecules to click together forming a cyclic molecule. The click chemistry concept was awarded the 2022 Nobel Prize in Chemistry to Carolyn R. Bertozzi, Morten Meldal and K. Barry Sharpless. For more information refer to infographic 22.3a.
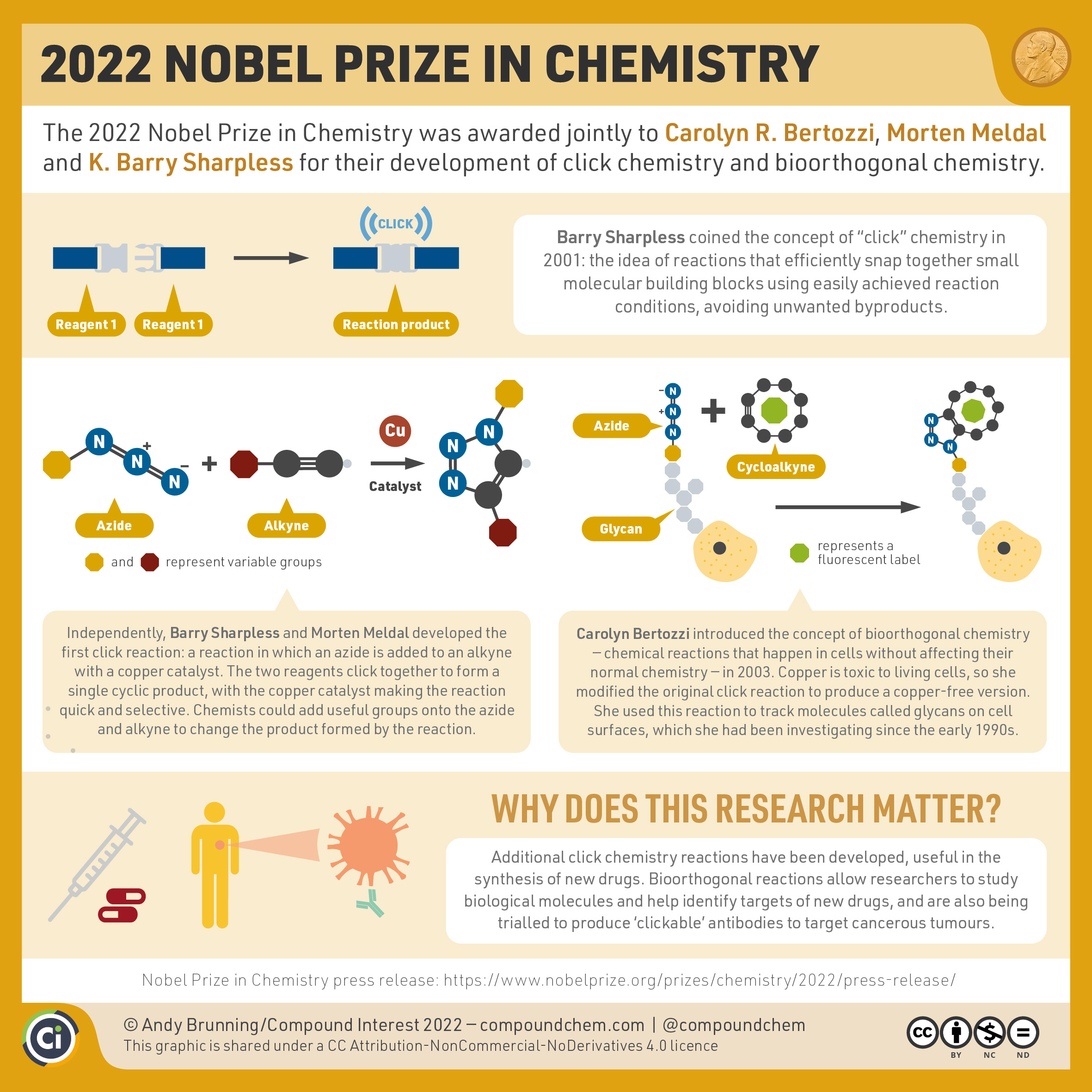
Testing for Presence of Alkenes/Alkynes
There are several ways to test for the presence of carbon-carbon double bonds and triple bonds (unsaturated hydrocarbons). One such method, as previously mentioned above, is the bromine test. Here the organic compound containing a double or triple C-C bond is mixed with an aqueous solution of bromine (or chlorine). With bromine, there is a visible colour change resulting when bromine is added to the double or triple bond (Figure 22.3p. and Figure 22.3q.). Before addition, the bromine is brownish-red. After addition the solution is colourless. If the solution stays brownish-red, it is a negative result and the compound being tested is saturated. This means there is no opportunity for addition.
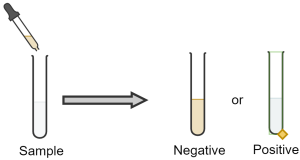

A second such test to confirm the presence of a carbon-carbon double or triple bond (unsaturated hydrocarbon) is the oxidation or permanganate test (Figure 22.3s.). This test is also known as the Baeyer test. Here, potassium permanganate, KMnO4, is used as an oxidizing agent to convert the alkene or alkyne to a diol (two alcohol functional groups in the same molecule). The visual colour change is from dark purple (permanganate solution) to dark green (manganate solution) then to black precipitate (manganese dioxide) (Figure 22.3r.). If the solution stays purple, it is a negative result and the compound being tested is saturated. This means there is no opportunity for oxidation. This test can give conflicting results in that any other components in the molecule or solution that are mildly reducing will also give a positive result.
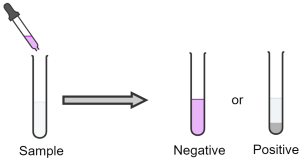
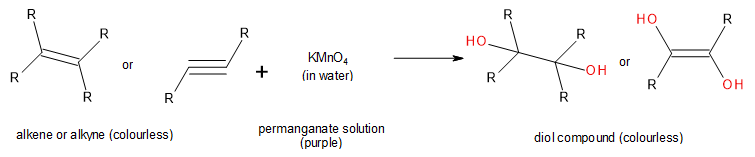
Links to Enhanced Learning
- Watch E/Z Alkenes, Electrophilic Addition, & Carbocations: Crash Course Organic Chemistry Crash Course Organic Chemistry #14 by Crash Course.
- Watch Alkene Redox Reactions Crash Course Organic Chemistry #17 by Crash Course.
- Watch Alkyne Reactions & Tautomerization Crash Course Organic Chemistry #18 by Crash Course.
Attribution & References
Except where otherwise noted, this page is written and adapted by David Wegman, Adrienne Richards and Samantha Sullivan Sauer from
- “13.6: Addition Reactions of Alkenes” and “13.7: Alkene Polymers” In Map: Fundamentals of General Organic and Biological Chemistry (McMurry et al.) by LibreTexts, licensed under CC BY-NC-SA 3.0. / A derivative of “13.4: Chemical Properties of Alkenes” In Basics of GOB (Ball et al.), CC BY-NC-SA 4.0 a LibreTexts version of Introduction to Chemistry: GOB (v. 1.0), CC BY-NC 3.0.
- “Reactions of Alkynes” section is adapted from “18.1 Hydrocarbons” In General Chemistry 1 & 2 by Rice University, a derivative of Chemistry (Open Stax) by Paul Flowers, Klaus Theopold, Richard Langley & William R. Robinson and is licensed under CC BY 4.0. Access for free at Chemistry (OpenStax)
- “7.8 Orientation of Electrophilic Additions: Markovnikov’s Rule“, “9.3 Reactions of Alkynes: Addition of HX and X2“, “9.4 Hydration of Alkynes “, and “9.5 Reduction of Alkynes” In Organic Chemistry (OpenStax) by John McMurray, CC BY-NC-SA 4.0. Access for free at Organic Chemistry (OpenStax)
- “21.1 Hydrocarbons” In Chemistry: Atoms First 2e (OpenStax) by Paul Flowers, Edward J. Neth, William R. Robinson, Klaus Theopold & Richard Langley, CC BY 4.0
- Testing for Presence of Alkenes/Alkynes section is adapted by Samantha Sullivan Sauer from “4.6.2.1: Chemistry of Manganese” by Jim Clark In Inorganic Chemistry II (CHEM4210) , CC BY-NC 4.0 and “Reactions of Alkenes with Bromine” by Jim Clark In Supplemental Modules (Organic Chemistry), CC BY-NC 4.0
Reactions involving alkenes and electrophiles. Alkenes are the neutrophiles and the electrophile is a carbon bonded to an electronegative atom such oxygen, nitrogen, sulfur or a halogen.