28.2 Lipids
Learning Objectives
By the end of this section, you will be able to:
- Describe the classification of lipids
- Recognize the structures of common fatty acids and classify them as saturated, monounsaturated, or polyunsaturated
- Explain why fats and oils are referred to as triglycerides
- Explain how the fatty acid composition of the triglycerides determines whether a substance is a fat or oil
- Describe the importance of key reactions of triglycerides, such as hydrolysis, hydrogenation, and oxidation
- Identify the distinguishing characteristics of membrane lipids
- Describe membrane components and how they are arranged
- Identify the functions of steroids produced in mammals
Nature’s Storehouse of Energy and the Backbone of the Cell
Lipids, a diverse group of organic molecules that includes fats, oils, phospholipids, and steroids, have played a pivotal role in the history and importance of biochemistry. Their significance lies in their multifaceted functions, such as energy storage, cellular membrane structure, and as crucial components of hormones and signaling molecules. The study of lipids has deep roots in biochemistry, dating back to the early 19th century when scientists began unraveling their structure and metabolic roles. Since then, research in lipid biochemistry has greatly expanded, shedding light on their role in health, disease, and cellular regulation, making them a cornerstone of our understanding of biological systems. The exploration of lipids has paved the way for breakthroughs in areas like nutrition, metabolism, and the development of life-saving drugs, underscoring their enduring importance in the field of biochemistry.
Spotlight on Everyday Chemistry: Diet and Metabolism
On July 11, 2003, the Food and Drug Administration amended its food labeling regulations to require that manufacturers list the amount of trans fatty acids on Nutrition Facts labels of foods and dietary supplements, effective January 1, 2006. This amendment was a response to published studies demonstrating a link between the consumption of trans fatty acids and an increased risk of heart disease. Trans fatty acids are produced in the conversion of liquid oils to solid fats, as in the creation of many commercial margarines and shortenings. They have been shown to increase the levels of low-density lipoproteins (LDLs)—complexes that are often referred to as bad cholesterol—in the blood. In this chapter, you will learn about fatty acids and what is meant by a trans fatty acid, as well as the difference between fats and oils. You will also learn what cholesterol is and why it is an important molecule in the human body.
Fats and oils, found in many of the foods we eat, belong to a class of biomolecules known as lipids. Gram for gram, they pack more than twice the caloric content of carbohydrates: the oxidation of fats and oils supplies about 9 kcal of energy for every gram oxidized, whereas the oxidation of carbohydrates supplies only 4 kcal/g. Although the high caloric content of fats may be bad news for the dieter, it says something about the efficiency of nature’s designs. Our bodies use carbohydrates, primarily in the form of glucose, for our immediate energy needs. Our capacity for storing carbohydrates for later use is limited to tucking away a bit of glycogen in the liver or in muscle tissue. We store our reserve energy in lipid form, which requires far less space than the same amount of energy stored in carbohydrate form. Lipids have other biological functions besides energy storage. They are a major component of the membranes of the 10 trillion cells in our bodies. They serve as protective padding and insulation for vital organs. Furthermore, without lipids in our diets, we would be deficient in the fat-soluble vitamins A, D, E, and K.
Classification of Lipids
Lipids are not defined by the presence of specific functional groups, as carbohydrates are, but by a physical property—solubility. Compounds isolated from body tissues are classified as lipids if they are more soluble in organic solvents, such as dichloromethane, than in water. By this criterion, the lipid category includes not only fats and oils, which are esters of the trihydroxy alcohol glycerol and fatty acids, but also compounds that incorporate functional groups derived from phosphoric acid, carbohydrates, or amino alcohols, as well as steroid compounds such as cholesterol (Figure 28.2a. presents one scheme for classifying the various kinds of lipids). We will discuss the various kinds of lipids by considering one subclass at a time and pointing out structural similarities and differences as we go.
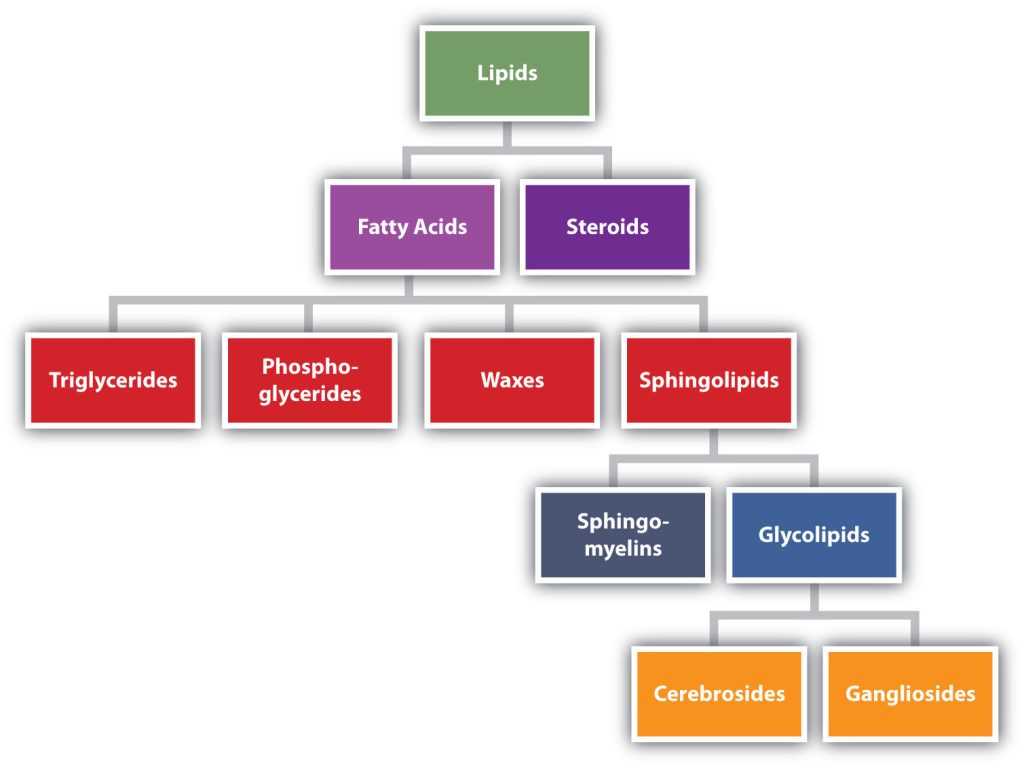
Fatty Acids
Fatty acids are carboxylic acids that are structural components of fats, oils, and all other categories of lipids, except steroids. More than 70 have been identified in nature. They usually contain an even number of carbon atoms (typically 12–20), are generally unbranched, and can be classified by the presence and number of carbon-to-carbon double bonds. Thus, saturated fatty acids contain no carbon-to-carbon double bonds, monounsaturated fatty acids contain one carbon-to-carbon double bond, and polyunsaturated fatty acids contain two or more carbon-to-carbon double bonds. The presence of carbon-to-carbon double bonds allows for both cis and trans isomers of fatty acids to exist, as shown in figure 28.2b.
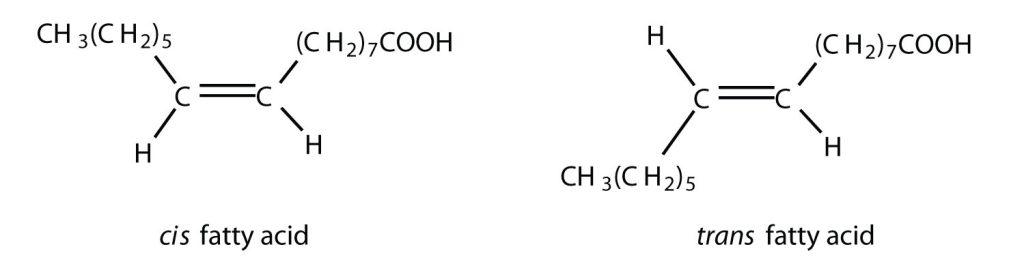
Table 28.2a. lists some common fatty acids and one important source for each. The atoms or groups around the double bonds in unsaturated fatty acids can be arranged in either the cis or trans isomeric form. Naturally occurring fatty acids are generally in the cis configuration.
Name | Abbreviated Structural Formula | Condensed Structural Formula | Melting Point (°C) | Source |
---|---|---|---|---|
lauric acid | C11H23COOH | CH3(CH2)10COOH | 44 | palm kernel oil |
myristic acid | C13H27COOH | CH3(CH2)12COOH | 58 | oil of nutmeg |
palmitic acid | C15H31COOH | CH3(CH2)14COOH | 63 | palm oil |
palmitoleic acid | C15H29COOH | CH3(CH2)5CH=CH(CH2)7COOH | 0.5 | macadamia oil |
stearic acid | C17H35COOH | CH3(CH2)16COOH | 70 | cocoa butter |
oleic acid | C17H33COOH | CH3(CH2)7CH=CH(CH2)7COOH | 16 | olive oil |
linoleic acid | C17H31COOH | CH3(CH2)3(CH2CH=CH)2(CH2)7COOH | −5 | canola oil |
α-linolenic acid | C17H29COOH | CH3(CH2CH=CH)3(CH2)7COOH | −11 | flaxseed |
arachidonic acid | C19H31COOH | CH3(CH2)4(CH2CH=CH)4(CH2)2COOH | −50 | liver |
Link to Enhanced Learning
Two polyunsaturated fatty acids—linoleic and α-linolenic acids—are termed essential fatty acids because humans must obtain them from their diets. Both substances are required for normal growth and development, but the human body does not synthesize them. The body uses linoleic acid to synthesize many of the other unsaturated fatty acids, such as arachidonic acid, a precursor for the synthesis of prostaglandins. In addition, the essential fatty acids are necessary for the efficient transport and metabolism of cholesterol. The average daily diet should contain about 4–6 g of the essential fatty acids.
Prostaglandins are chemical messengers synthesized in the cells in which their physiological activity is expressed. They are unsaturated fatty acids containing 20 carbon atoms and are synthesized from arachidonic acid—a polyunsaturated fatty acid—when needed by a particular cell. They are called prostaglandins because they were originally isolated from semen found in the prostate gland. It is now known that they are synthesized in nearly all mammalian tissues and affect almost all organs in the body. The five major classes of prostaglandins are designated as PGA, PGB, PGE, PGF, and PGI. Subscripts are attached at the end of these abbreviations to denote the number of double bonds outside the five-carbon ring in a given prostaglandin.
The prostaglandins are among the most potent biological substances known. Slight structural differences give them highly distinct biological effects; however, all prostaglandins exhibit some ability to induce smooth muscle contraction, lower blood pressure, and contribute to the inflammatory response. Aspirin and other nonsteroidal anti-inflammatory agents, such as ibuprofen, obstruct the synthesis of prostaglandins by inhibiting cyclooxygenase, the enzyme needed for the initial step in the conversion of arachidonic acid to prostaglandins.
Their wide range of physiological activity has led to the synthesis of hundreds of prostaglandins and their analogs. Derivatives of PGE2 are now used in the United States to induce labor (Figure 28.2c.). Other prostaglandins have been employed clinically to lower or increase blood pressure, inhibit stomach secretions, relieve nasal congestion, relieve asthma, and prevent the formation of blood clots, which are associated with heart attacks and strokes.
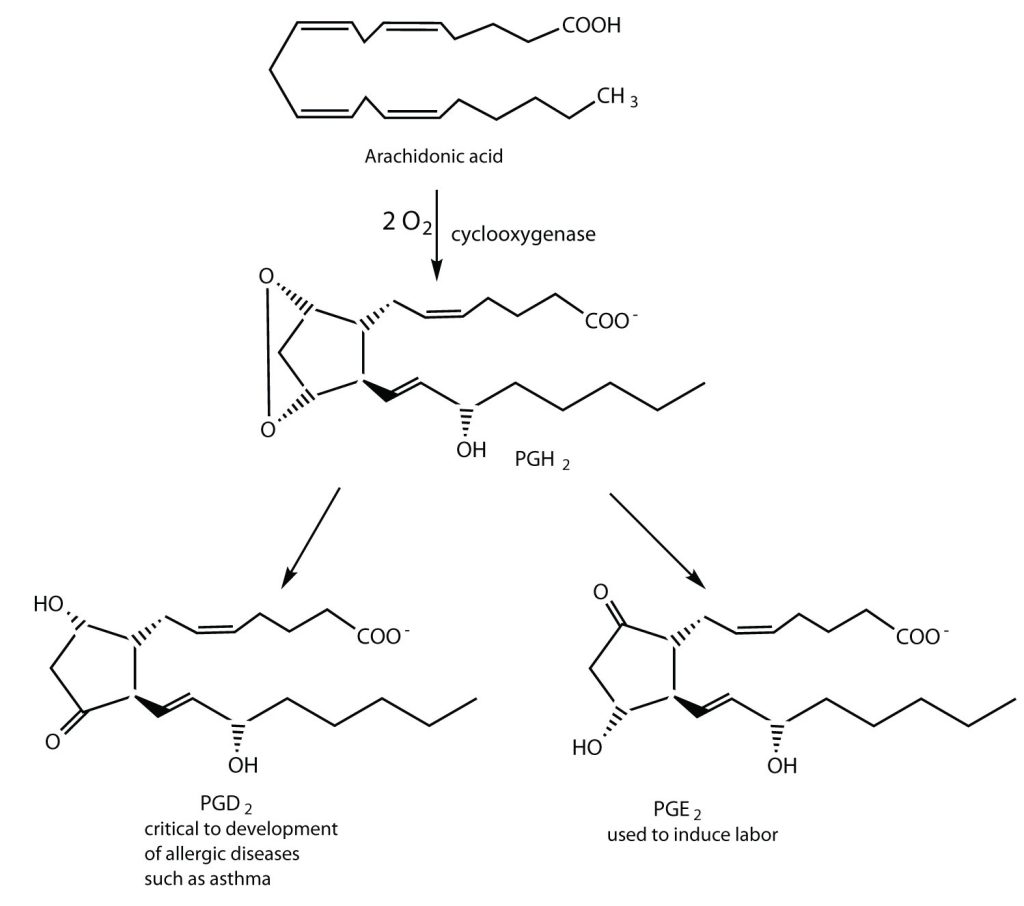
Although we often draw the carbon atoms in a straight line, they actually have more of a zigzag configuration (part a of Figure 28.2d.). Viewed as a whole, however, the saturated fatty acid molecule is relatively straight (part b of Figure 28.2d.). Such molecules pack closely together into a crystal lattice, maximizing the strength of dispersion forces and causing fatty acids and the fats derived from them to have relatively high melting points. In contrast, each cis carbon-to-carbon double bond in an unsaturated fatty acid produces a pronounced bend in the molecule, so that these molecules do not stack neatly. As a result, the intermolecular attractions of unsaturated fatty acids (and unsaturated fats) are weaker, causing these substances to have lower melting points. Most are liquids at room temperature.
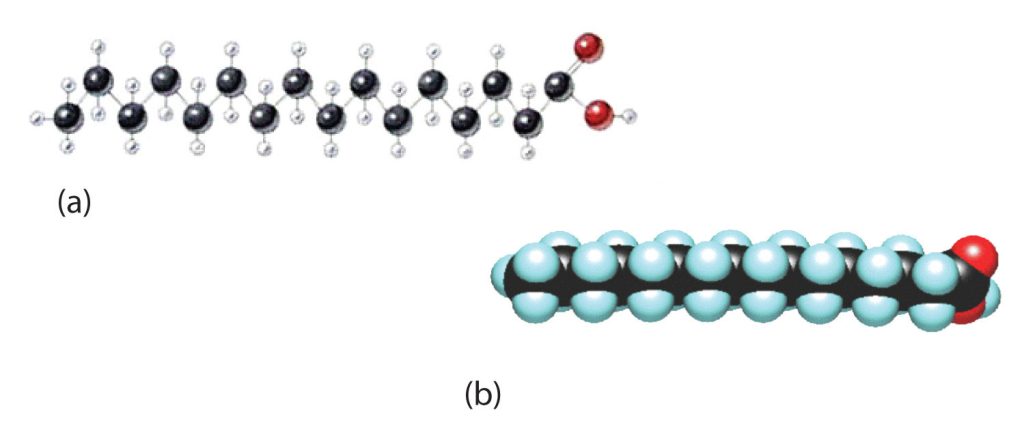
Waxes
Waxes are esters formed from long-chain fatty acids and long-chain alcohols. Most natural waxes are mixtures of such esters. Plant waxes on the surfaces of leaves, stems, flowers, and fruits protect the plant from dehydration and invasion by harmful microorganisms. Carnauba wax, used extensively in floor waxes, automobile waxes, and furniture polish, is largely myricyl cerotate, obtained from the leaves of certain Brazilian palm trees (Figure 28.2e.). Animals also produce waxes that serve as protective coatings, keeping the surfaces of feathers, skin, and hair pliable and water repellent. In fact, if the waxy coating on the feathers of a water bird is dissolved as a result of the bird swimming in an oil slick, the feathers become wet and heavy, and the bird, unable to maintain its buoyancy, drowns.
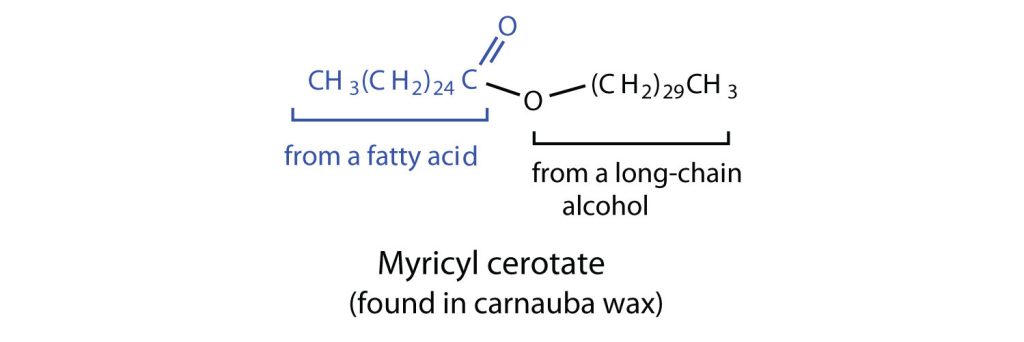
Fats and Oils
Fats and oils are the most abundant lipids in nature. They provide energy for living organisms, insulate body organs, and transport fat-soluble vitamins through the blood. Fats and oils are called triglycerides (or triacylcylgerols) because they are esters composed of three fatty acid units joined to glycerol, a trihydroxy alcohol, as seen in Figure 28.2f.
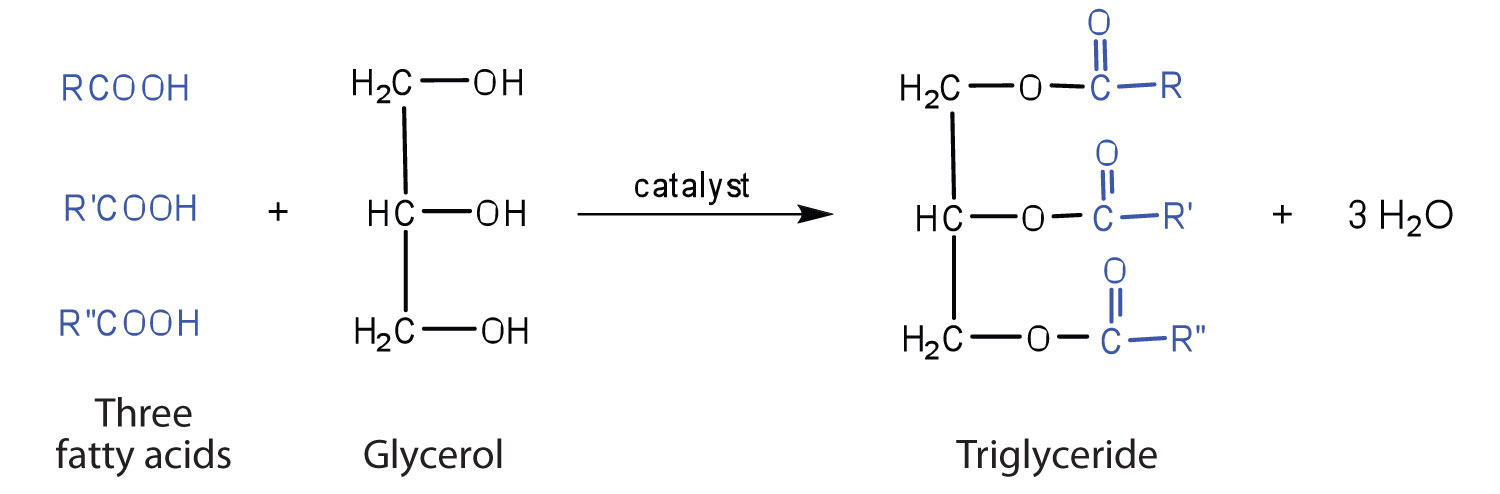
If all three OH groups on the glycerol molecule are esterified with the same fatty acid, the resulting ester is called a simple triglyceride. Although simple triglycerides have been synthesized in the laboratory, they rarely occur in nature. Instead, a typical triglyceride obtained from naturally occurring fats and oils contains two or three different fatty acid components and is thus termed a mixed triglyceride. Examples of both can be seen in Figure 28.2g.
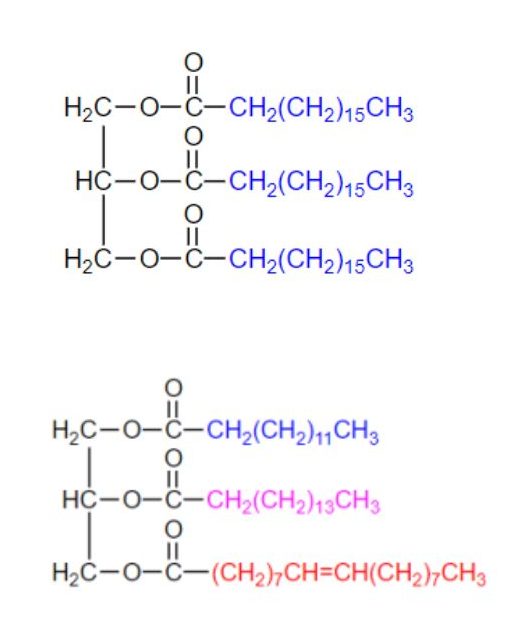
A triglyceride is called a fat if it is a solid at 25°C; it is called an oil if it is a liquid at that temperature. These differences in melting points reflect differences in the degree of unsaturation and number of carbon atoms in the constituent fatty acids. Triglycerides obtained from animal sources are usually solids, while those of plant origin are generally oils. Therefore, we commonly speak of animal fats and vegetable oils.
No single formula can be written to represent the naturally occurring fats and oils because they are highly complex mixtures of triglycerides in which many different fatty acids are represented. Table 28.2b. shows the fatty acid compositions of some common fats and oils. The composition of any given fat or oil can vary depending on the plant or animal species it comes from as well as on dietetic and climatic factors. To cite just one example, lard from corn-fed hogs is more highly saturated than lard from peanut-fed hogs. Palmitic acid is the most abundant of the saturated fatty acids, while oleic acid is the most abundant unsaturated fatty acid.
Fat/Oil | Lauric | Myristic | Palmitic | Stearic | Oleic | Linoleic | Linolenic |
---|---|---|---|---|---|---|---|
Fat – butter (cow) | 3 | 11 | 27 | 12 | 29 | 2 | 1 |
Fat – tallow | 3 | 24 | 19 | 43 | 3 | 1 | |
Fat – lard | 2 | 26 | 14 | 44 | 10 | ||
canola oil | 4 | 2 | 62 | 22 | 10 | ||
coconut oil† | 47 | 18 | 9 | 3 | 6 | 2 | |
corn oil | 11 | 2 | 28 | 58 | 1 | ||
olive oil | 13 | 3 | 71 | 10 | 1 | ||
peanut oil | 11 | 2 | 48 | 32 | |||
soybean oil | 11 | 4 | 24 | 54 | 7 | ||
*Totals less than 100% indicate the presence of fatty acids with fewer than 12 carbon atoms or more than 18 carbon atoms. | |||||||
†Coconut oil is highly saturated. It contains an unusually high percentage of the low-melting C8, C10, and C12 saturated fatty acids. |
Source: “17.2: Fats and Oils” In Basics of GOB Chemistry (Ball et al.), CC BY-NC-SA 4.0.
Terms such as saturated fat or unsaturated oil are often used to describe the fats or oils obtained from foods. Saturated fats contain a high proportion of saturated fatty acids, while unsaturated oils contain a high proportion of unsaturated fatty acids. The high consumption of saturated fats is a factor, along with the high consumption of cholesterol, in increased risks of heart disease.
Physical Properties of Fats and Oils
Contrary to what you might expect, pure fats and oils are colourless, odourless, and tasteless. The characteristic colours, odours, and flavours that we associate with some of them are imparted by foreign substances that are lipid soluble and have been absorbed by these lipids. For example, the yellow color of butter is due to the presence of the pigment carotene; the taste of butter comes from two compounds—diacetyl and 3-hydroxy-2-butanone—produced by bacteria in the ripening cream from which the butter is made (Figure 28.2h.).

Fats and oils are lighter than water, having densities of about 0.8 g/cm3. They are poor conductors of heat and electricity and therefore serve as excellent insulators for the body, slowing the loss of heat through the skin.
Chemical Reactions of Fats and Oils
Fats and oils can participate in a variety of chemical reactions—for example, because triglycerides are esters, they can be hydrolyzed in the presence of an acid, a base, or specific enzymes known as lipases. The hydrolysis of fats and oils in the presence of a base is used to make soap and is called saponification. Today most soaps are prepared through the hydrolysis of triglycerides (often from tallow, coconut oil, or both) using water under high pressure and temperature [700 lb/in2 (∼50 atm or 5,000 kPa) and 200°C]. Sodium carbonate or sodium hydroxide is then used to convert the fatty acids to their sodium salts (soap molecules), as seen in Figure 28.2i.
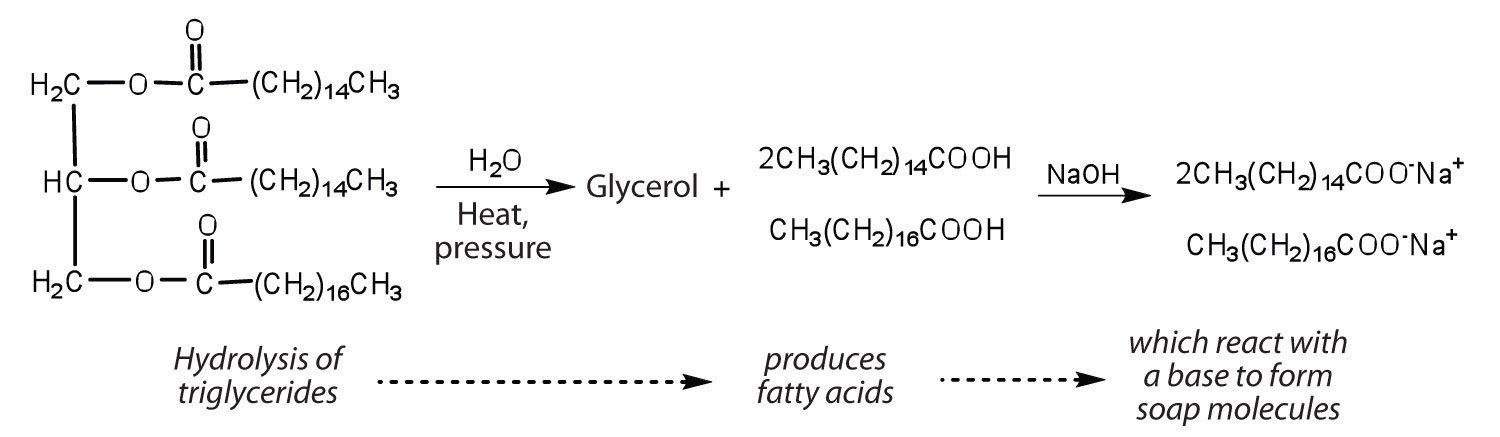
Spotlight on Everyday Chemistry: Soaps
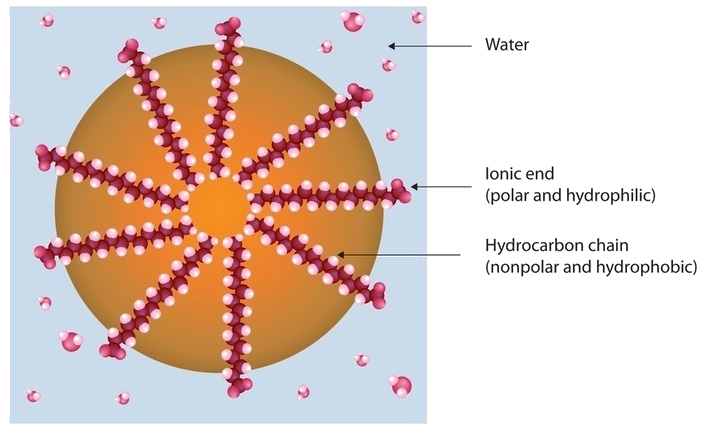
Ordinary soap is a mixture of the sodium salts of various fatty acids, produced in one of the oldest organic syntheses practiced by humans (second only to the fermentation of sugars to produce ethyl alcohol). Both the Phoenicians (600 BCE) and the Romans made soap from animal fat and wood ash. Even so, the widespread production of soap did not begin until the 1700s. Soap was traditionally made by treating molten lard or tallow with a slight excess of alkali in large open vats. The mixture was heated, and steam was bubbled through it. After saponification was completed, the soap was precipitated from the mixture by the addition of sodium chloride (NaCl), removed by filtration, and washed several times with water. It was then dissolved in water and reprecipitated by the addition of more NaCl. The glycerol produced in the reaction was also recovered from the aqueous wash solutions.
Pumice or sand is added to produce scouring soap, while ingredients such as perfumes or dyes are added to produce fragrant, coloured soaps. Blowing air through molten soap produces a floating soap. Soft soaps, made with potassium salts, are more expensive but produce a finer lather and are more soluble. They are used in liquid soaps, shampoos, and shaving creams.
Dirt and grime usually adhere to skin, clothing, and other surfaces by combining with body oils, cooking fats, lubricating greases, and similar substances that act like glues. Because these substances are not miscible in water, washing with water alone does little to remove them. Soap removes them, however, because soap molecules have a dual nature. One end, called the head, carries an ionic charge (a carboxylate anion) and therefore dissolves in water; the other end, the tail, has a hydrocarbon structure and dissolves in oils (Figure 28.2j.). The hydrocarbon tails dissolve in the soil; the ionic heads remain in the aqueous phase, and the soap breaks the oil into tiny soap-enclosed droplets called micelles, which disperse throughout the solution. The droplets repel each other because of their charged surfaces and do not coalesce. With the oil no longer “gluing” the dirt to the soiled surface (skin, cloth, dish), the soap-enclosed dirt can easily be rinsed away.
The double bonds in fats and oils can undergo hydrogenation and also oxidation. The hydrogenation of vegetable oils to produce semisolid fats is an important process in the food industry. Chemically, it is essentially identical to the catalytic hydrogenation reaction described for alkenes, as seen in Figure 28.2k.

In commercial processes, the number of double bonds that are hydrogenated is carefully controlled to produce fats with the desired consistency (soft and pliable). Inexpensive and abundant vegetable oils (canola, corn, soybean) are thus transformed into margarine and cooking fats. In the preparation of margarine, for example, partially hydrogenated oils are mixed with water, salt, and nonfat dry milk, along with flavouring agents, colouring agents, and vitamins A and D, which are added to approximate the look, taste, and nutrition of butter. (Preservatives and antioxidants are also added.) In most commercial peanut butter, the peanut oil has been partially hydrogenated to prevent it from separating out. Consumers could decrease the amount of saturated fat in their diet by using the original unprocessed oils on their foods, but most people would rather spread margarine on their toast than pour oil on it.
Many people have switched from butter to margarine or vegetable shortening because of concerns that saturated animal fats can raise blood cholesterol levels and result in clogged arteries. However, during the hydrogenation of vegetable oils, an isomerization reaction occurs that produces the trans fatty acids. However, studies have shown that trans fatty acids also raise cholesterol levels and increase the incidence of heart disease. Trans fatty acids do not have the bend in their structures, which occurs in cis fatty acids and thus pack closely together in the same way that the saturated fatty acids do. Consumers are now being advised to use polyunsaturated oils and soft or liquid margarine and reduce their total fat consumption to less than 30% of their total calorie intake each day.
Fats and oils that are in contact with moist air at room temperature eventually undergo oxidation and hydrolysis reactions that cause them to turn rancid, acquiring a characteristic disagreeable odour. One cause of the odour is the release of volatile fatty acids by hydrolysis of the ester bonds. Butter, for example, releases foul-smelling butyric, caprylic, and capric acids. Microorganisms present in the air furnish lipases that catalyze this process. Hydrolytic rancidity can easily be prevented by covering the fat or oil and keeping it in a refrigerator.
Rancidity is a major concern of the food industry, which is why food chemists are always seeking new and better antioxidants, substances added in very small amounts (0.001%–0.01%) to prevent oxidation and thus suppress rancidity. Antioxidants are compounds whose affinity for oxygen is greater than that of the lipids in the food; thus they function by preferentially depleting the supply of oxygen absorbed into the product. Because vitamin E has antioxidant properties, it helps reduce damage to lipids in the body, particularly to unsaturated fatty acids found in cell membrane lipids.
Membranes and Membrane Lipids
All living cells are surrounded by a cell membrane. Plant cells (Figure 28.2l., top) and animal cells (Figure 28.2l., bottom) contain a cell nucleus that is also surrounded by a membrane and holds the genetic information for the cell. Everything between the cell membrane and the nuclear membrane—including intracellular fluids and various subcellular components such as the mitochondria and ribosomes—is called the cytoplasm. The membranes of all cells have a fundamentally similar structure, but membrane function varies tremendously from one organism to another and even from one cell to another within a single organism. This diversity arises mainly from the presence of different proteins and lipids in the membrane.
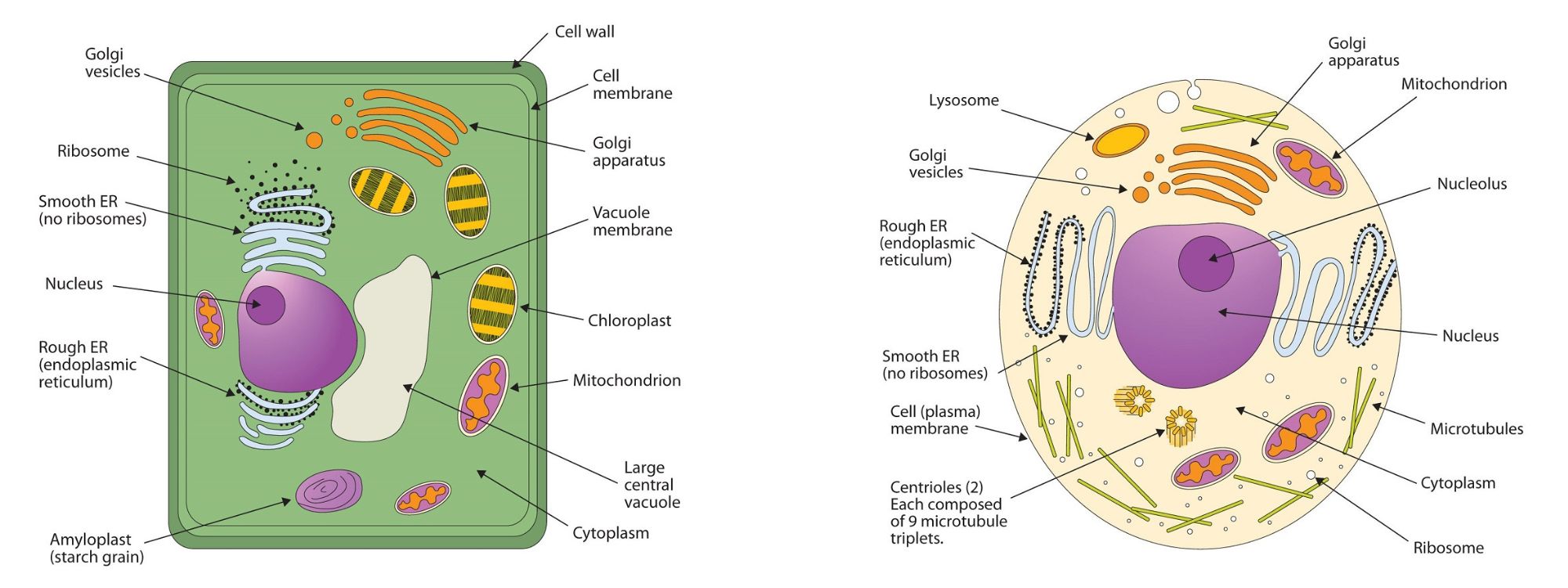
The lipids in cell membranes are highly polar but have dual characteristics: part of the lipid is ionic and therefore dissolves in water, whereas the rest has a hydrocarbon structure and therefore dissolves in nonpolar substances. Often, the ionic part is referred to as hydrophilic, meaning “water loving,” and the nonpolar part as hydrophobic, meaning “water fearing” (repelled by water). When allowed to float freely in water, polar lipids spontaneously cluster together in any one of three arrangements: micelles, monolayers, and bilayers, as seen in Figure 28.2m.
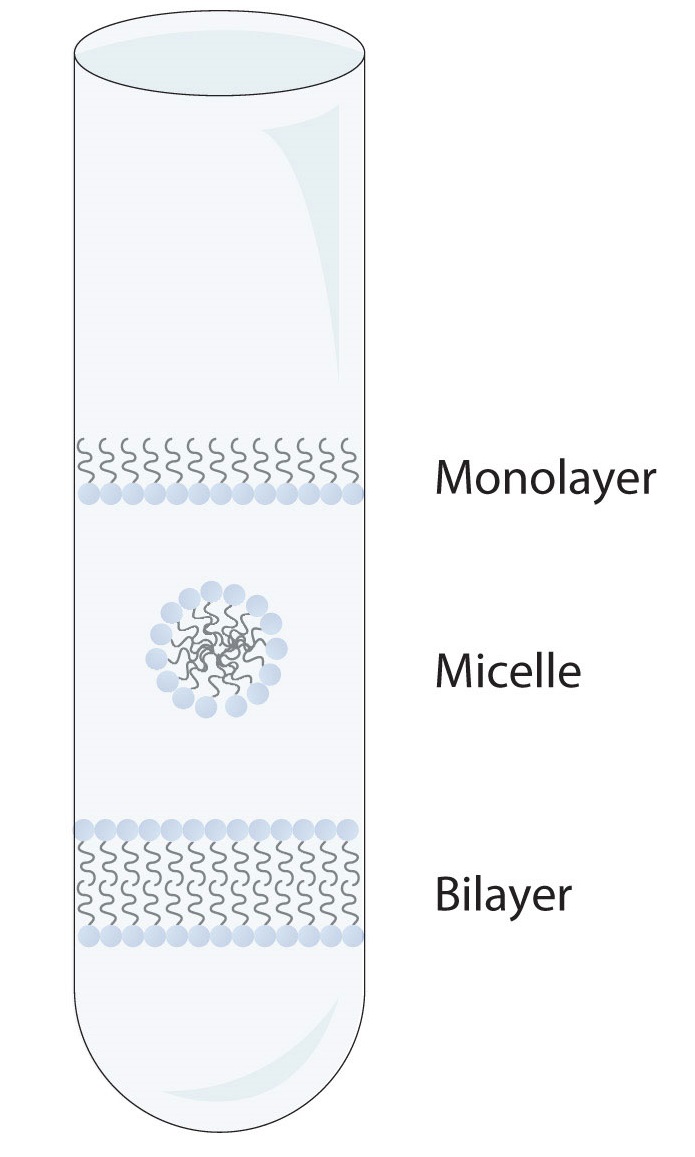
Micelles are aggregations in which the lipids’ hydrocarbon tails—being hydrophobic—are directed toward the center of the assemblage and away from the surrounding water while the hydrophilic heads are directed outward, in contact with the water. Each micelle may contain thousands of lipid molecules. Polar lipids may also form a monolayer, a layer one molecule thick on the surface of the water. The polar heads face into water, and the nonpolar tails stick up into the air. Bilayers are double layers of lipids arranged so that the hydrophobic tails are sandwiched between an inner surface and an outer surface consisting of hydrophilic heads. The hydrophilic heads are in contact with water on either side of the bilayer, whereas the tails, sequestered inside the bilayer, are prevented from having contact with the water. Bilayers like this make up every cell membrane (Figure 28.2n.).
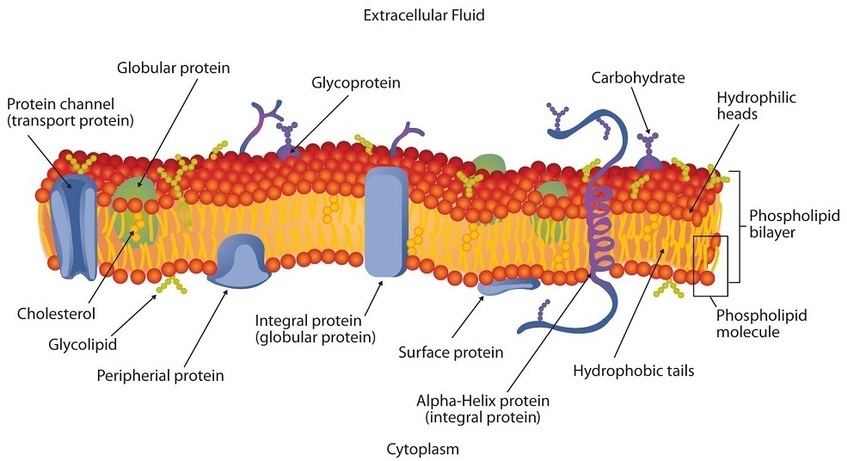
In the bilayer interior, the hydrophobic tails (that is, the fatty acid portions of lipid molecules) interact by means of dispersion forces. The interactions are weakened by the presence of unsaturated fatty acids. As a result, the membrane components are free to mill about to some extent, and the membrane is described as fluid.
Exercise 28.2a
Using your knowledge of functional groups and their physical properties, explain why membrane lipids have water loving and water fearing regions.
Check Your Answer:[1]
Source: Exercise 28.2a by Samantha Sullivan Sauer, licensed under CC BY-NC 4.0
The lipids found in cell membranes can be categorized in various ways. Phospholipids are lipids containing phosphorus. Glycolipids are sugar-containing lipids. The latter are found exclusively on the outer surface of the cell membrane, acting as distinguishing surface markers for the cell and thus serving in cellular recognition and cell-to-cell communication. Sphingolipids are phospholipids or glycolipids that contain the unsaturated amino alcohol sphingosine rather than glycerol. Diagrammatic structures of representative membrane lipids are presented in Figure 28.2o.
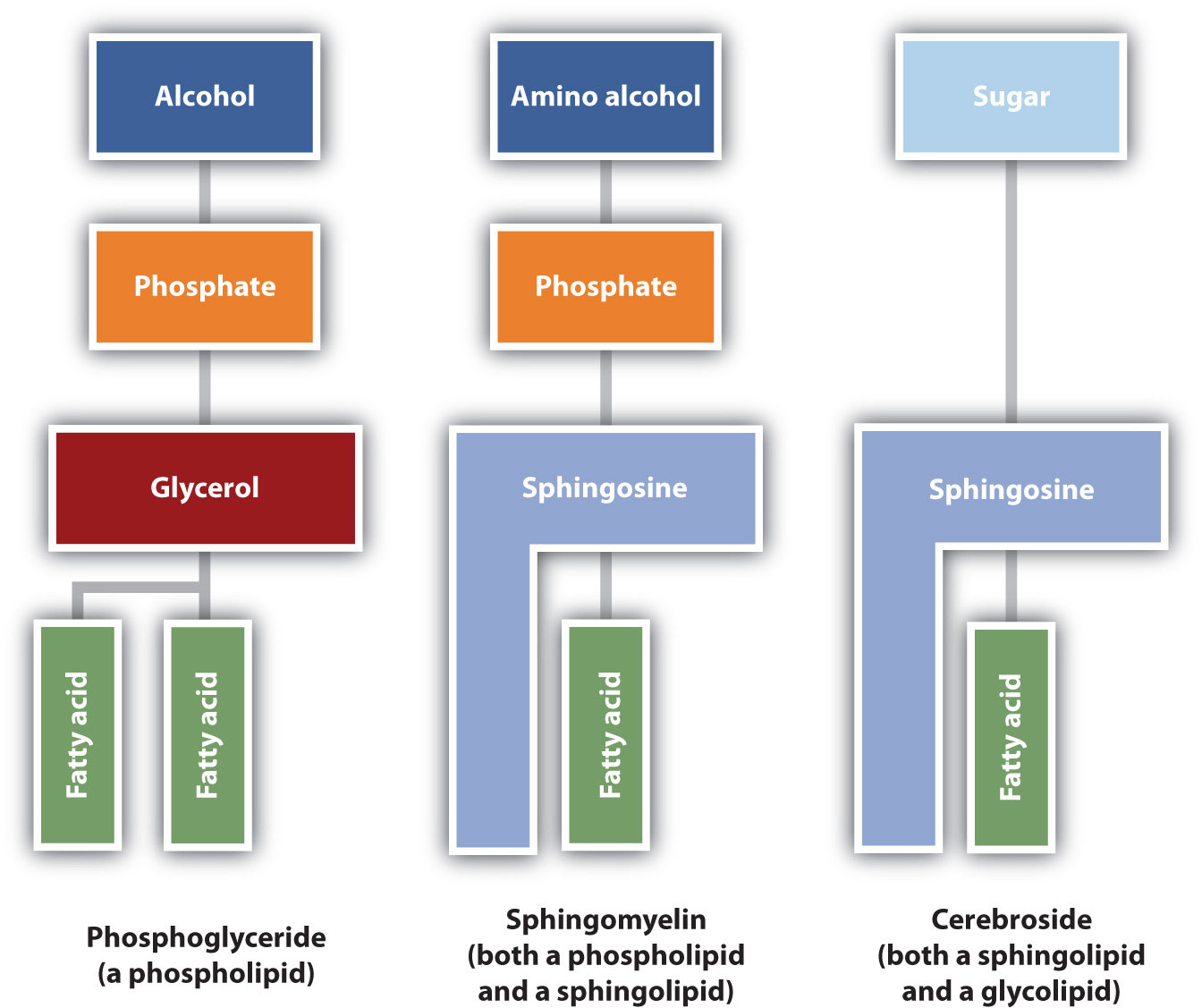
Phosphoglycerides (also known as glycerophospholipids) are the most abundant phospholipids in cell membranes. They consist of a glycerol unit with fatty acids attached to the first two carbon atoms, while a phosphoric acid unit, esterified with an alcohol molecule (usually an amino alcohol, as in part (a) of Figure 28.2p.) is attached to the third carbon atom of glycerol (part (b) of Figure 28.12e.). Notice that the phosphoglyceride molecule is identical to a triglyceride up to the phosphoric acid unit (part (b) of Figure 28.2p.).
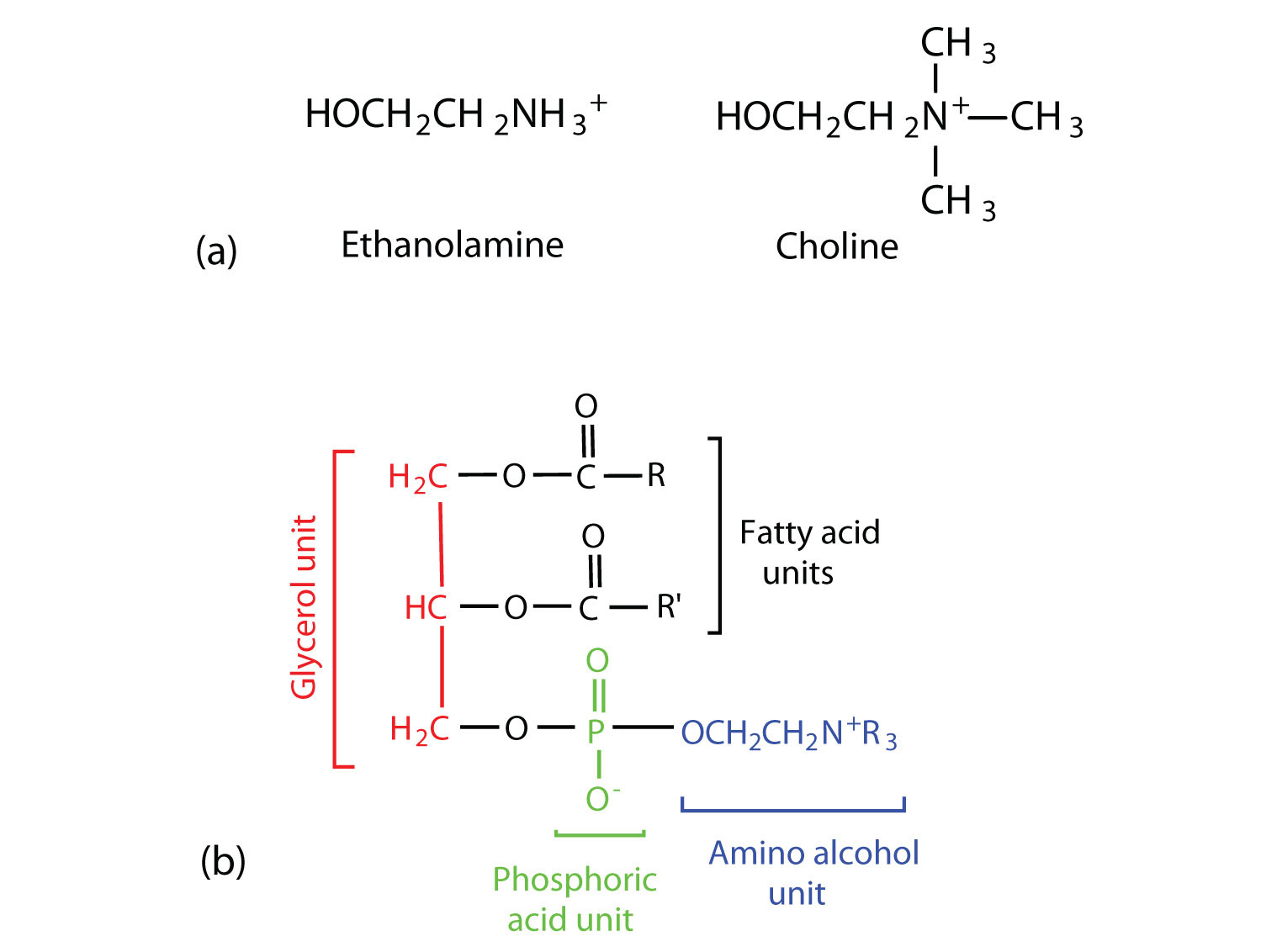
There are two common types of phosphoglycerides. Phosphoglycerides containing ethanolamine as the amino alcohol are called phosphatidylethanolamines or cephalins. Cephalins are found in brain tissue and nerves and also have a role in blood clotting (Figure 28.2q.). Phosphoglycerides containing choline as the amino alcohol unit are called phosphatidylcholines or lecithins. Lecithins occur in all living organisms (Figure 28.2q.). Like cephalins, they are important constituents of nerve and brain tissue. Egg yolks are especially rich in lecithins. Commercial-grade lecithins isolated from soybeans are widely used in foods as emulsifying agents. An emulsifying agent is used to stabilize an emulsion—a dispersion of two liquids that do not normally mix, such as oil and water. Many foods are emulsions. Milk is an emulsion of butterfat in water. The emulsifying agent in milk is a protein called casein. Mayonnaise is an emulsion of salad oil in water, stabilized by lecithins present in egg yolk.
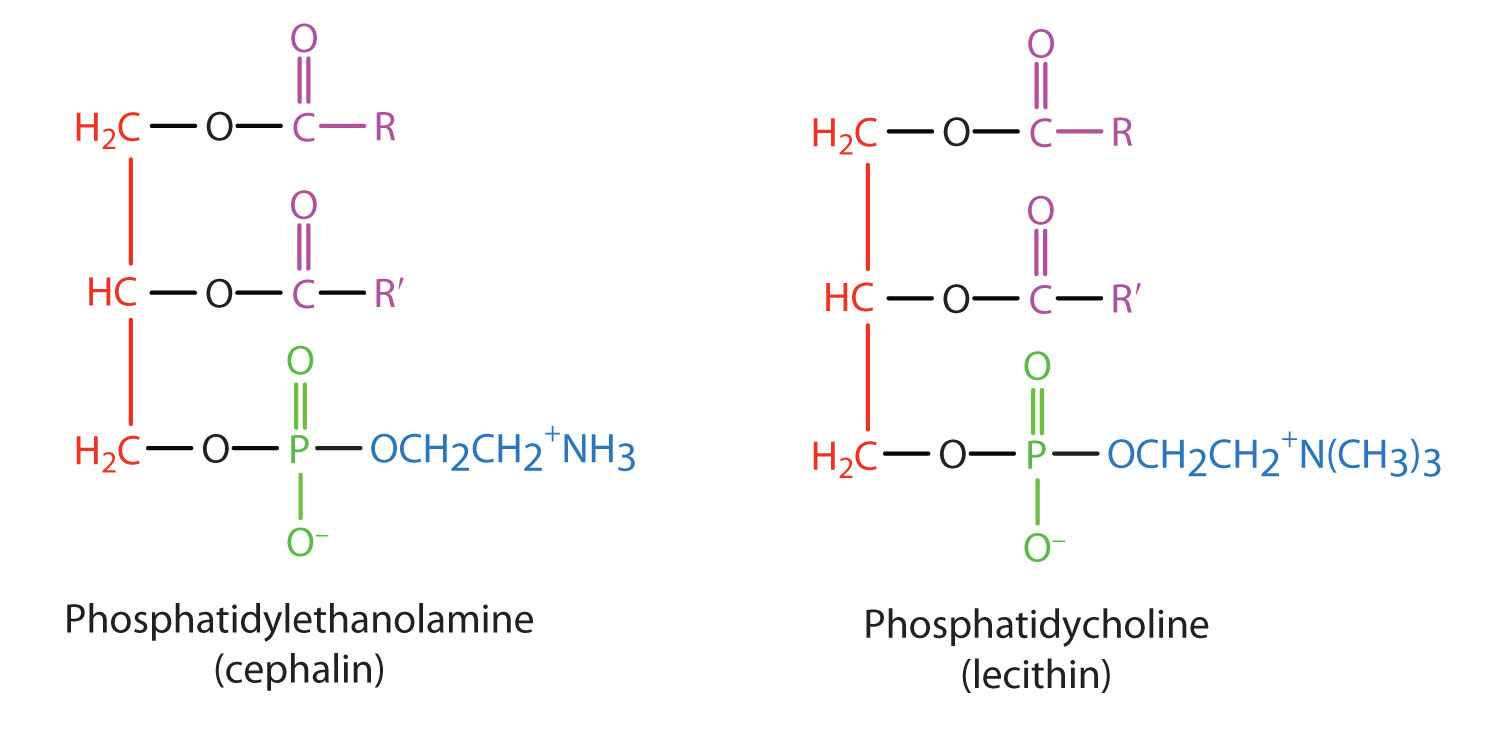
Sphingomyelins, the simplest sphingolipids, each contain a fatty acid, a phosphoric acid, sphingosine, and choline (Figure 28.2r.). Because they contain phosphoric acid, they are also classified as phospholipids. Sphingomyelins are important constituents of the myelin sheath surrounding the axon of a nerve cell. Multiple sclerosis is one of several diseases resulting from damage to the myelin sheath.
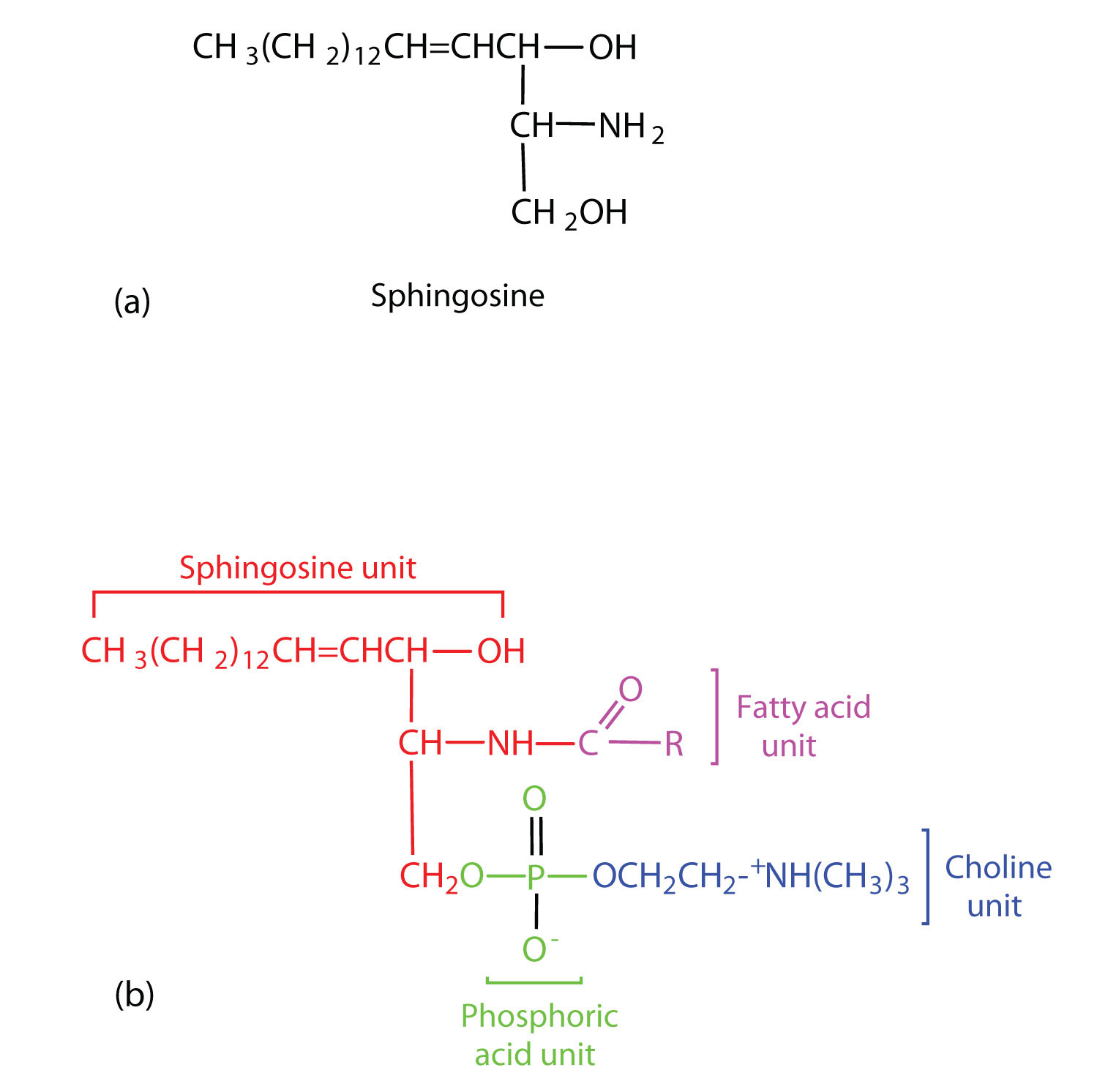
Most animal cells contain sphingolipids called cerebrosides (Figure 28.2s.). Cerebrosides are composed of sphingosine, a fatty acid, and galactose or glucose. They therefore resemble sphingomyelins but have a sugar unit in place of the choline phosphate group. Cerebrosides are important constituents of the membranes of nerve and brain cells.
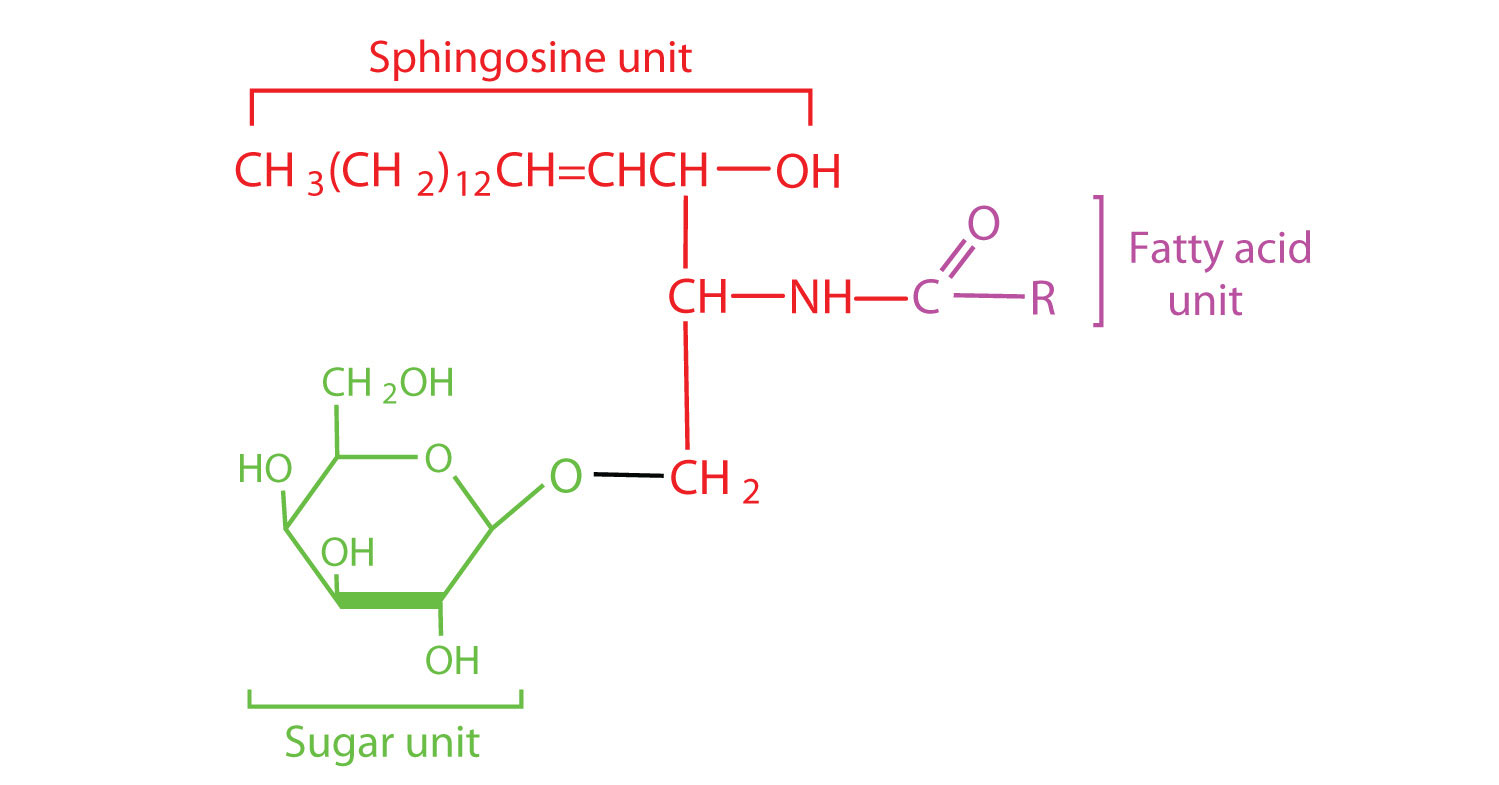
The sphingolipids called gangliosides are more complex, usually containing a branched chain of three to eight monosaccharides and/or substituted sugars. Because of considerable variation in their sugar components, about 130 varieties of gangliosides have been identified. Most cell-to-cell recognition and communication processes (e.g., blood group antigens) depend on differences in the sequences of sugars in these compounds. Gangliosides are most prevalent in the outer membranes of nerve cells, although they also occur in smaller quantities in the outer membranes of most other cells. Because cerebrosides and gangliosides contain sugar groups, they are also classified as glycolipids.
Membrane Proteins
If membranes were composed only of lipids, very few ions or polar molecules could pass through their hydrophobic “sandwich filling” to enter or leave any cell. However, certain charged and polar species do cross the membrane, aided by proteins that move about in the lipid bilayer. The two major classes of proteins in the cell membrane are integral proteins, which span the hydrophobic interior of the bilayer, and peripheral proteins, which are more loosely associated with the surface of the lipid bilayer (Figure 28.2c.). Peripheral proteins may be attached to integral proteins, to the polar head groups of phospholipids, or to both by hydrogen bonding and electrostatic forces.
Small ions and molecules soluble in water enter and leave the cell by way of channels through the integral proteins. Some proteins, called carrier proteins, facilitate the passage of certain molecules, such as hormones and neurotransmitters, by specific interactions between the protein and the molecule being transported.
Steroids
All the lipids discussed so far are saponifiable, reacting with aqueous alkali to yield simpler components, such as glycerol, fatty acids, amino alcohols, and sugars. Lipid samples extracted from cellular material, however, also contain a small but important fraction that does not react with alkali. The most important nonsaponifiable lipids are the steroids. These compounds include the bile salts, cholesterol and related compounds, and certain hormones (such as cortisone and the sex hormones).
Steroids occur in plants, animals, yeasts, and molds but not in bacteria. They may exist in free form or combined with fatty acids or carbohydrates. All steroids have a characteristic structural component consisting of four fused rings. Chemists identify the rings by capital letters and number the carbon atoms as shown in Figure 28.2t. Slight variations in this structure or in the atoms or groups attached to it produce profound differences in biological activity.
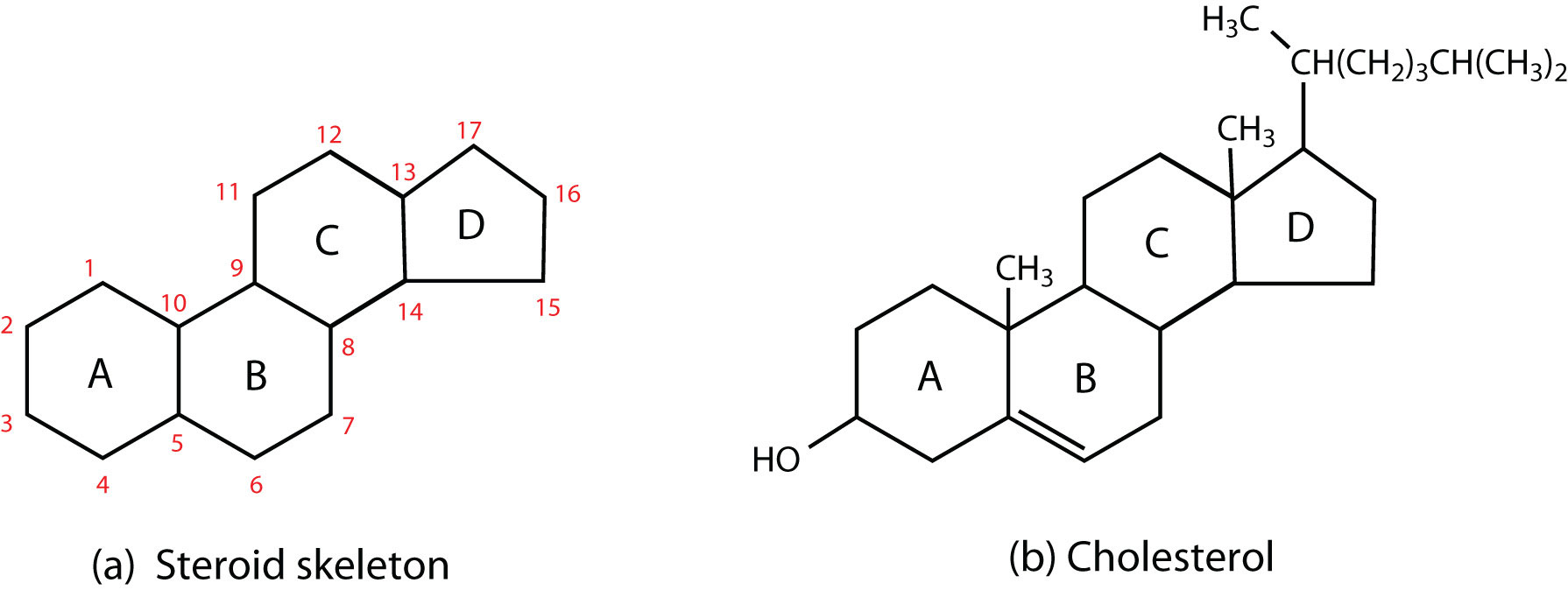
Cholesterol
Cholesterol (Figure 28.2t., part b) does not occur in plants, but it is the most abundant steroid in the human body (240 g is a typical amount). Excess cholesterol is believed to be a primary factor in the development of atherosclerosis and heart disease, which are major health problems in the United States and Canada today. About half of the body’s cholesterol is interspersed in the lipid bilayer of cell membranes. Much of the rest is converted to cholic acid, which is used in the formation of bile salts. Cholesterol is also a precursor in the synthesis of sex hormones, adrenal hormones, and vitamin D.
Excess cholesterol not metabolized by the body is released from the liver and transported by the blood to the gallbladder. Normally, it stays in solution there until being secreted into the intestine (as a component of bile) to be eliminated. Sometimes, however, cholesterol in the gallbladder precipitates in the form of gallstones (Figure 28.2u). Indeed, the name cholesterol is derived from the Greek chole, meaning “bile,” and stereos, meaning “solid.”
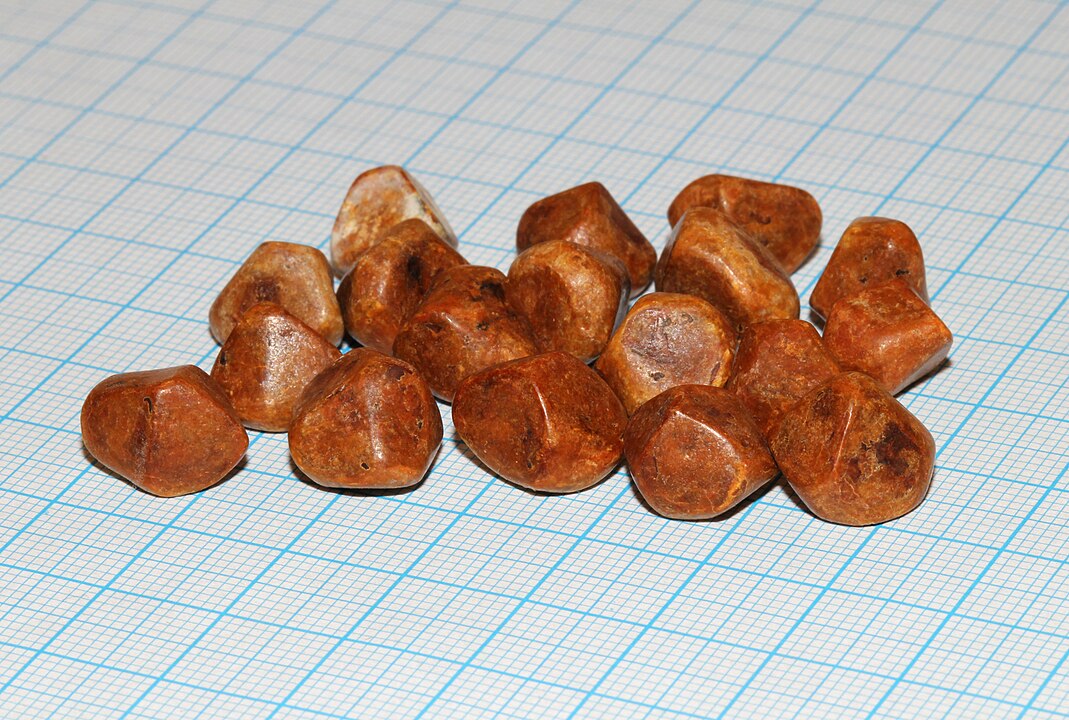
Exercise 28.2b
Is cholesterol water soluble? Explain.
Check Your Answer:[2]
Source: Exercise 28.2b by Samantha Sullivan Sauer, licensed under CC BY-NC 4.0
Heart disease is the leading cause of death in the United States and Canada for both men and women. The Centers for Disease Control and Prevention reported that heart disease claimed 631,636 lives in the United States (26% of all reported deaths) in 2006.
Scientists agree that elevated cholesterol levels in the blood, as well as high blood pressure, obesity, diabetes, and cigarette smoking, are associated with an increased risk of heart disease. A long-term investigation by the National Institutes of Health showed that among men ages 30 to 49, the incidence of heart disease was five times greater for those whose cholesterol levels were above 260 mg/100 mL of serum than for those with cholesterol levels of 200 mg/100 mL or less. The cholesterol content of blood varies considerably with age, diet, and sex. Young adults average about 170 mg of cholesterol per 100 mL of blood, whereas males at age 55 may have cholesterol levels at 250 mg/100 mL or higher because the rate of cholesterol breakdown decreases with age. Females tend to have lower blood cholesterol levels than males.
To understand the link between heart disease and cholesterol levels, it is important to understand how cholesterol and other lipids are transported in the body. Lipids, such as cholesterol, are not soluble in water and therefore cannot be transported in the blood (an aqueous medium) unless they are complexed with proteins that are soluble in water, forming assemblages called lipoproteins (Figure 28.2v.). Lipoproteins are classified according to their density, which is dependent on the relative amounts of protein and lipid they contain. Lipids are less dense than proteins, so lipoproteins containing a greater proportion of lipid are less dense than those containing a greater proportion of protein.
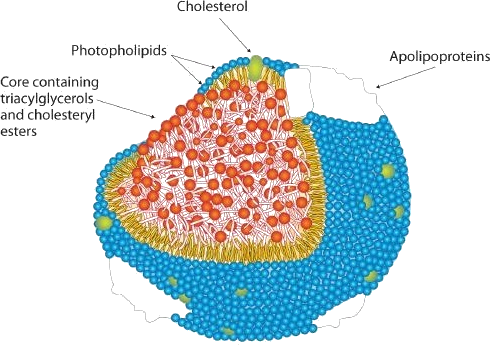
Research on cholesterol and its role in heart disease has focused on serum levels of low-density lipoproteins (LDLs) and high-density lipoproteins (HDLs). One of the most fascinating discoveries is that high levels of HDLs reduce a person’s risk of developing heart disease, whereas high levels of LDLs increase that risk. Thus the serum LDL:HDL ratio is a better predictor of heart disease risk than the overall level of serum cholesterol. Persons who, because of hereditary or dietary factors, have high LDL:HDL ratios in their blood have a higher incidence of heart disease.
How do HDLs reduce the risk of developing heart disease? No one knows for sure, but one role of HDLs appears to be the transport of excess cholesterol to the liver, where it can be metabolized. Therefore, HDLs aid in removing cholesterol from blood and from the smooth muscle cells of the arterial wall.
Dietary modifications and increased physical activity can help lower total cholesterol and improve the LDL:HDL ratio. The average American consumes about 600 mg of cholesterol from animal products each day and also synthesizes approximately 1 g of cholesterol each day, mostly in the liver. The amount of cholesterol synthesized is controlled by the cholesterol level in the blood; when the blood cholesterol level exceeds 150 mg/100 mL, the rate of cholesterol biosynthesis is halved. Hence, if cholesterol is present in the diet, a feedback mechanism suppresses its synthesis in the liver. However, the ratio of suppression is not a 1:1 ratio; the reduction in biosynthesis does not equal the amount of cholesterol ingested. Thus, dietary substitutions of unsaturated fat for saturated fat, as well as a reduction in consumption of trans fatty acids, is recommended to help lower serum cholesterol and the risk of heart disease.
Steroid Hormones
Hormones are chemical messengers that are released in one tissue and transported through the circulatory system to one or more other tissues. One group of hormones is known as steroid hormones because these hormones are synthesized from cholesterol, which is also a steroid. There are two main groups of steroid hormones: adrenocortical hormones and sex hormones.
The adrenocortical hormones, such as aldosterone and cortisol (Table 28.2c.), are produced by the adrenal gland, which is located adjacent to each kidney. Aldosterone acts on most cells in the body, but it is particularly effective at enhancing the rate of reabsorption of sodium ions in the kidney tubules and increasing the secretion of potassium ions and/or hydrogen ions by the tubules. Because the concentration of sodium ions is the major factor influencing water retention in tissues, aldosterone promotes water retention and reduces urine output. Cortisol regulates several key metabolic reactions (for example, increasing glucose production and mobilizing fatty acids and amino acids). It also inhibits the inflammatory response of tissue to injury or stress. Cortisol and its analogs are therefore used pharmacologically as immunosuppressants after transplant operations and in the treatment of severe skin allergies and autoimmune diseases, such as rheumatoid arthritis.
Hormone | Effect |
---|---|
![]() |
regulates salt metabolism; stimulates kidneys to retain sodium and excrete potassium |
![]() |
stimulates the conversion of proteins to carbohydrates |
![]() |
regulates the menstrual cycle; maintains pregnancy |
![]() |
stimulates female sex characteristics; regulates changes during the menstrual cycle |
![]() |
stimulates and maintains male sex characteristics |
The sex hormones are a class of steroid hormones secreted by the gonads (ovaries or testes), the placenta, and the adrenal glands. Testosterone and androstenedione are the primary male sex hormones, or androgens, controlling the primary sexual characteristics of males, or the development of the male genital organs and the continuous production of sperm. Androgens are also responsible for the development of secondary male characteristics, such as facial hair, deep voice, and muscle strength. Two kinds of sex hormones are of particular importance in females: progesterone, which prepares the uterus for pregnancy and prevents the further release of eggs from the ovaries during pregnancy, and the estrogens, which are mainly responsible for the development of female secondary sexual characteristics, such as breast development and increased deposition of fat tissue in the breasts, the buttocks, and the thighs. Both males and females produce androgens and estrogens, differing in the amounts of secreted hormones rather than in the presence or absence of one or the other.
Sex hormones, both natural and synthetic, are sometimes used therapeutically. For example, a woman who has had her ovaries removed may be given female hormones to compensate. Some of the earliest chemical compounds employed in cancer chemotherapy were sex hormones. For example, estrogens are one treatment option for prostate cancer because they block the release and activity of testosterone. Testosterone enhances prostate cancer growth. Sex hormones are also administered in preparation for sex-change operations, to promote the development of the proper secondary sexual characteristics. Oral contraceptives are synthetic derivatives of the female sex hormones; they work by preventing ovulation.
Bile Salts
Bile is a yellowish green liquid (pH 7.8–8.6) produced in the liver. The most important constituents of bile are bile salts, which are sodium salts of amide like combinations of bile acids, such as cholic acid (part (a) of Figure 28.2w.) and an amine such as the amino acid glycine (part (b) of Figure 28.2w.). They are synthesized from cholesterol in the liver, stored in the gallbladder, and then secreted in bile into the small intestine. In the gallbladder, the composition of bile gradually changes as water is absorbed and the other components become more concentrated.
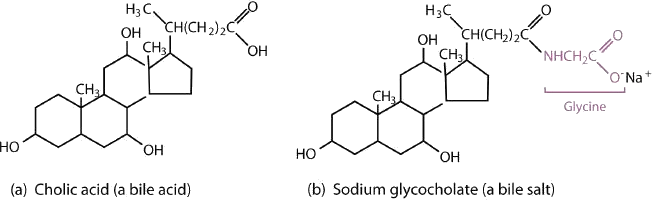
Because they contain both hydrophobic and hydrophilic groups, bile salts are highly effective detergents and emulsifying agents; they break down large fat globules into smaller ones and keep those smaller globules suspended in the aqueous digestive environment. Enzymes can then hydrolyze fat molecules more efficiently. Thus, the major function of bile salts is to aid in the digestion of dietary lipids.
Surgical removal is often advised for a gallbladder that becomes infected, inflamed, or perforated. This surgery does not seriously affect digestion because bile is still produced by the liver, but the liver’s bile is more dilute and its secretion into the small intestine is not as closely tied to the arrival of food.
Attribution & References
- Except where otherwise noted, portions of this page were written by Gregory A. Anderson while others were adapted by Gregory A. Anderson and Samantha Sullivan Sauer from “17.0: Prelude to Lipids“, “17.1: Fatty Acids“, “17.2: Fats and Oils“, “17.3: Membranes and Membrane Lipids“, “17.4: Steroids” In Basics of General, Organic, and Biological Chemistry (Ball et al.) by David W. Ball, John W. Hill, and Rhonda J. Scott via LibreTexts, CC BY-NC-SA 4.0./ A LibreTexts version of Introduction to Chemistry: GOB (v. 1.0), CC BY-NC 3.0. / Pages combined and streamlined for student understanding.
-
Hydrophilic means water loving. In order to be soluble in water, a structure must be polar. The carboxylic acid ends of lipids are polar and thus will interact with water. Hydrophobic means water fearing. In order to be insoluble in water, a structure must be essentially non-polar. The hydrocarbon ends of lipids are non-polar and thus will not interact with water.
↵
-
Cholesterol is not water soluble. Its structure is predominantly hydrocarbon based and non-polar resulting in very limited (if any) ability to interact with water.
↵