23.4 Reactions of Alcohols
Learning Objectives
By the end of this section, you will be able to:
- Learn about the major types of reactions of alcohols.
- Describe the result of the oxidation of a primary and secondary alcohols.
- Draw the products from dehydration of alcohols.
- Understand the production of alcohols from esters.
Organic functional groups can be converted into other functional groups through reactions. A map of some of the more common reactions to convert functional groups can be found in Section 19.6 – General Reactions of Carbon in Infographic 19.6a.
Chemical reactions in alcohols occur mainly at the functional group, but some involve hydrogen atoms attached to the OH-bearing carbon atom or to an adjacent carbon atom. We will be discussing three major kinds of alcohol reactions – substitution, elimination and oxidation.
The versatility of the alcohol functional group is well defined as it can be transformed into many other functional groups. Figure 23.4a. highlights the formation of aldehydes, ketones, carboxylic acids, ethers, alkenes, and esters.
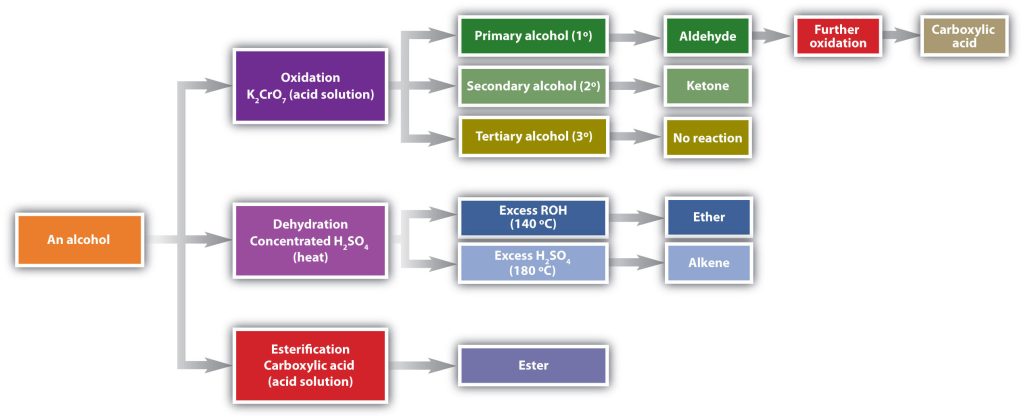
Oxidation
Primary and secondary alcohols are readily oxidized. We saw earlier how methanol and ethanol are oxidized by liver enzymes to form aldehydes. Because a variety of oxidizing agents can bring about oxidation, we can indicate an oxidizing agent without specifying a particular one by writing an equation with the symbol [O] above the arrow. For example, we write the oxidation of ethanol, a primary alcohol, to form acetaldehyde or ethanal, an aldehyde, as shown in Figure 23.4b.

We shall see that aldehydes are even more easily oxidized than alcohols and yield carboxylic acids. Secondary alcohols are oxidized to ketones. The oxidation of propan-2-ol (isopropyl alcohol) by potassium dichromate (K2Cr2O7) gives propanone (acetone), the simplest ketone (Figure 23.4c.).
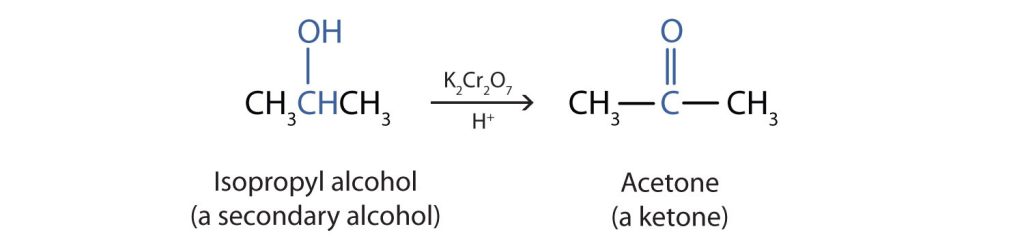
Unlike aldehydes, ketones are relatively resistant to further oxidation, so no special precautions are required to isolate them as they form. Note that in oxidation of both primary (RCH2OH) and secondary (R2CHOH) alcohols, two hydrogen atoms are removed from the alcohol molecule, one from the OH group and other from the carbon atom that bears the OH group.
Alcohol oxidation is important in living organisms. Enzyme-controlled oxidation reactions provide the energy cells need to do useful work. One step in the metabolism of carbohydrates involves the oxidation of the secondary alcohol group in isocitric acid to a ketone group (Figure 23.4d.). The overall type of reaction is the same as that in the conversion of isopropyl alcohol to acetone.
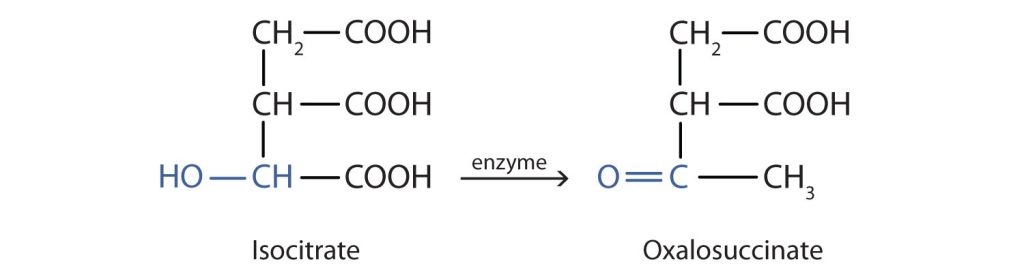
Tertiary alcohols (R3COH) are resistant to oxidation because the carbon atom that carries the OH group does not have a hydrogen atom attached but is instead bonded to other carbon atoms. The oxidation reactions we have described involve the formation of a carbon-to-oxygen double bond. Thus, the carbon atom bearing the OH group must be able to release one of its attached atoms to form the double bond. The carbon-to-hydrogen bonding is easily broken under oxidative conditions, but carbon-to-carbon bonds are not. Therefore tertiary alcohols are not easily oxidized.
The process of oxidation of alcohols is highlighted in Infographic 23.4a.
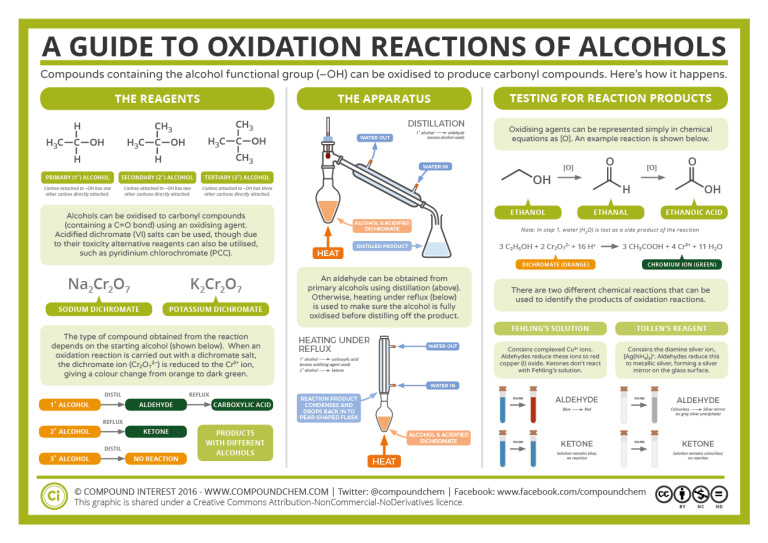
Write an equation for the oxidation of each alcohol. Use [O] above the arrow to indicate an oxidizing agent. If no reaction occurs, write “no reaction” after the arrow.
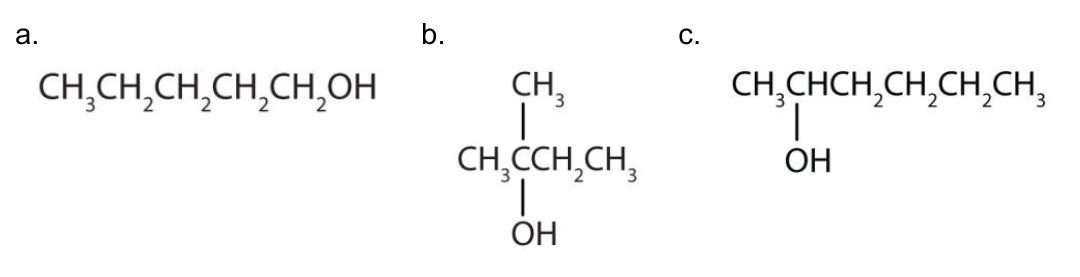
Solution
The first step is to recognize the class of each alcohol as primary, secondary, or tertiary.
- This alcohol has the OH group on a carbon atom that is attached to only one other carbon atom, so it is a primary alcohol. Oxidation forms first an aldehyde and further oxidation forms a carboxylic acid.
(Credit: Introduction to Chemistry: GOB (V. 1.0)., CC BY-NC-SA 3.0.) - This alcohol has the OH group on a carbon atom that is attached to three other carbon atoms, so it is a tertiary alcohol. No reaction occurs.
(Credit: Introduction to Chemistry: GOB (V. 1.0)., CC BY-NC-SA 3.0.) - This alcohol has the OH group on a carbon atom that is attached to two other carbon atoms, so it is a secondary alcohol; oxidation gives a ketone.
(Credit: Introduction to Chemistry: General, Organic, and Biological (V. 1.0)., CC BY-NC-SA 3.0.)
Spotlight on Everyday Chemistry: Chemistry of a Hangover
The process of alcohol oxidation is key to the effects of alcohol in the human body. Infographic 24.3b. highlights some of the chemistry of a hangover.
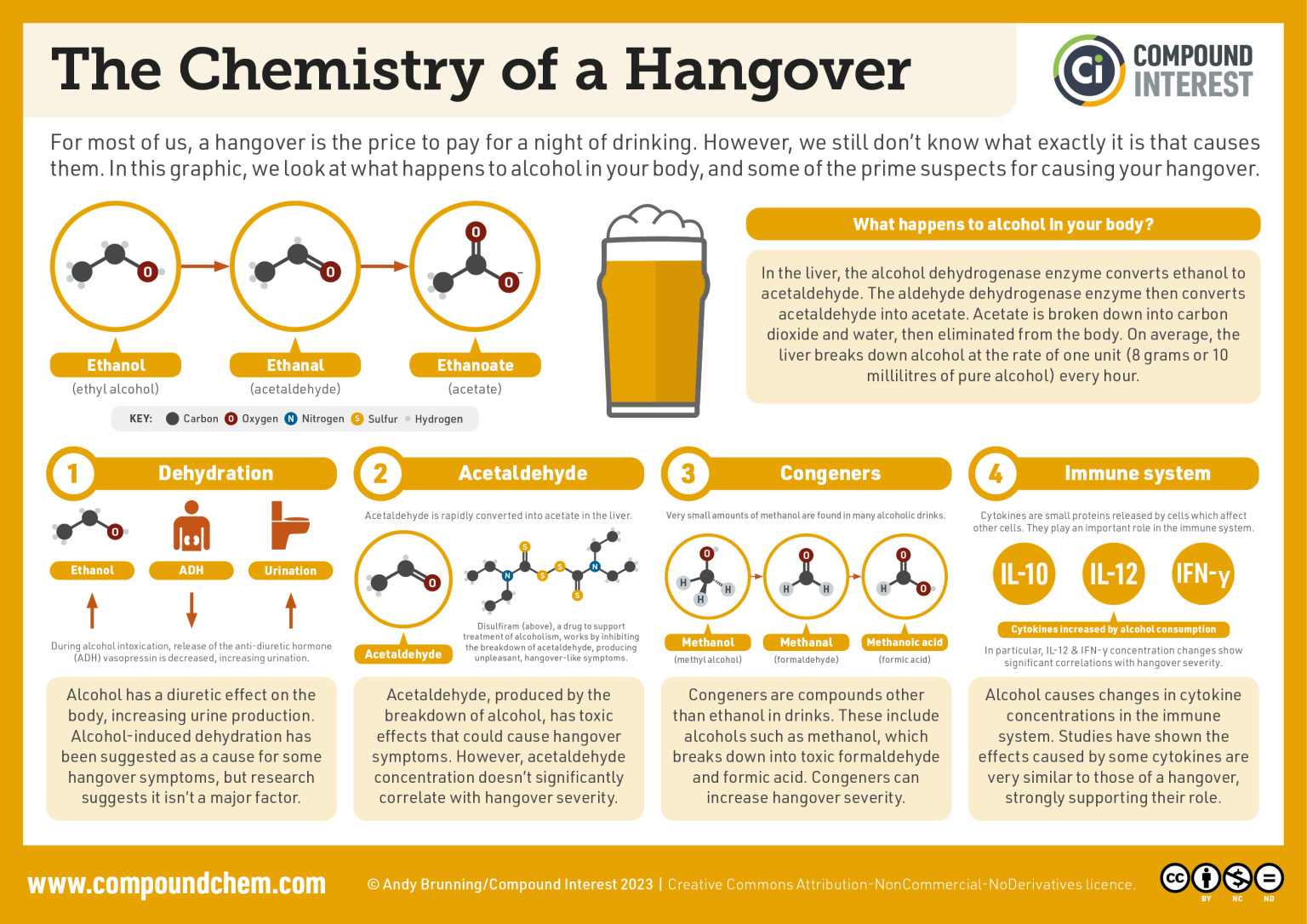
Ethanol: Chemical, Drug & Poison
Ethanol is classified medically as a central nervous system (CNS) depressant. Its effects—that is, being drunk—resemble the human response to anesthetics. There is an initial excitability and increase in sociable behavior, but this results from depression of inhibition rather than from stimulation. At a blood alcohol concentration of 0.1% to 0.3%, motor coordination is affected, accompanied by loss of balance, slurred speech, and amnesia. When blood alcohol concentration rises to between 0.3% and 0.4%, nausea and loss of consciousness occur. Above 0.6%, spontaneous respiration and cardiovascular regulation are affected, ultimately leading to death. The LD50 of ethanol is 10.6 g/kg.
The passage of ethanol through the body begins with its absorption in the stomach and small intestine, followed by rapid distribution to all body fluids and organs. In the pituitary gland, ethanol inhibits the production of a hormone that regulates urine flow, causing increased urine production and dehydration. In the stomach, ethanol stimulates production of acid. Throughout the body, ethanol causes blood vessels to dilate, resulting in flushing of the skin and a sensation of warmth as blood moves into capillaries beneath the surface. The result is not a warming of the body, but an increased loss of heat at the surface.
Ethanol metabolism occurs mainly in the liver and proceeds by oxidation in two steps, first to acetaldehyde (CH3CHO) and then to acetic acid (CH3CO2H). When continuously present in the body, ethanol and acetaldehyde are toxic, leading to the devastating physical and metabolic deterioration seen in people with chronic alcohol use disorder. The liver usually suffers the worst damage since it is the major site of alcohol metabolism.
Approximately 17,000 people are killed each year in the United States in alcohol-related automobile accidents. Thus, all 50 states have made it illegal to drive with a blood alcohol concentration (BAC) above 0.08%. Fortunately, simple tests have been devised for measuring blood alcohol concentration. The original breath analyzer test measured alcohol concentration in expired air by the colour change occurring when the bright-orange oxidizing agent potassium dichromate (K2Cr2O7) reduced to blue-green chromium(III). Current consumer devices use a conductivity sensor, and tests used by law-enforcement agencies use IR spectroscopy to measure blood-alcohol levels in expired air. Just breathe into the machine, and let the spectrum tell the tale.
Source: “Ethanol: Chemical, Drug & Poison” is adapted from “Ch. 17 Chemistry Matters—Ethanol: Chemical, Drug, and Poison” In Organic Chemistry (OpenStax), CC BY-NC-SA 4.0.
Write an equation for the oxidation of each alcohol. Use [O] above the arrow to indicate an oxidizing agent. If no reaction occurs, write “no reaction” after the arrow.
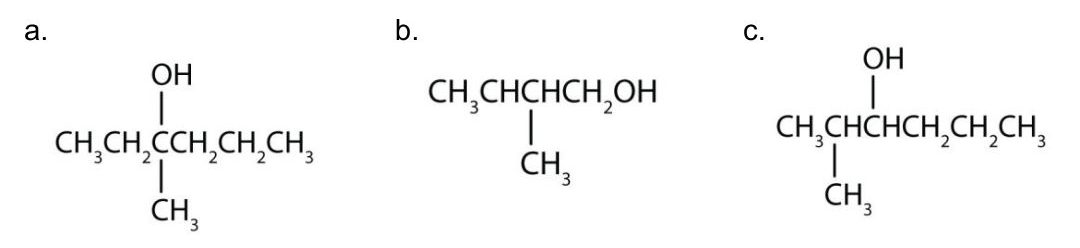
Check Your Answers:[1]
Source: Exercise 23.4a is adapted from Introduction to Chemistry: GOB (V. 1.0)., CC BY-NC-SA 3.0 with solution images by Samantha Sullivan Sauer using Biovia Draw.
Elimination – Dehydration Reactions
Formation of Alkenes
As noted in Figure 24.3a., an alcohol undergoes dehydration in the presence of a catalyst to form an alkene and water or an ether and water. It all depends on the conditions.
To form an alkene, the reaction requires a higher temperature and limited supply of alcohol. The reaction removes the OH group from the alcohol carbon atom and a hydrogen atom from an adjacent carbon atom in the same molecule (Figure 24.3e.):
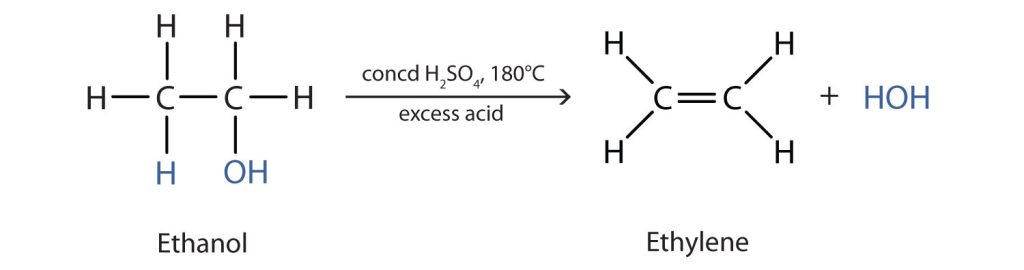
The required dehydration reaction temperature is dependent on the classification of the starting alcohol. The needed temperature decreases with increasing substitution of the hydroxy-containing carbon:
- 1° alcohols: 170° – 180°C
- 2° alcohols: 100°– 140 °C
- 3° alcohols: 25°– 80°C
If the reaction is not sufficiently heated, the alcohols do not dehydrate to form alkenes, but react with one another to form ethers.
Example 23.4b
Draw the product of the dehydration of cyclohexanol.
Solution:
Cyclohexene is formed.
Example and image source: Supplemental Modules (Organic Chemistry), CC BY 4.0
Zaitsev’s Rule
When an unsymmetrical alcohol undergoes dehydration to form an alkene, Zaitsev’s rule applies. According to Zaitsev’s rule, formulated in 1875 by the Russian chemist Alexander Zaitsev, elimination reactions generally (although not always) give the more stable alkene product—that is, the alkene with more alkyl substituents on the double-bond carbons. Another way to say this is the more highly-substituted double bond isomer is favoured among the products.
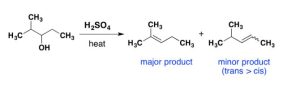
Exercise 24.3b
Draw the major product of the given reactions.
Check Your Answers:[2]
Source: Exercise 24.3b is adapted from Organic Chemistry (Wade), images created by Samantha Sullivan Sauer using Biovia Draw, licensed under CC BY-NC-SA 4.0.
Formation of Ethers
Under the proper conditions (lower temperature and excess alcohol), it is possible for the dehydration to occur between two small primary alcohol molecules. The entire OH group of one molecule and only the hydrogen atom of the OH group of the second molecule are removed. The two ethyl groups attached to an oxygen atom form an ether molecule (Figure 23.4g.). Only symmetrical ethers can be prepared this way.

Example 23.4c
Write the two potential reactions from the dehydration of ethanol.
Solution:
The success of the dehydration reaction is dependent on the temperature and the amount of alcohol present. The two possible reactions are shown. You are not responsible for knowing the temperatures required.
Both reactions are dehydration reactions using sulfuric acid and result in a by-product of water. The first reaction (at lower temperature) combines two ethanol molecules to form diethyl ether (ethoxyethane). The second reaction (at higher temperature and limited alcohol) dehydrates intramolecularly resulting in ethene.
Example and image source: Synthesis of Ethers, CC BY-NC-SA 4.0
Both dehydration and hydration reactions occur continuously in cellular metabolism, with enzymes serving as catalysts and at a temperature of about 37°C. The following reaction occurs in the “Embden–Meyerhof” pathway (Figure 23.4h.).
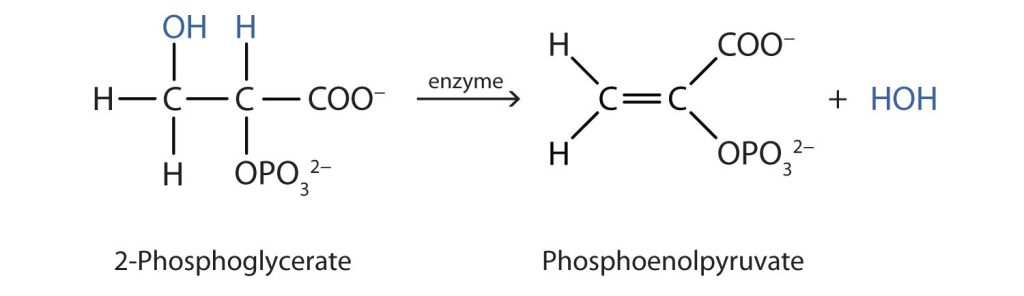
Although the participating compounds are complex, the reaction is the same: elimination of water from the starting material. The idea is that if you know the chemistry of a particular functional group, you know the chemistry of hundreds of different compounds.
Esterification
Carboxylic acids can react with alcohols to form esters in a process called Fischer esterification. An acid catalyst is required and the alcohol is also used as the reaction solvent. The oxygen atoms are colour-coded in the reaction below to help understand the reaction mechanism.

For example, butanoic acid reacts with methanol to synthesize methyl butanoate. It is important to note that any proton source can be used as the catalyst. Sulfuric acid is shown in the example (Figure 23.4j.).

Further details about esters and carboxylic acids are provided in Chapter 25.
Substitution of Alcohols
Although substitution of alcohols is not listed in Figure 23.4a., it is possible to form an alkyl halide from an alcohol.
When alcohols react with a hydrogen halide, a substitution takes place producing an alkyl halide and water:
- The order of reactivity of alcohols is 3° > 2° > 1° methyl.
- The order of reactivity of the hydrogen halides is HI > HBr > HCl (HF is generally unreactive).
The reaction is acid catalyzed. Alcohols react with the strongly acidic hydrogen halides HCl, HBr, and HI, but they do not react with nonacidic NaCl, NaBr, or NaI. Primary and secondary alcohols can be converted to alkyl chlorides and bromides by allowing them to react with a mixture of a sodium halide and sulfuric acid:
Source: “17.6: Reactions of Alcohols” from Organic Chemistry (Morsch et al) is used under CC BY-SA 4.0.
Video Source: Crash Course. (2021, March 17). Alcohols, Ethers, and Epoxides: Crash Course Organic Chemistry #24 [Video]. YouTube.
Attribution & References
Except where otherwise noted, this page is written and adapted by David Wegman and Samantha Sullivan Sauer from
- “14.5: Reactions of Alcohols” In Basics of General, Organic, and Biological Chemistry (Ball et al.) by David W. Ball, John W. Hill, and Rhonda J. Scott via LibreTexts, CC BY-NC-SA 4.0. / A derivative of Introduction to Chemistry: GOB (v. 1.0), CC BY-NC 3.0.
- “14.4: Dehydration Reactions of Alcohols” by Jeffery Ma In Map: Organic Chemistry (Wade), Complete and Semesters I and II, CC BY-NC-SA 4.0
- “Substitution – Conversion of Alcohols into Alkyl Halides” section is adapted from “17.6: Reactions of Alcohols” by Steven Farmer, Dietmar Kennepohl, Layne Morsch, James Kabrhel In Organic Chemistry (Morsch et al.), CC BY-SA 4.0.
- “14.4: Reactions of Alcohols” In Map: Fundamentals of General Organic and Biological Chemistry (McMurry et al.), CC BY-NC-SA 3.0. / A derivative of Basics of General, Organic, and Biological Chemistry (Ball et al.)by David W. Ball, John W. Hill, and Rhonda J. Scott via LibreTexts, CC BY-NC-SA 4.0., which is a LibreTexts version of Introduction to Chemistry: GOB (v. 1.0), CC BY-NC 3.0.
- “Ethanol: Chemical, Drug & Poison” is adapted from “Ch. 17 Chemistry Matters—Ethanol: Chemical, Drug, and Poison” In Organic Chemistry (OpenStax) by John McMurray, CC BY-NC-SA 4.0.
- “11.7 Elimination Reactions: Zaitsev’s Rule” In Organic Chemistry (OpenStax) by John McMurray, CC BY-NC-SA 4.0.
- “Esterification” section is adapted “21.6: Condensation of Acids with Alcohols” by Dr. Dietmar Kennepohl, and Prof. Steven Farmer. In Map: Organic Chemistry (Wade), Complete and Semesters I and II, CC BY-NC-SA 4.0. Attributions from original source: Organic Chemistry With a Biological Emphasis by Tim Soderberg (University of Minnesota, Morris)
- “Elimination Reactions of Alcohols” and “Dehydration of Alcohols to Make Ethers” by William Reusch In Supplemental Modules (Organic Chemistry), CC BY-NC-SA 4.0
- “Alkenes by Dehydration of Alcohols” by Binod Shrestha, In Supplemental Modules (Organic Chemistry), CC BY 4.0