19.6 General Reactions of Carbon
Learning Objectives
By the end of this section, you will be able to:
- Describe the reactions characteristic of saturated and unsaturated hydrocarbons
Common Chemical Reactions in Organic Chemistry
There are multiple types of organic chemical reactions. Some of the general organic reactions are additions, eliminations, substitutions, rearrangements and oxidation-reduction.
Addition reactions
Addition reactions occur when two reactants add together to form a single product with no atoms “left over.” An example that we’ll be studying soon is the reaction of an alkene, such as ethylene, with HBr to yield an alkyl bromide as shown in Figure 19.6a.
Chemically, the alkynes are similar to the alkenes. Since the [latex]\text{C}\;{\equiv}\;\text{C}[/latex] functional group has two π bonds, alkynes typically react even more readily, and react with twice as much reagent in addition reactions. The reaction of acetylene with bromine is a typical example that is demonstrated in Figure 19.6b.

Elimination reactions
- Elimination reactions are, in a sense, the opposite of addition reactions. They occur when a single reactant splits into two products, often with the formation of a small molecule such as water or HBr. An example is the acid-catalyzed reaction of an alcohol to yield water and an alkene as shown in Figure 19.6c.
Figure 19.6c. Elimination reaction of ethanol to yield ethylene and water. (credit: Organic Chemistry (OpenStax), CC BY NC SA 4.0).
Substitution reactions
Substitution reactions occur when two reactants exchange parts to give two new products. An example is the reaction of an ester such as methyl acetate with water to yield a carboxylic acid plus an alcohol as shown in Figure 19.6d. Similar reactions occur in many biological pathways, including the metabolism of dietary fats.
Another typical substitution reaction is one that involves alkanes, where one or more of the alkane’s hydrogen atoms is replaced with a different atom or group of atoms. No carbon-carbon bonds are broken in these reactions, and the hybridization of the carbon atoms does not change. For example, the reaction between ethane and molecular chlorine depicted in Figure 19.6e. is a substitution reaction:
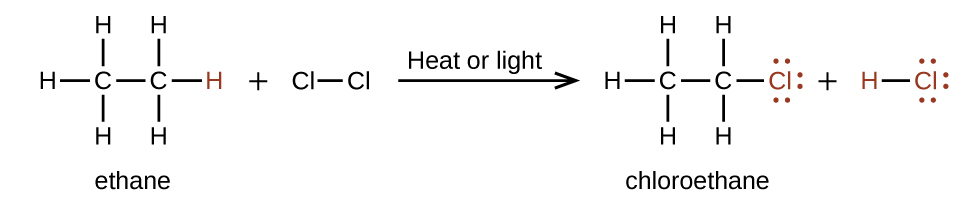
Rearrangement reactions
Rearrangement reactions occur when a single reactant undergoes a reorganization of bonds and atoms to yield an isomeric product. An example as demonstrated in Figure 19.6f. is the conversion of dihydroxyacetone phosphate into its constitutional isomer glyceraldehyde 3-phosphate, a step in the glycolysis pathway by which carbohydrates are metabolized.
Oxidation-reduction reactions
Oxidation–reduction reactions, which are common in organic chemistry, can often be identified by changes in the number of oxygen atoms at a particular position in the hydrocarbon skeleton or in the number of bonds between carbon and oxygen at that position (Figure 19.6g.). An increase in either corresponds to an oxidation, whereas a decrease corresponds to a reduction (Figure 19.6h.). Conversely, an increase in the number of hydrogen atoms in a hydrocarbon is often an indication of a reduction. In Figure 19.6g, ethane is oxidized to carbon dioxide. The number of oxygen atoms and this the number of bonds between carbon and oxygen increase through the reaction so it is an oxidation of carbon reaction. In Figure 19.6h., carbon dioxide is reduced to ethane. The number of carbon-oxygen bonds is decreased; more carbon-hydrogen bonds are formed so it is a reduction of carbon reaction.


We can illustrate these points by considering how the oxidation state of the carbon atom changes in the series of compounds, which is shown in part (a) in Figure 19.6i. The number of oxygen atoms or the number of bonds to oxygen changes throughout the series. Hence the conversion of methane to formic acid is an oxidation, whereas the conversion of carbon dioxide to methanol is a reduction. Also, the number of hydrogen atoms increases in going from the most oxidized to least oxidized compound. As expected, as the oxidation state of carbon increases, the carbon becomes a more potent electrophile. Thus the carbon of CO2 is a stronger electrophile (i.e., more susceptible to nucleophilic attack) than the carbon of an alkane such as methane.
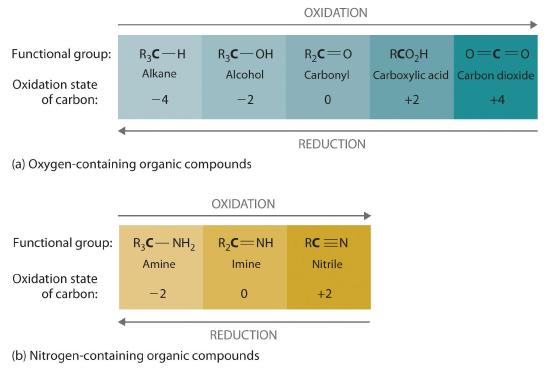
Similarly, in compounds with a carbon–nitrogen bond, the number of bonds between the C and N atoms increases as the oxidation state of the carbon increases (part (b) in Figure 19.6i. In a nitrile, which contains the –C≡N group, the carbon has the same oxidation state (+2) as in a carboxylic acid, characterized by the –CO2H group. We therefore expect the carbon of a nitrile to be a rather strong electrophile.
An example of an oxidation reaction is a combustion reaction involving hydrocarbons such as alkanes. Alkanes are relatively stable molecules, but heat or light will activate reactions that involve the breaking of C–H or C–C single bonds. Combustion is one such reaction:
Alkanes burn in the presence of oxygen, a highly exothermic oxidation-reduction reaction that produces carbon dioxide and water. As a consequence, alkanes are excellent fuels. For example, methane, CH4, is the principal component of natural gas. Butane, C4H10, used in camping stoves and lighters is an alkane. Gasoline is a liquid mixture of continuous- and branched-chain alkanes, each containing from five to nine carbon atoms, plus various additives to improve its performance as a fuel. Kerosene, diesel oil, and fuel oil are primarily mixtures of alkanes with higher molecular masses. The main source of these liquid alkane fuels is crude oil, a complex mixture that is separated by fractional distillation. Fractional distillation takes advantage of differences in the boiling points of the components of the mixture. You may recall that boiling point is a function of intermolecular interactions. Acetylene and the other alkynes also burn readily. An acetylene torch takes advantage of the high heat of combustion for acetylene.
Smudging is an example of a combustion reaction. Learn more about this by looking at the Indigenous Perspective below.
Indigenous Perspectives: Smudging
Combustion reactions occur within indigenous smudging ceremonies through the burning of natural plants such as sage, sweetgrass, cedar and tobacco.
Watch Smudging Ceremony Explained by Stephen Augustine on YouTube (5 mins)
For more information on smudging refer to Smudging by The Canadian Encyclopedia.
Video Source: Cape Breton University. (2020, September 9). Smudging Ceremony Explained by Stephen Augustine [Video]. YouTube.
Example 19.6a
Write an equation to describe each reaction.
- the substitution reaction of potassium cyanide with 1-chloropropane to give CH3CH2CH2CN (butyronitrile)
- the addition reaction of HBr with cis-2-butene
Solution
Given: reactants, products, and reaction mechanism
Asked for: equation
Strategy: Use the mechanisms described to show how the indicated products are formed from the reactants.
- The CN− ion of KCN can displace the chlorine atom of 1-chloropropane, releasing a chloride ion. Substitution results in the formation of a new C–C bond:
\( CN^{-}+\underset{1-chloropropane}{CH_{3}CH_{2}CH_{2}Cl}\rightarrow \underset{butylnitrile}{CH_{3}CH_{2}CH_{2}CN} + Cl^{-} \) - In the addition of a hydrogen halide to an alkene, the reaction is shown in Figure 19.6j.
Figure 19.6j. The electrophilic addition of a HBr to 2-butene. (credit: General Chem: Principles, Patterns, and Applications (Averill), CC BY-NC-SA 3.0). Source: Example 19.6a is adapted by Adrienne Richards from General Chemistry: Principles, Patterns, and Applications (Averill), CC BY-NC-SA 3.0
Exercise 19.6a
Write an equation to describe each reaction.
- the substitution reaction of sodium methoxide (NaOCH3) with benzyl bromide (C6H5CH2Br)
- the acid-catalyzed addition reaction of water with cyclopentene
Check Your Answer[1]
Source: Exercise 19.6a is adapted by Adrienne Richards from Map: General Chemistry: Principles, Patterns, and Applications (Averill), CC BY-NC-SA 3.0
The reactions described above only cover the common reactions seen in organic chemistry, however, there are plenty more! Infographic 19.6a. provides an overview of the various types of organic chemistry reactions that can occur.
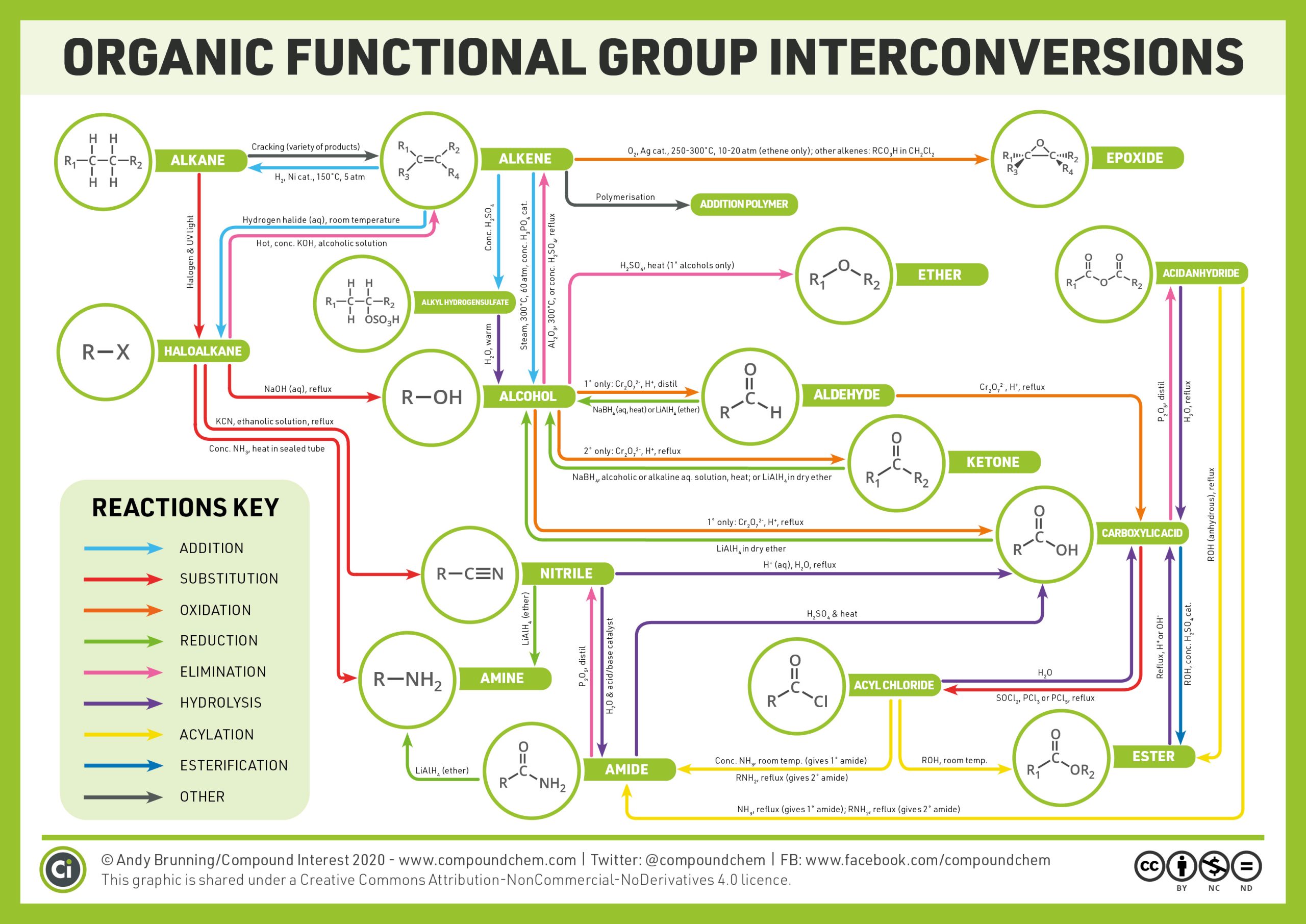
Reaction Mechanisms
Having looked at the kinds of reactions that take place, let’s now see how they occur. An overall description of how a reaction occurs is called a reaction mechanism. A mechanism describes in detail exactly what takes place at each stage of a chemical transformation—which bonds are broken and in what order, which bonds are formed and in what order, and what the relative rates are for each step. A complete mechanism must also account for all reactants used and all products formed.
Arrows in organic chemistry reactions are important to understand as they differ depending on the type of reaction taking place. Infographic 19.6b. summarizes the various arrows used in organic chemistry reactions.
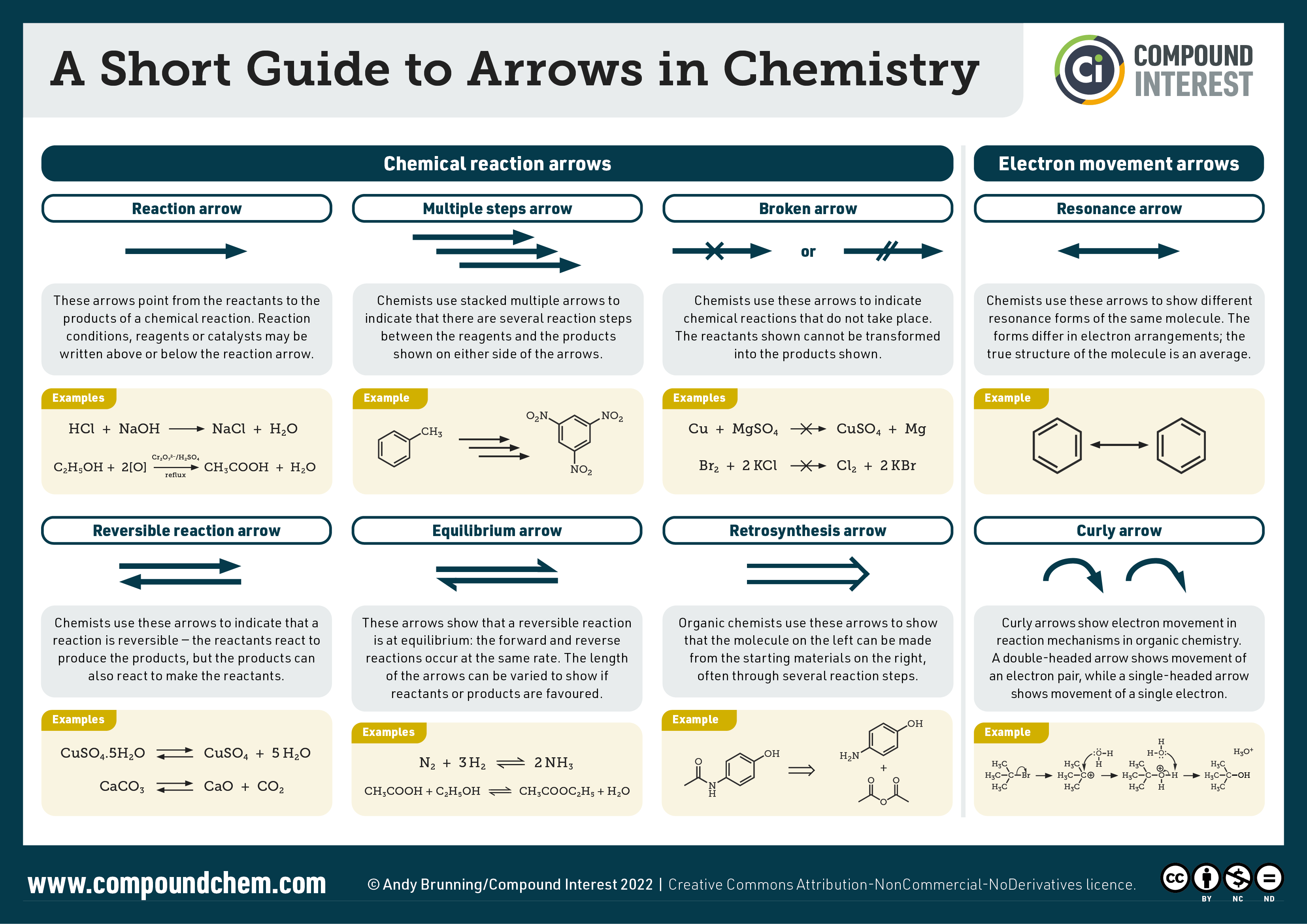
All chemical reactions involve bond-breaking and bond-making. When two molecules come together, react, and yield products, specific bonds in the reactant molecules are broken and specific bonds in the product molecules are formed. Fundamentally, there are two ways in which a covalent two-electron bond can break. A bond can break in an electronically unsymmetrical way so that both bonding electrons remain with one product fragment, leaving the other with a vacant orbital, or a bond can break in an electronically symmetrical way so that one electron remains with each product fragment. The unsymmetrical cleavage is said to be heterolytic, and the symmetrical cleavage is said to be homolytic.
Generally, the movement of two electrons in the unsymmetrical process is indicated using a full-headed curved arrow (), whereas the movement of one electron in the symmetrical process is indicated using a half-headed, or “fishhook,” arrow (
) as demonstrated in Figure 19.6k.
Just as there are two ways in which a bond can break, there are two ways in which a covalent two-electron bond can form. A bond can form in an electronically unsymmetrical way if both bonding electrons are donated to the new bond by one reactant, or in a symmetrical way if one electron is donated by each reactant as demonstrated in Figure 19.6l.
Processes that involve unsymmetrical bond-breaking and bond-making are called polar reactions. Polar reactions involve species that have an even number of electrons and thus have only electron pairs in their orbitals. Polar processes are by far the more common reaction type in both organic and biological chemistry, and a large part of this book is devoted to their description.
Processes that involve symmetrical bond-breaking and bond-making are called radical reactions. A radical, often called a free radical, is a neutral chemical species that contains an odd number of electrons and thus has a single, unpaired electron in one of its orbitals.
In addition to polar and radical reactions, there is a third, less commonly encountered process called a pericyclic reaction.
For a visual explanation of reaction mechanism refer to the video below called Intro to Reaction Mechanisms.
Watch Intro to Reaction Mechanisms: Crash Course Organic Chemistry #13 – YouTube (13 min)
Video Source: Crash Course. (2020, September 30). Intro to Reaction Mechanisms: Crash Course Organic Chemistry #13 (youtube.com)[Video]. YouTube.
Attribution & References
Except where otherwise noted, this page is adapted by Adrienne Richards from
- “18.1 Hydrocarbons” In General Chemistry 1 & 2 by Rice University, a derivative of Chemistry (Open Stax) by Paul Flowers, Klaus Theopold, Richard Langley & William R. Robinson and is licensed under CC BY 4.0. Access for free at Chemistry (OpenStax)
- “6.1 Kinds of Organic Reactions” and “6.2 How Organic Reactions Occur: Mechanisms” In Organic Chemistry (OpenStax), CC BY-NC-SA 4.0. Access for free at Organic Chemistry (OpenStax)
- Oxidation- Reduction Reaction section is adapted from “23.5: Common Classes of Organic Reactions” In Map: General Chemistry: Principles, Patterns, and Applications (Averill) was authored, remixed, and/or curated by Joshua B. Halpern / LibreTexts / Anonymous, CC BY-NC-SA 3.0
- “23.5: Common Classes of Organic Reactions” In Map: General Chemistry: Principles, Patterns, and Applications (Averill), CC BY-NC-SA 3.0
References cited in-text
Chen, C. S., Wan, J. H., & Yeo, B. S. (2015). Electrochemical reduction of Carbon Dioxide to Ethane using nanostructured Cu2O-Derived Copper Catalyst and Palladium(II) Chloride. The Journal of Physical Chemistry, 119(48), 26875-26882. DOI: 10.1021/acs.jpcc.5b09144
-
1. OCH3− (methoxide ion) + C6H5CH2Br (benzyl bromide) → C6H5CH2OCH3 (benzylmethyl ether) + Br
2. The addition reaction is shown below. Water and acid become the hydronium ion.
↵
reactions in which a double carbon-carbon bond forms a single carbon-carbon bond by the addition of a reactant. Typical reaction for an alkene.
The opposite of addition reactions. They occur when a single reactant splits into two products, often with the formation of a small molecule such as water or HBr
occur when two reactants exchange parts to give two new products
occur when a single reactant undergoes a reorganization of bonds and atoms to yield an isomeric product
identified by changes in the number of oxygen atoms at a particular position in the hydrocarbon skeleton or in the number of bonds between carbon and oxygen at that position. An increase in either corresponds to an oxidation, whereas a decrease corresponds to a reduction. Conversely, an increase in the number of hydrogen atoms in a hydrocarbon is often an indication of a reduction.
occurs when alkanes (hydrocarbons) burn in the presence of oxygen, a highly exothermic oxidation-reduction reaction that produces carbon dioxide and water. A spark or flame are needed to start the reaction.
a neutral chemical species that contains an odd number of electrons and thus has a single, unpaired electron in one of its orbitals