20.1 Characteristics of Alkanes
Learning Objectives
By the end of this section, you will be able to:
- Understand carbon-hydrogen bonding
- Identify and name simple (straight-chain) alkanes given formulas and write formulas for straight-chain alkanes given their names.
- Identify the physical properties of alkanes and describe trends in these properties.
Alkanes
We begin our study of organic chemistry with the hydrocarbons, the simplest organic compounds, which are composed of carbon and hydrogen atoms only. As we noted, there are several different kinds of hydrocarbons. They are distinguished by the types of bonding between carbon atoms and the properties that result from that bonding.
The bonding in the hydrogen molecule is fairly straightforward, but the situation is more complicated in organic molecules with tetravalent carbon atoms. Take methane, CH4, for instance. As we’ve seen, carbon has four valence electrons (2s2 2p2) and forms four bonds. Because carbon uses two kinds of orbitals for bonding, 2s and 2p, we might expect methane to have two kinds of C–H bonds. In fact, though, all four C–H bonds in methane are identical and are spatially oriented toward the corners of a regular tetrahedron as shown in Figure 20.1a. How can we explain this?
An answer was provided in 1931 by Linus Pauling, who showed mathematically how an s orbital and three p orbitals on an atom can combine, or hybridize, to form four equivalent atomic orbitals with tetrahedral orientation. Shown in Figure 20.1b., these tetrahedrally oriented orbitals are called sp3 hybrid orbitals. Note that the superscript 3 in the name sp3 tells how many of each type of atomic orbital combine to form the hybrid, not how many electrons occupy it.
The concept of hybridization explains how carbon forms four equivalent tetrahedral bonds but not why it does so. The shape of the hybrid orbital suggests the answer to why. When an s orbital hybridizes with three p orbitals, the resultant sp3 hybrid orbitals are unsymmetrical about the nucleus. One of the two lobes is larger than the other and can therefore overlap more effectively with an orbital from another atom to form a bond. As a result, sp3 hybrid orbitals form stronger bonds than do unhybridized s or p orbitals.
The asymmetry of sp3 orbitals arises because, as noted previously, the two lobes of a p orbital have different algebraic signs, + and –, in the wave function. Thus, when a p orbital hybridizes with an s orbital, the positive p lobe adds to the s orbital but the negative p lobe subtracts from the s orbital. The resultant hybrid orbital is therefore unsymmetrical about the nucleus and is strongly oriented in one direction.
When each of the four identical sp3 hybrid orbitals of a carbon atom overlaps with the 1s orbital of a hydrogen atom, four identical C–H bonds are formed and methane results. Each C–H bond in methane has a strength of 439 kJ/mol (105 kcal/mol) and a length of 109 pm. Because the four bonds have a specific geometry, we also can define a property called the bond angle. The angle formed by each H–C–H is 109.5°, the so-called tetrahedral angle. Methane thus has the structure shown in Figure 20.1c.
The flat representations shown do not accurately portray bond angles or molecular geometry. Methane has a tetrahedral shape that chemists often portray with wedges indicating bonds coming out toward you and dashed lines indicating bonds that go back away from you. An ordinary solid line indicates a bond in the plane of the page. Recall that the VSEPR theory correctly predicts a tetrahedral shape for the methane molecule (Figure 20.1d.).
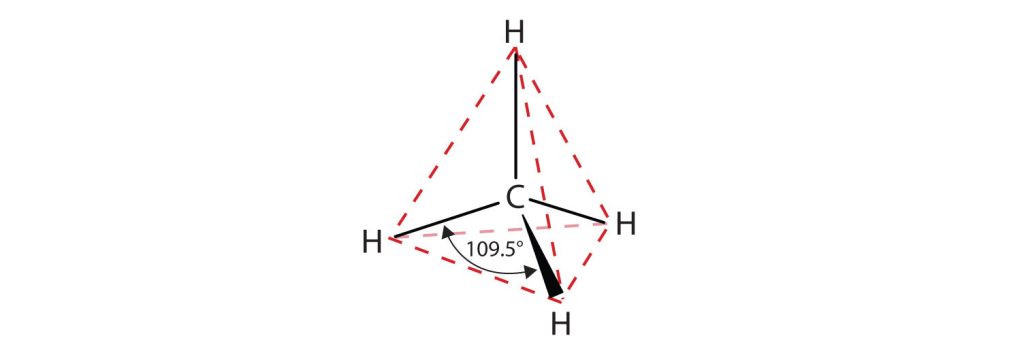
Exercise 20.1a
By using the PhET Interactive Simulation, view the CH4 molecule by selecting the Real Molecules option. From the drop-down menu, select the compound CH4 then select Show Lone Pairs, Bond Angles, Electron Geometry, and Molecular Geometry. Alternatively, you can build your own CH4 molecule by selecting the Model option.
Practice using the following PhET simulation: Molecule Shapes [New tab]
Hydrocarbons with only carbon-to-carbon single bonds (C–C) and existing as a continuous chain of carbon atoms also bonded to hydrogen atoms are called alkanes (or saturated hydrocarbons). Saturated, in this case, means that each carbon atom is bonded to four other atoms (hydrogen or carbon)—the most possible; there are no double or triple bonds in the molecules. The word saturated has the same meaning for hydrocarbons as it does for the dietary fats and oils: the molecule has no carbon-to-carbon double bonds (C=C).
The three simplest alkanes—methane (CH4), ethane (C2H6), and propane (C3H8) and they are shown in Figure 20.1e.
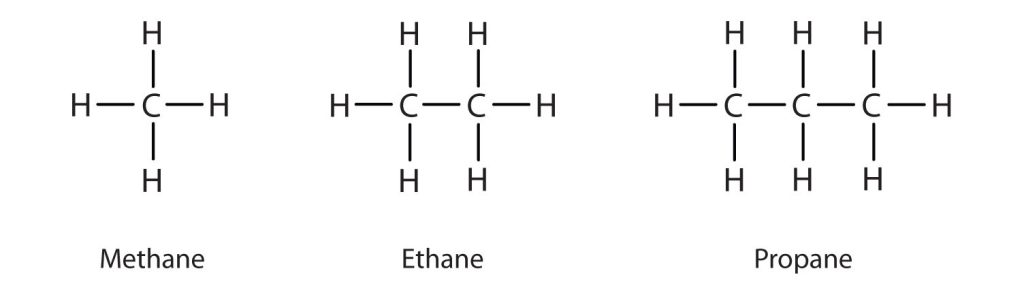
Methane (CH4), ethane (C2H6), and propane (C3H8) are the beginning of a series of compounds in which any two members in a sequence differ by one carbon atom and two hydrogen atoms—namely, a CH2 unit. The first 10 members of this series are given in Table 20.1a.
Table source: “12.2: Structures and Names of Alkanes” In Basics of GOB Chemistry (Ball et al.), CC BY-NC-SA 4.0.
Consider the series in Figure 20.1f. The sequence starts with C3H8, and a CH2 unit is added in each step moving up the series. Any family of compounds in which adjacent members differ from each other by a definite factor (here a CH2 group) is called a homologous series. The members of such a series, called homologs, have properties that vary in a regular and predictable manner. The principle of homology gives organization to organic chemistry in much the same way that the periodic table gives organization to inorganic chemistry. Instead of a bewildering array of individual carbon compounds, we can study a few members of a homologous series and from them deduce some of the properties of other compounds in the series.
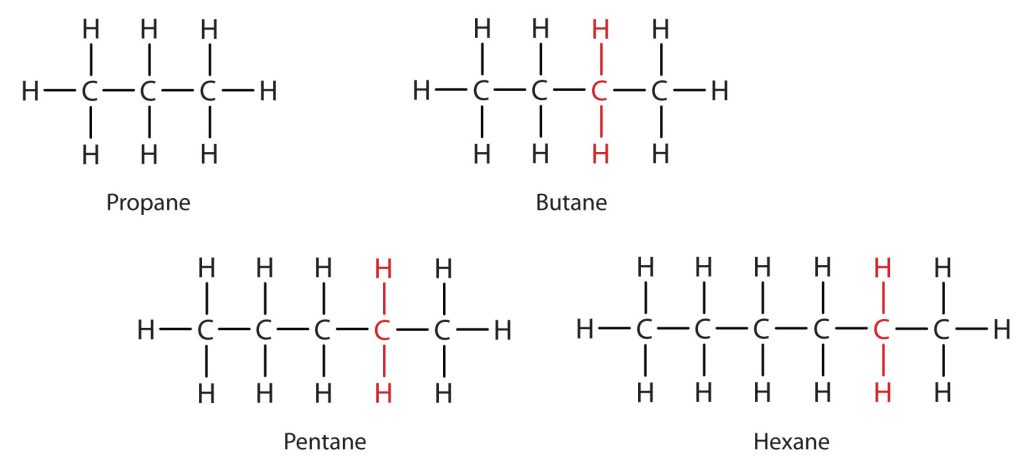
The principle of homology allows us to write a general formula for alkanes: CnH2n + 2. Using this formula, we can write a molecular formula for any alkane with a given number of carbon atoms. For example, an alkane with eight carbon atoms has the molecular formula C8H(2 × 8) + 2 = C8H18.
The video What is Organic Chemistry below gives an introduction to alkanes.
Watch What Is Organic Chemistry?: Crash Course Organic Chemistry #1 – YouTube (10 min)
Video source: Crash Course. (2020, April 30). What Is Organic Chemistry?: Crash Course Organic Chemistry #1 – YouTube [Video]. YouTube.
Physical Properties of Alkanes
Because alkanes have relatively predictable physical properties and undergo relatively few chemical reactions other than combustion, they serve as a basis of comparison for the properties of many other organic compound families. Let’s consider their physical properties first.
Table 20.1b. describes some of the properties of some of the first 10 straight-chain alkanes. Because alkane molecules are nonpolar, they are insoluble in water, which is a polar solvent, but are soluble in nonpolar and slightly polar solvents. Consequently, alkanes themselves are commonly used as solvents for organic substances of low polarity, such as fats, oils, and waxes. Nearly all alkanes have densities less than 1.0 g/mL and are therefore less dense than water (the density of H2O is 1.00 g/mL at 20°C). These properties explain why oil and grease do not mix with water but rather float on its surface as demonstrated in Figure 20.1g.
Molecular Name | Formula | Melting Point (°C) | Boiling Point (°C) | Density (20°C)* | Physical State (at 20°C) |
---|---|---|---|---|---|
methane | CH4 | –182 | –164 | 0.668 g/L | gas |
ethane | C2H6 | –183 | –89 | 1.265 g/L | gas |
propane | C3H8 | –190 | –42 | 1.867 g/L | gas |
butane | C4H10 | –138 | –1 | 2.493 g/L | gas |
pentane | C5H12 | –130 | 36 | 0.626 g/mL | liquid |
hexane | C6H14 | –95 | 69 | 0.659 g/mL | liquid |
octane | C8H18 | –57 | 125 | 0.703 g/mL | liquid |
decane | C10H22 | –30 | 174 | 0.730 g/mL | liquid |
*Note the change in units going from gases (grams per liter) to liquids (grams per milliliter). Gas densities are at 1 atm pressure. |
Table source: “12.6: Physical Properties of Alkanes” In Basics of GOB Chemistry (Ball et al.), CC BY-NC-SA 4.0.
Table 20.1b. indicates that the first four members of the alkane series are gases at ordinary temperatures. Natural gas is composed chiefly of methane, which has a density of about 0.67 g/L. The density of air is about 1.29 g/L. Because natural gas is less dense than air, it rises. When a natural-gas leak is detected and shut off in a room, the gas can be removed by opening an upper window. On the other hand, bottled gas can be either propane (density 1.88 g/L) or butanes (a mixture of butane and isobutane; density about 2.5 g/L). Both are much heavier than air (density 1.2 g/L). If bottled gas escapes into a building, it collects near the floor. This presents a much more serious fire hazard than a natural-gas leak because it is more difficult to rid the room of the heavier gas.
Also shown in Table 20.1b are the boiling points of the straight-chain alkanes increase with increasing molar mass. This general rule holds true for the straight-chain homologs of all organic compound families. Larger molecules have greater surface areas and consequently interact more strongly; more energy is therefore required to separate them. For a given molar mass, the boiling points of alkanes are relatively low because these nonpolar molecules have only weak dispersion forces to hold them together in the liquid state.
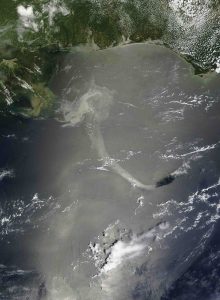
Spotlight on Everyday Chemistry: Characteristics of Alkanes
Hydrocarbons are the simplest organic compounds, but they have interesting physiological effects. These effects depend on the size of the hydrocarbon molecules and where on or in the body they are applied. Alkanes of low molar mass—those with from 1 to approximately 10 or so carbon atoms—are gases or light liquids that act as anesthetics. Inhaling (“sniffing”) these hydrocarbons in gasoline or aerosol propellants for their intoxicating effect is a major health problem that can lead to liver, kidney, or brain damage or to immediate death by asphyxiation by excluding oxygen.
Swallowed, liquid alkanes do little harm while in the stomach. In the lungs, however, they cause “chemical” pneumonia by dissolving fatlike molecules from cell membranes in the tiny air sacs (alveoli). The lungs become unable to expel fluids, just as in pneumonia caused by bacteria or viruses. People who swallow gasoline or other liquid alkane mixtures should not be made to vomit, as this would increase the chance of getting alkanes into the lungs. (There is no home-treatment antidote for gasoline poisoning; call a poison control center.)
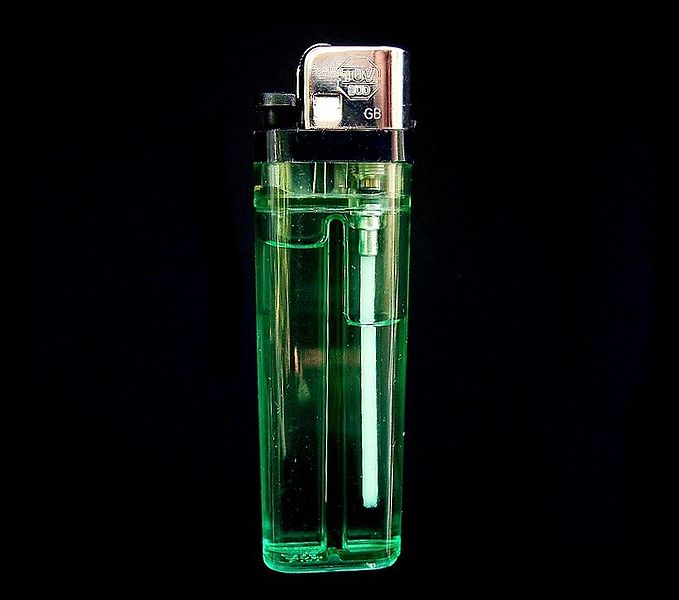
Liquid alkanes with approximately 5–16 carbon atoms per molecule wash away natural skin oils and cause drying and chapping of the skin, while heavier liquid alkanes (those with approximately 17 or more carbon atoms per molecule) act as emollients (skin softeners). Such alkane mixtures as mineral oil and petroleum jelly can be applied as a protective film. Water and aqueous solutions such as urine will not dissolve such a film, which explains why petroleum jelly protects a baby’s tender skin from diaper rash.
Attribution & References
Except where otherwise noted, this page is adapted by Adrienne Richards from:
- "12.0: Prelude to Organic Chemistry - Alkanes and Halogenated Hydrocarbons," "12.2: Structures and Names of Alkanes," "12.6: Physical Properties of Alkanes" and "12.7: Chemical Properties of Alkanes" In Basics of General, Organic, and Biological Chemistry (Ball et al.) by David W. Ball, John W. Hill, and Rhonda J. Scott (LibreTexts), licensed under CC BY-NC-SA 4.0. / A derivative of Introduction to Chemistry: General, Organic, and Biological (V. 1.0), CC BY-NC-SA 3.0. / Content from individual pages has been remixed into this one page. Tables and images are attributed individually.
- Except where otherwise noted, content from the 2nd paragraph under Alkanes heading through Figure 20.1 c is adapted from "1.6 sp3 Hybrid Orbitals and the Structure of Methane" In Organic Chemistry (OpenStax) by John McMurray, licensed under CC BY-NC-SA 4.0. Access for free at Organic Chemistry (OpenStax)
- Figure 20.1a is adapted from "1.4 Development of Chemical Bonding Theory" In Organic Chemistry (OpenStax) by John McMurray, licensed under CC BY-NC-SA 4.0. Access for free at Organic Chemistry (OpenStax)