20.6 Reactions of Alkanes
Learning Objectives
By the end of this section, you will be able to:
- Understand the reactions of alkanes: combustion and substitution.
Reactions of Alkanes
Alkane molecules are nonpolar and therefore generally do not react with ionic compounds such as most laboratory acids, bases, oxidizing agents, or reducing agents. Consider butane as an example in Figure 20.6a.
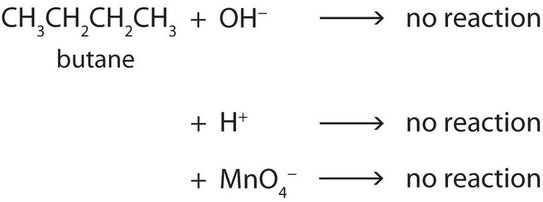
Neither positive ions nor negative ions are attracted to a nonpolar molecule. In fact, the alkanes undergo so few reactions that they are sometimes called paraffins, from the Latin parum affinis, meaning “little affinity.”
However, heat or light can initiate the breaking of C–H or C–C single bonds in reactions called combustion and substitution.
Watch Alkanes: Crash Course Organic Chemistry #6 – YouTube (12 min)
Video Source: Crash Course. (2020, June 24). Alkanes: Crash Course Organic Chemistry #6 [Video]. YouTube.
Recall that organic functional groups can be converted into other functional groups through reactions. A map of some of the more common reactions to convert functional groups can be found in Section 19.6 – General Reactions of Carbon in Infographic 19.6a.
Combustion
Alkanes are relatively stable molecules, but heat or light will activate reactions that involve the breaking of C–H or C–C single bonds. Combustion is one such reaction:
Alkanes burn in the presence of oxygen, a highly exothermic oxidation-reduction reaction that produces carbon dioxide and water. As a consequence, alkanes are excellent fuels. For example, methane, CH4, is the principal component of natural gas. Butane, C4H10, used in camping stoves and lighters is an alkane. Gasoline is a liquid mixture of continuous- and branched-chain alkanes, each containing from five to nine carbon atoms, plus various additives to improve its performance as a fuel. Kerosene, diesel oil, and fuel oil are primarily mixtures of alkanes with higher molecular masses. The main source of these liquid alkane fuels is crude oil, a complex mixture that is separated by fractional distillation. Fractional distillation takes advantage of differences in the boiling points of the components of the mixture (see Figure 20.6b.). You may recall that boiling point is a function of intermolecular interactions.
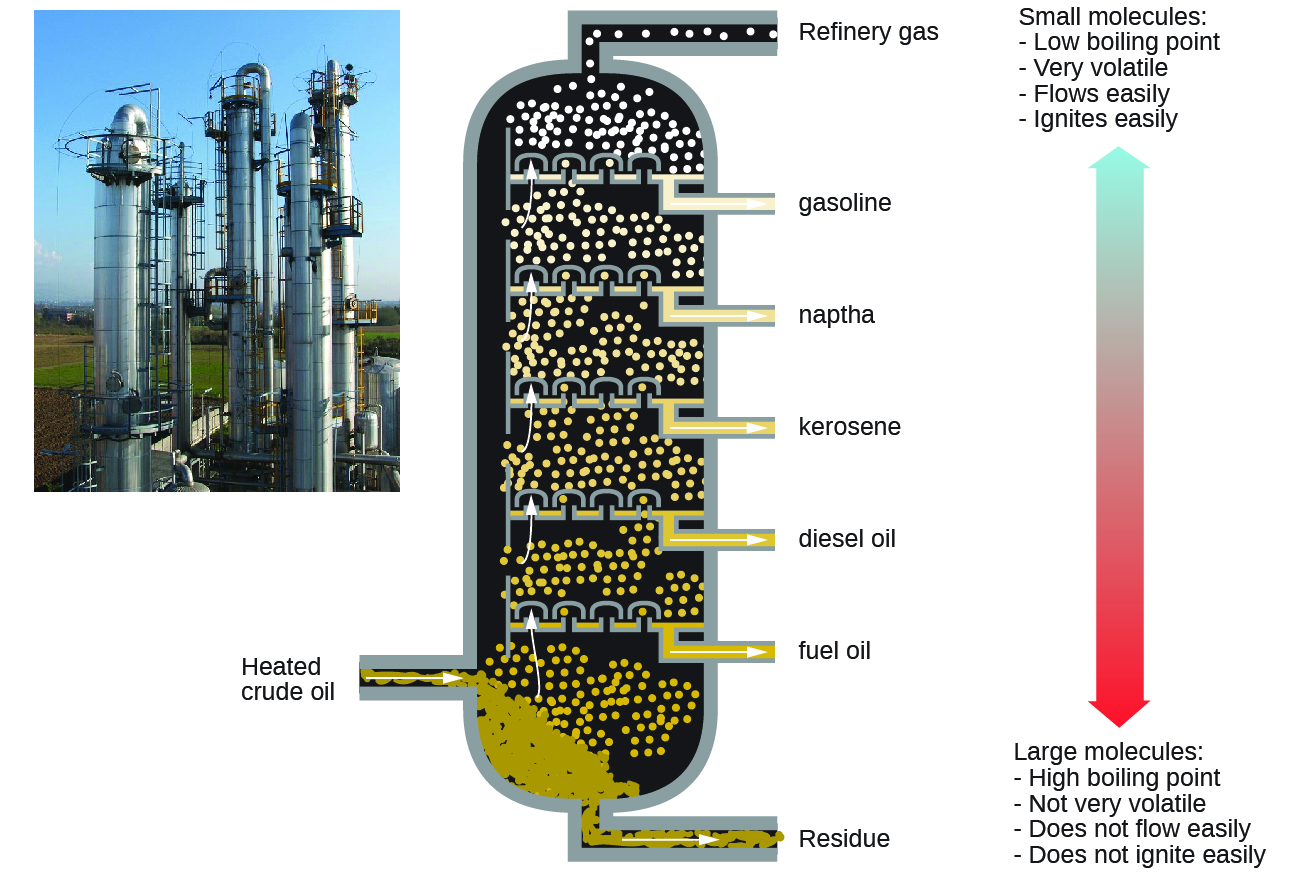
If the reactants of combustion reactions are adequately mixed, and there is sufficient oxygen, the only products are carbon dioxide (CO2), water (H2O), and energy—heat for cooking foods, heating homes, and drying clothes. Because conditions are rarely ideal, other unwanted by-products are frequently formed. When the oxygen supply is limited, carbon monoxide (CO) is a by-product:
\[2CH_4 + 3O_2 \rightarrow 2CO + 4H_2O\label{2} \]
This reaction is responsible for dozens of deaths each year from unventilated or improperly adjusted gas heaters. (Similar reactions with similar results occur with kerosene heaters.)
Spotlight on Everyday Chemistry: Fuel
We use fuel (or petrol in the UK) in our vehicles everyday. Fuel comes from fossil fuels. Read more about how fuel works in Infographic 20.6a.
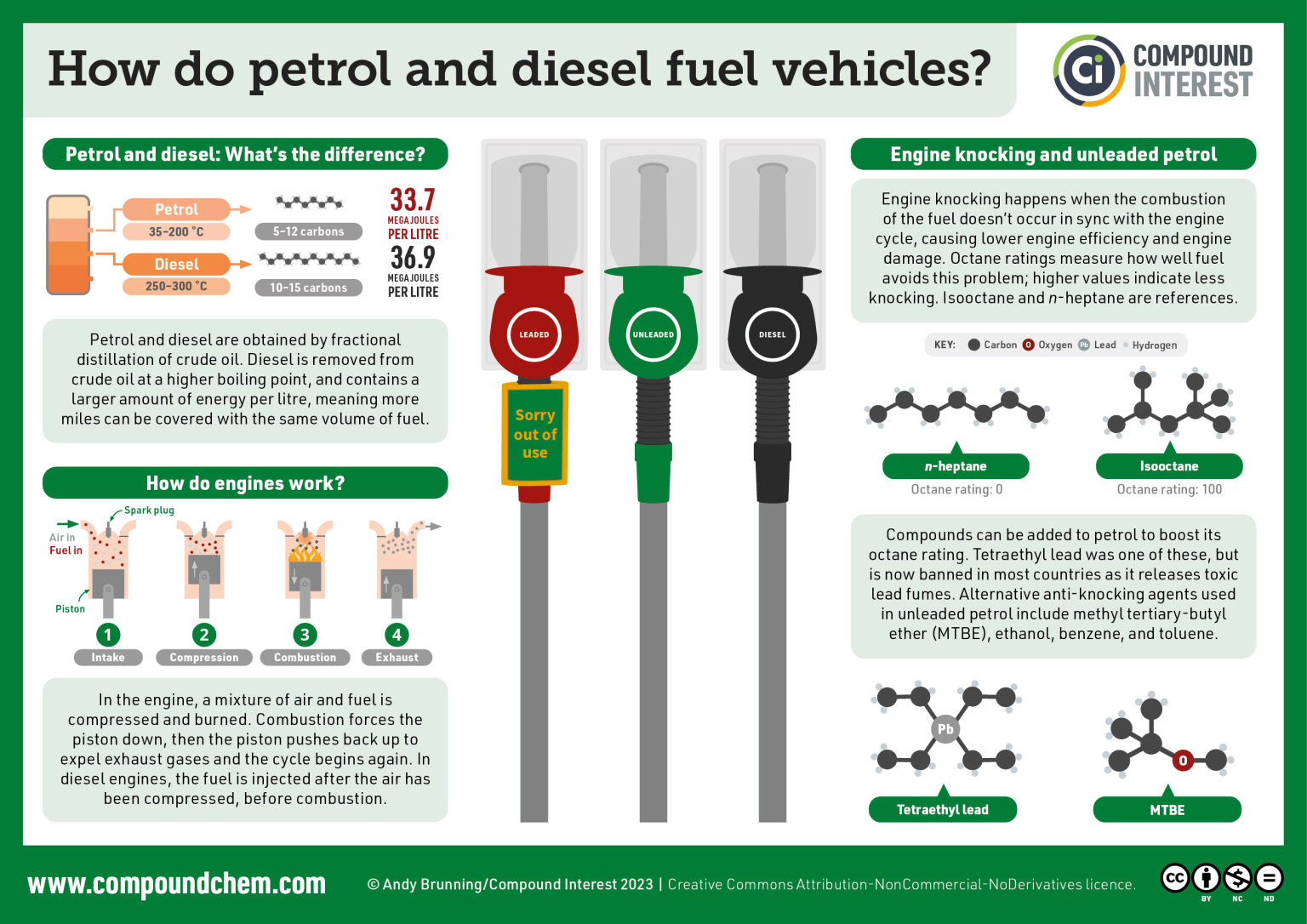
Substitution
In a substitution reaction (ex. halogenation), another typical reaction of alkanes, one or more of the alkane’s hydrogen atoms is replaced with a different atom or group of atoms. No carbon-carbon bonds are broken in these reactions, and the hybridization of the carbon atoms does not change. For example in Figure 20.6c., the reaction between ethane and molecular chlorine demonstrates a substitution reaction.
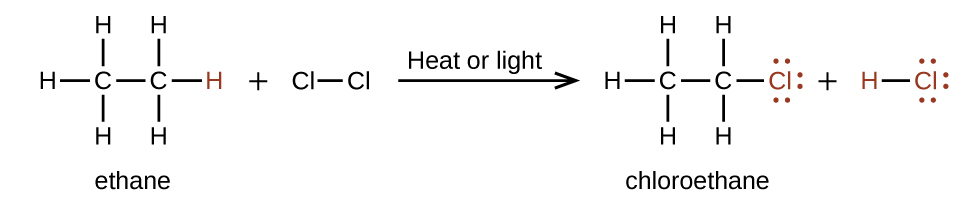
The C–Cl portion of the chloroethane molecule is an example of a functional group, the part or moiety of a molecule that imparts a specific chemical reactivity. The types of functional groups present in an organic molecule are major determinants of its chemical properties and are used as a means of classifying organic compounds as detailed in the remaining sections of this chapter.
A wide variety of interesting and often useful compounds have one or more halogen atoms per molecule. For example, methane (CH4) can react with chlorine (Cl2), replacing one, two, three, or all four hydrogen atoms with Cl atoms. With more chlorine, a mixture of products is obtained: CH3Cl, CH2Cl2, CHCl3, and CCl4. Fluorine (F), the lightest halogen, combines explosively with most hydrocarbons. Iodine (I) is relatively unreactive. Fluorinated and iodinated alkanes are produced by indirect methods.
Several halogenated products derived from methane and ethane (CH3CH3) are listed in Table 20.6a., along with some of their uses.
Formula | Common Name | IUPAC Name | Some Important Uses |
---|---|---|---|
Derived from CH4 | |||
CH3Cl | methyl chloride | chloromethane | refrigerant; the manufacture of silicones, methyl cellulose, and synthetic rubber |
CH2Cl2 | methylene chloride | dichloromethane | laboratory and industrial solvent |
CHCl3 | chloroform | trichloromethane | industrial solvent |
CCl4 | carbon tetrachloride | tetrachloromethane | dry-cleaning solvent and fire extinguishers (but no longer recommended for use) |
CBrF3 | halon-1301 | bromotrifluoromethane | fire extinguisher systems |
CCl3F | chlorofluorocarbon-11 (CFC-11) | trichlorofluoromethane | foaming plastics |
CCl2F2 | chlorofluorocarbon-12 (CFC-12) | dichlorodifluoromethane | refrigerant |
Derived from CH3CH3 | |||
CH3CH2Cl | ethyl chloride | chloroethane | local anesthetic |
ClCH2CH2Cl | ethylene dichloride | 1,2-dichloroethane | solvent for rubber |
CCl3CH3 | methylchloroform | 1,1,1-trichloroethane | solvent for cleaning computer chips and molds for shaping plastics |
Table source: Map: Fundamentals of GOB Chemistry (McMurry et al.), CC BY-NC-SA 3.0.
Spotlight on Everyday Chemistry: Chlorofluorocarbons (CFC’s), The Ozone Layer and Susan Solomon
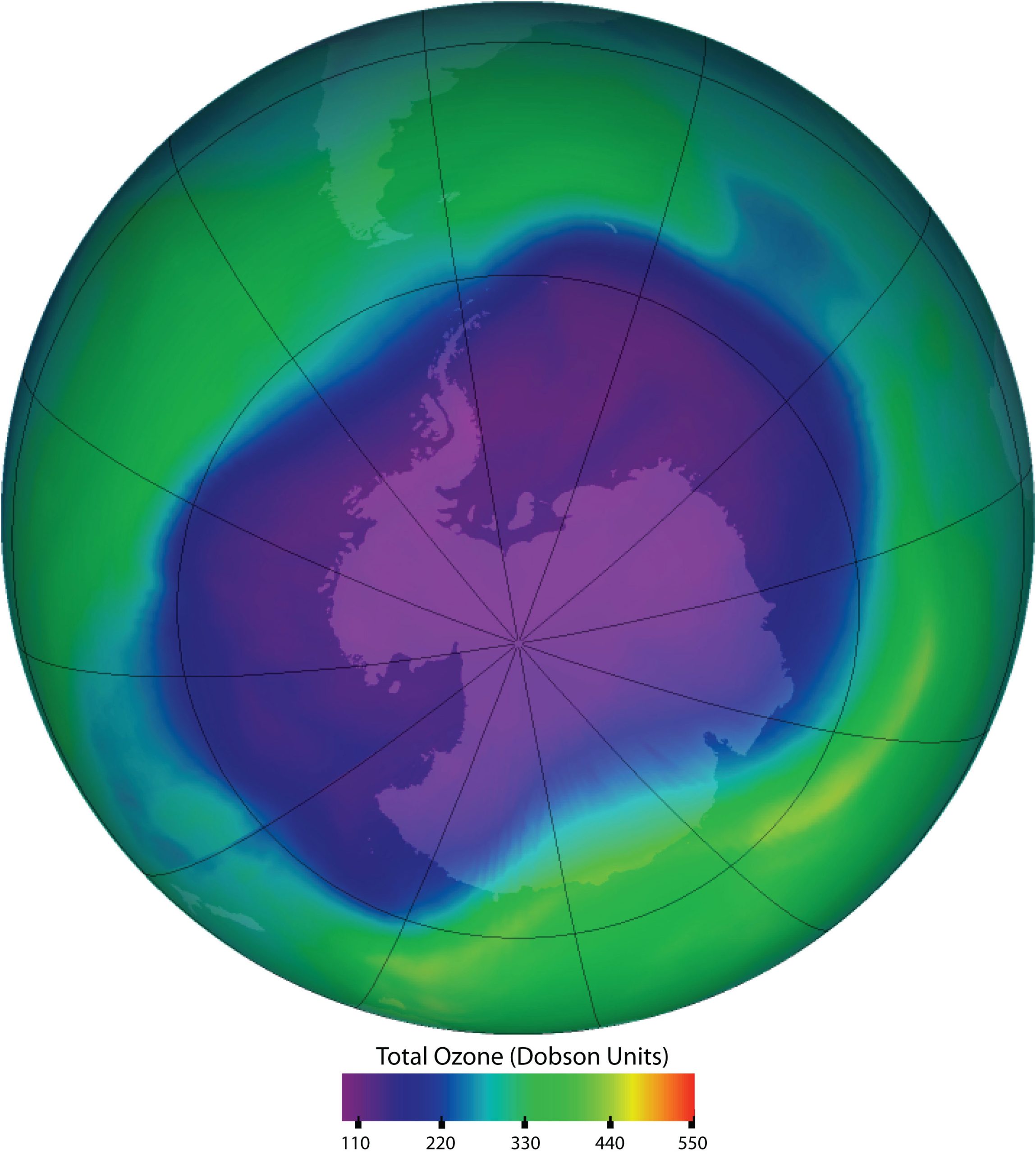
Chlorofluorocarbons and the Ozone Layer
Alkanes substituted with both fluorine (F) and chlorine (Cl) atoms have been used as the dispersing gases in aerosol cans, as foaming agents for plastics, and as refrigerants. Two of the best known of these chlorofluorocarbons (CFCs) are listed in Table 20.6a.
Chlorofluorocarbons contribute to the greenhouse effect in the lower atmosphere. They also diffuse into the stratosphere, where they are broken down by ultraviolet (UV) radiation to release Cl atoms. These in turn break down the ozone (O3) molecules that protect Earth from harmful UV radiation as shown in Figure 20.6d. Worldwide action has reduced the use of CFCs and related compounds. The CFCs and other Cl- or bromine (Br)-containing ozone-destroying compounds are being replaced with more benign substances. Hydrofluorocarbons (HFCs), such as CH2FCF3, which have no Cl or Br to form radicals, are one alternative. Another is hydrochlorofluorocarbons (HCFCs), such as CHCl2CF3. HCFC molecules break down more readily in the troposphere, and fewer ozone-destroying molecules reach the stratosphere.
Thanks to Susan Solomon as described in infographic 20.6b, she confirmed that ozone could react with CFC’s in the stratosphere breaking it down..
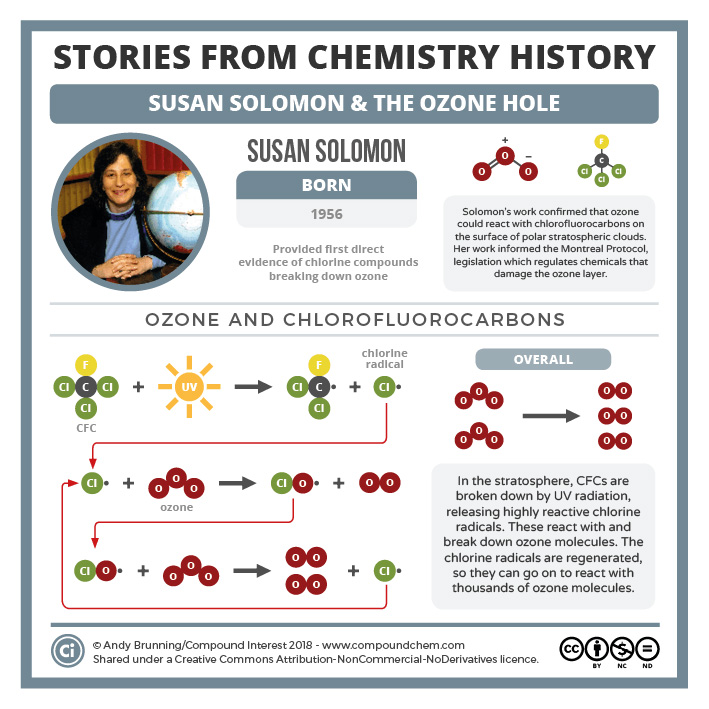
For more information on reactions of alkanes, watch Radical Reactions & Hammond’s Postulate below.
Watch Radical Reactions & Hammond’s Postulate: Crash Course Organic Chemistry #19 – YouTube (12 min)
Video Source: Crash Course. (2021, January 6). Radical Reactions & Hammond’s Postulate: Crash Course Organic Chemistry #19 [Video]. YouTube.
Cracking (Elimination) – Making Alkenes
Ethylene and propylene, the simplest alkenes, are the two most important organic chemicals produced industrially. Approximately 220 million tons of ethylene and 138 million tons of propylene are produced worldwide each year for use in the synthesis of polyethylene, polypropylene, ethylene glycol, acetic acid, acetaldehyde, and a host of other substances (Figure 20.6e.).
Ethylene, propylene, and butene are synthesized from (C2–C8) alkanes by a process called steam cracking at temperatures up to 900 °C. This process is shown in Figure 20.6f. below.
The cracking process is complex, although it undoubtedly involves radical reactions. The high-temperature reaction conditions cause spontaneous breaking of C−C and C−H bonds, with the resultant formation of smaller fragments. We might imagine, for instance, that a molecule of butane splits into two ethyl radicals, each of which then loses a hydrogen atom to generate two molecules of ethylene as demonstrated in Figure 20.6g.
Other Alkane Reactions
Two additional alkane reactions include dehydrogenation and isomerization. Dehydrogenation is an elimination reaction where a hydrogen is lost from an alkane to create an alkene under high temperatures. The results are a by-product of hydrogen gas and an alkene. This reaction is unpredictable as the location of the carbon-carbon double bond is random. The dehydrogenation process is used in the production of motor fuels and petrochemicals (Hein et al., 2013, p. 464). The dehydrogenation reaction of butane is shown in Figure 20.6h.
Isomerization occurs when there is a rearrangement of the molecular structure under heat, pressure and exposure to a catalyst. Again, this process is used in the production of motor fuels and petrochemicals (Hein et al., 2013, p. 464). For example, in Figure 20.6i., the isomerization of butane is demonstrated.
For more details on reactions involving alkanes refer to the map of some of the more common reactions to convert functional groups in Section 19.6 – General Reactions of Carbon in Infographic 19.6a.
Attribution & References
Except where otherwise noted, this page is adapted by Adrienne Richards from
- “12.8: Reactions of Alkanes” In Map: Fundamentals of General Organic and Biological Chemistry (McMurry et al.) by LibreTexts, licensed under CC BY-NC-SA 3.0 . / A derivative of
- “12.7: Chemical Properties of Alkanes“, “12.8: Halogenated Hydrocarbons” In Basics of GOB (Ball et al.), CC BY-NC-SA 4.0 a LibreTexts version of Introduction to Chemistry: GOB (v. 1.0), CC BY-NC 3.0
- “20.1: Hydrocarbons” In Chemistry 1e (OpenStax) a LibreText version of Chemistry / Chemistry 2e (OpenStax) by by Paul Flowers, Klaus Theopold, Richard Langley & William R. Robinson is licensed CC BY 4.0. Access for free at Chemistry 2e (Open Stax)
- Combustion section also includes content from “18.1 Hydrocarbons” In General Chemistry 1 & 2 by Rice University. / A derivative of Chemistry (Open Stax) by Paul Flowers, Klaus Theopold, Richard Langley & William R. Robinson and is licensed under CC BY 4.0. Access for free at Chemistry (OpenStax)
- “Cracking – Making Alkenes” is adapted from “7.1 Industrial Preparation and Use of Alkenes” In Organic Chemistry (OpenStax) by John McMurray, licensed under CC BY-NC-SA 4.0. Access for free at Organic Chemistry (OpenStax)
References cited in-text
Hein, M., Pattison, S., Arena, S., & Best, L. (2013). Introduction to general, organic, and biochemistry (11th ed.). John Wiley & Sons, Inc.
occurs when alkanes (hydrocarbons) burn in the presence of oxygen, a highly exothermic oxidation-reduction reaction that produces carbon dioxide and water. A spark or flame are needed to start the reaction.
occur when two reactants exchange parts to give two new products
The structural components differentiating different organic families involve specific arrangements of atoms or bonds. This is the part of a molecule that imparts a specific chemical reactivity.
elimination reaction where a hydrogen is lost from an alkane to create an alkene under high temperatures
rearrangement of the molecular structure under heat, pressure and exposure to a catalyst