29.8 Nuclear Magnetic Resonance (NMR)
Learning Objectives
By the end of this section, you will be able to:
- Describe how an NMR spectrum is formed.
- Identify the formation and purpose of a chemical shift.
Some types of atomic nuclei act as though they spin on their axis similar to the Earth. Since they are positively charged, they generate an electromagnetic field just as the Earth does. So, in effect, they will act as tiny bar magnetics. Not all nuclei act this way, but fortunately both 1H and 13C do have nuclear spins and will respond to this technique.
NMR Spectrometer
In the absence of an external magnetic field the direction of the spin of the nuclei will be randomly oriented (see Figure 29.8a.). However, when a sample of these nuclei is place in an external magnetic field, the nuclear spins will adopt specific orientations much as a compass needle response to the Earth’s magnetic field and aligns with it. Two possible orientations are possible, with the external field (i.e. parallel to and in the same direction as the external field) or against the field (i.e. antiparallel to the external field).
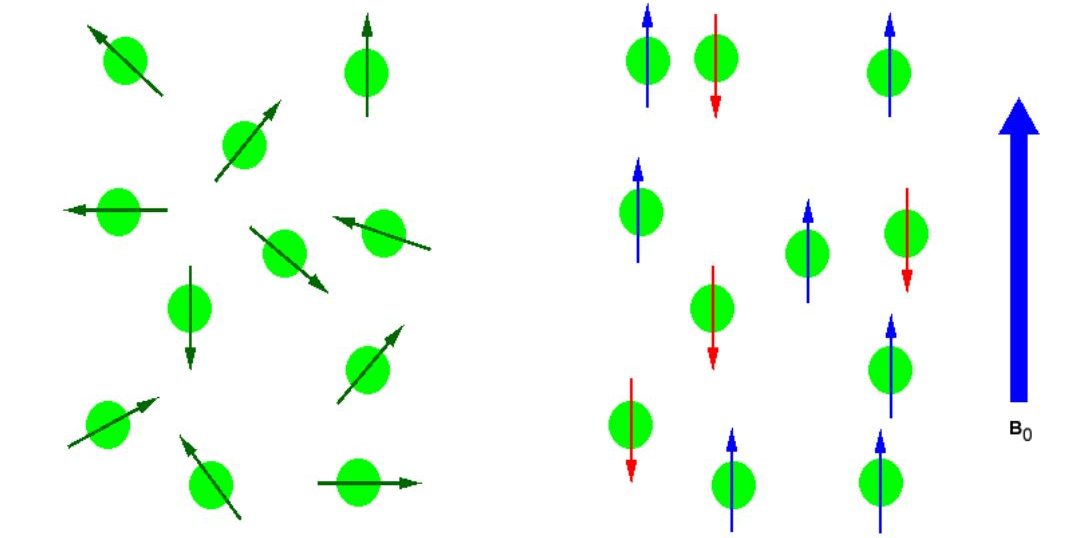
When the same sample is placed within the field of a very strong magnet in an NMR instrument, each hydrogen will assume one of two possible spin states (Figure 29.8b.). In what is referred to as the +½ spin state, the hydrogen’s magnetic moment is aligned with the direction of B0, while in the -½ spin state it is aligned opposed to the direction of B0.
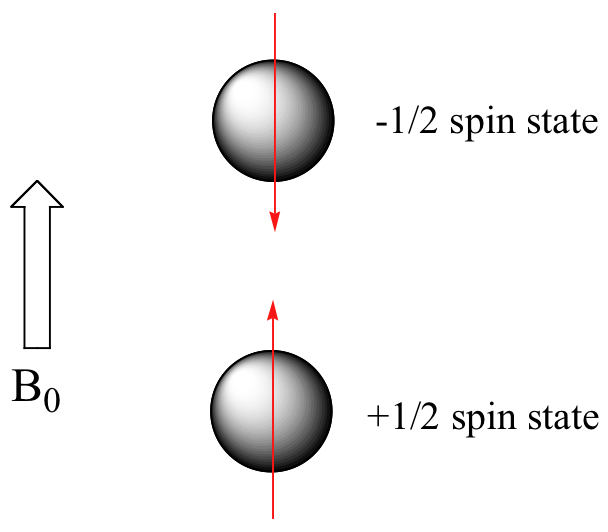
Because the +½ spin state is slightly lower in energy, in a large population of organic molecules slightly more than half of the hydrogen atoms will occupy this state, while slightly less than half will occupy the –½ state. The difference in energy between the two spin states increases with increasing strength of B0. With the strong magnetic fields generated by the superconducting magnets used in modern NMR instruments, the resonance frequency for atoms falls within the radio-wave range, anywhere from 100 MHz to 800 MHz depending on the strength of the magnet. The nuclei of atoms aligned with the field will absorb energy and “spin-flip” to align themselves against the field, a higher energy state. When this spin-flip occurs, the nuclei are said to be in “resonance” with the field, hence the name for the technique, Nuclear Magnetic Resonance or NMR.
1H and 13C are not unique in their ability to undergo NMR. All nuclei with an odd number of protons (1H, 2H, 14N, 19F, 31P …) or nuclei with an odd number of neutrons (i.e. 13C) show the magnetic properties required for NMR. Only nuclei with even number of both protons and neutrons (12C and 16O) do not have the required magnetic properties.
The basic arrangement of an NMR spectrometer is shown in Figure 29.8c. A sample (in a small glass tube) is placed between the poles of a strong magnetic. A radio frequency generator pulses the sample and excites the nuclei causing a spin-flip. The spin flip is detected by the detector and the signal sent to a computer where it is processed.
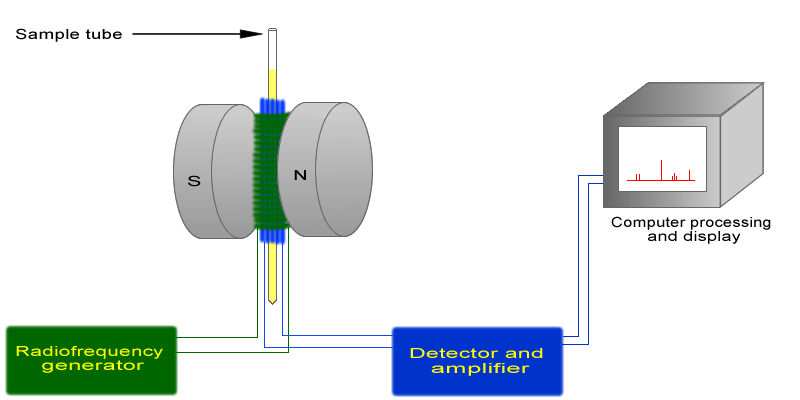
Chemical Shifts
The NMR spectra is displayed as a plot of the applied radio frequency versus the absorption. The applied frequency increases from left to right, thus the left side of the plot is the low field, downfield or deshielded side and the right side of the plot is the high field, upfield or shielded side (see Figure 29.8e.).
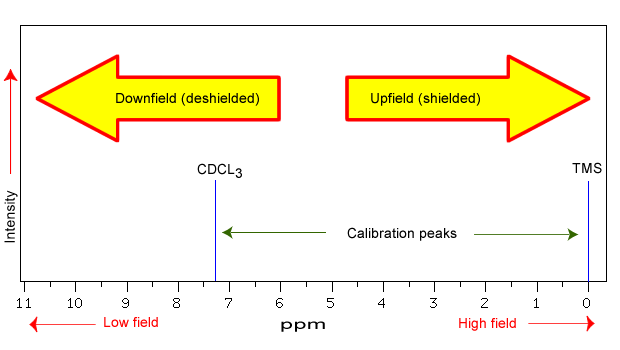
The position on the plot at which the nuclei absorbs is called the chemical shift. Since this has an arbitrary value a standard reference point must be used. The two most common standards are TMS (tetramethylsilane, (Si(CH3)4) which has been assigned a chemical shift of zero, and CDCl3 (deuterochloroform) which has a chemical shift of 7.26 for 1H NMR and 77 for 13C NMR. The delta (δ) scale is commonly expressed as parts per million (ppm) which is independent of the spectrometer frequency.
The range at which most NMR absorptions occur is quite narrow. Almost all 1H absorptions occur downfield within 10 ppm of TMS. For 13C NMR almost all absorptions occurs within 220 ppm downfield of the C atom in TMS.
Structural features of the molecule will have an effect on the exact magnitude of the magnetic field experienced by a particular nucleus. This means that H atoms which have different chemical environments will have different chemical shifts. This is what makes NMR so useful for structure determination in organic chemistry. There are three main features that will affect the shielding of the nucleus, electronegativity, magnetic anisotropy of π systems and hydrogen bonding.
The electrons that surround the nucleus are in motion so they created their own electromagnetic field. This field opposes the applied magnetic field and so reduces the field experienced by the nucleus. Thus, the electrons are said to shield the nucleus. Since the magnetic field experienced at the nucleus defines the energy difference between spin states it also defines what the chemical shift will be for that nucleus. Electron with-drawing groups can decrease the electron density at the nucleus, deshielding the nucleus and result in a larger chemical shift. These inductive effects are not only felt by the immediately adjacent atoms, but the deshielding can occur further down the chain.
The π electrons in a compound, when placed in a magnetic field, will move and generate their own magnetic field. The new magnetic field will have an effect on the shielding of atoms within the field. The best example of this is benzene (see Figure 29.8e.). This effect is common for any atoms near a π bond.
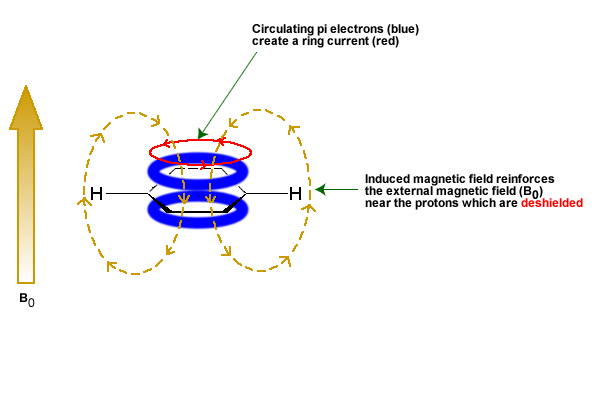
Protons that are involved in hydrogen bonding (i.e.-OH or -NH) are usually observed over a wide range of chemical shifts. This is due to the deshielding that occurs in the hydrogen bond. Since hydrogen bonds are dynamic, constantly forming, breaking and forming again, there will be a wide range of hydrogen bonds strengths and consequently a wide range of deshielding. This as well as solvation effects, acidity, concentration and temperature make it very difficult to predict the chemical shifts for these atoms.
Attribution & References
Except where otherwise noted, this page is adapted by Samantha Sullivan Sauer from “12.1: Theory of Nuclear Magnetic Resonance (NMR)” and “12.3: Chemical Shifts and Shielding” In Map: Organic Chemistry (Wade), Complete and Semesters I and II by LibreTexts, licensed under CC BY-NC-SA 4.0. Contributors from original source:
- Dr. Dietmar Kennepohl FCIC (Professor of Chemistry, Athabasca University)
- Prof. Steven Farmer (Sonoma State University)
- Dr. Richard Spinney (The Ohio State University)
The position on the NMR plot at which the nuclei absorbs