27.6 Condensation Polymers
Learning Objectives
By the end of this section, you will be able to:
- Identify condensation polymerization.
- Identify some common condensation polymers and their properties and uses.
A large number of important and useful polymeric materials are not formed by addition polymerization but proceed instead by conventional functional group transformations of polyfunctional reactants. These polymerizations often occur with loss of a small byproduct, such as water, and generally combine two different components in an alternating structure. The polyester Dacron and the polyamide Nylon 66 are two examples of synthetic condensation polymers.
Condensation polymerization (also known as step-growth) requires that the monomers possess two or more kinds of functional groups that are able to react with each other in such a way that parts of these groups combine to form a small molecule (often H2O) which is eliminated from the two pieces (Figure 27.6a.). The now-empty bonding positions on the two monomers can then join together.
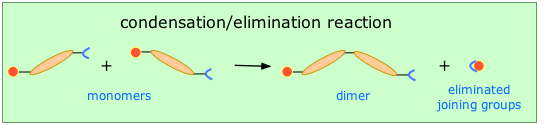
In contrast to addition polymerization, most of which grow by carbon-carbon bond formation, step-growth polymers generally grow by carbon-heteroatom bond formation (C-O & C-N in Dacron & Nylon respectively). Although polymers of this kind might be considered to be alternating copolymers, the repeating monomeric unit is usually defined as a combined moiety.
Examples of naturally occurring condensation polymers are cellulose, starch, the polypeptide chains of proteins, and poly(β-hydroxybutyric acid), a polyester synthesized in large quantity by certain soil and water bacteria. See Chapter 28 for more information on biological molecules.
Indigenous Perspectives: Inuit Materials
Through thousands of years living in the Arctic, Inuit have learned to use resources that the land provides. Many key materials such as animal skins and wood are derived from polymer based chemical structures.
Animal skin contains collagen (Figure 27.6b.) which is a protein formed from amino acid units. Each amino acid is joined to the next amino acid through a condensation reaction. Animal skin also contains muco-polysaccharide which is a condensation polymer made with sugar and amino-sugar molecules (Figure 27.6c.) (Nagitarvik, & Rayner-Canham, 2022).
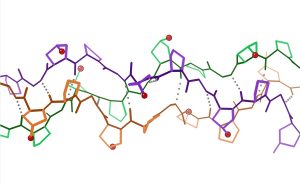
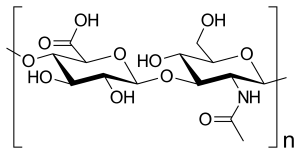
Wood contains cellulose is a condensation polymer made from repeating sugar units. Cellulose provides a rigid framework to wood (Figure 27.6d.).
For more information, read Composites in Inuit Life | Chem 13 News Magazine | University of Waterloo (uwaterloo.ca).
There are four main classes of condensation polymers: polyamides, polyesters, phenolics and polyurethanes (Hein et al, 2014).
Polyamides including Nylon
Polyamides, such as nylons, Kevlar (see Chapter 27 introduction) and proteins, are an important class of condensation polymers. They arise from the reaction of carboxylic acid and an amine. When prepared from diamines and dicarboxylic acids, the polymerization produces two molecules of water per repeat unit.
n H2N-X-NH2 + n HO2C-Y-CO2H → [HN-X-NHC(O)-Y-C(O)]n + 2n H2O
The production of nylon 66 is an example of this process (Figure 27.6e. and 27.6f.). Note that the monomeric units that make up the polymer are not identical with the starting components.
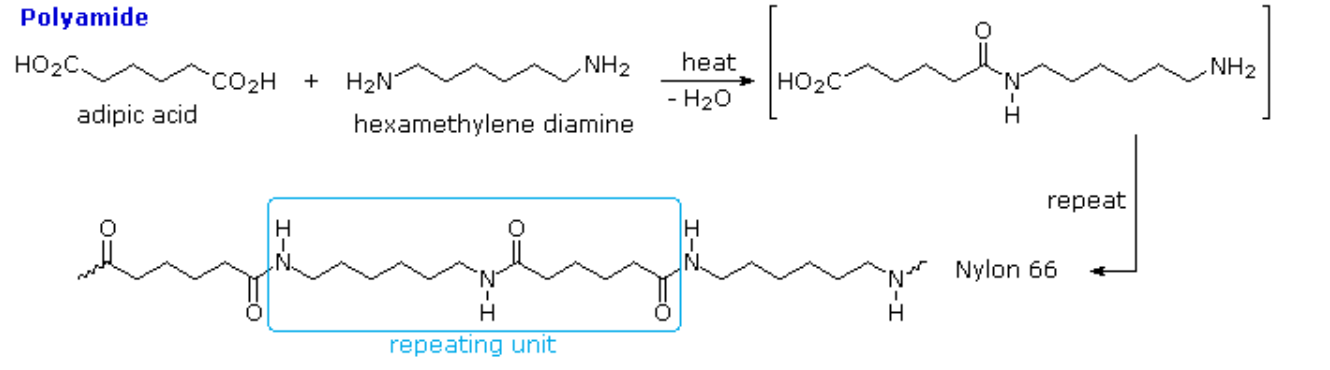
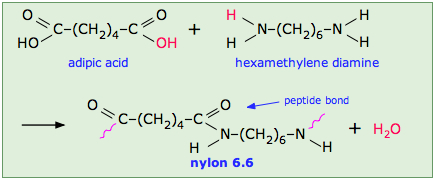
Nylon is a thermoplastic silky material that can be melt-processed into fibers, films, or shapes. It is made of repeating units linked by amide links similar to the peptide bonds in proteins. Nylon polymers can be mixed with a wide variety of additives to achieve many different property variations. Nylon polymers have found significant commercial applications in fabric and fibers (apparel, flooring and rubber reinforcement), in shapes (molded parts for cars, electrical equipment, etc.), and in films (mostly for food packaging).
Nylon was the first commercially successful synthetic thermoplastic polymer. DuPont began its research project in 1927. The first example of nylon (nylon 6,6) was produced using diamines on February 28, 1935, by Wallace Hume Carothers (Figure 27.6d.). In response to Carothers’ work, Paul Schlack at IG Farben developed nylon 6, a different molecule based on caprolactam, on January 29, 1938 (Figure 27.6g.). Notice that this already contains an amide link. When this molecule polymerizes, the ring opens, and the molecules join up in a continuous chain. Nylon 6 fibers are tough, possessing high tensile strength, as well as elasticity and lustre. They are wrinkleproof and highly resistant to abrasion and chemicals such as acids and alkalis. The fibers can absorb up to 2.4% of water, although this lowers tensile strength.
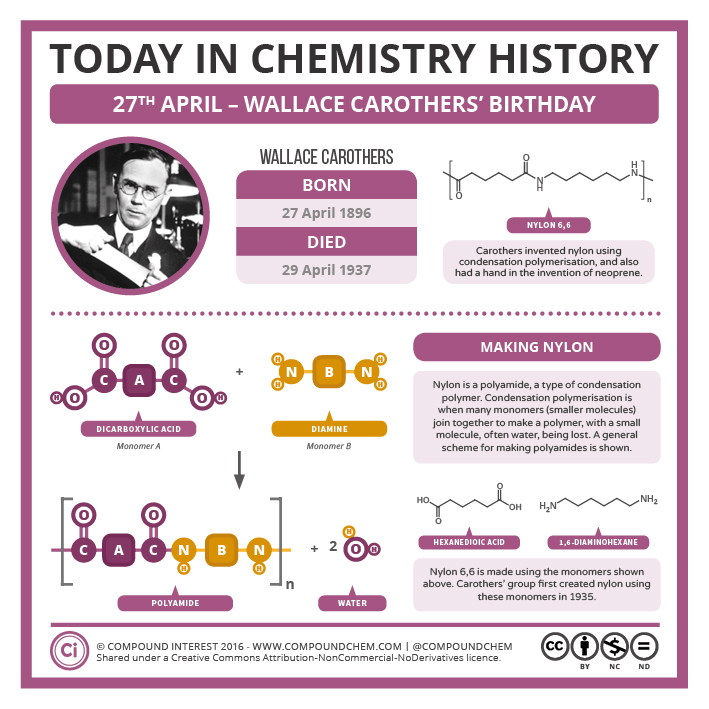
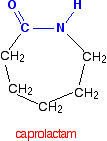
Nylon was first used commercially in a nylon-bristled toothbrush in 1938, followed more famously in women’s stockings or “nylons” which were shown at the 1939 New York World’s Fair and first sold commercially in 1940. During World War II, almost all nylon production was diverted to the military for use in parachutes and parachute cord. Wartime uses of nylon and other plastics greatly increased the market for the new materials.
Kevlar is similar in structure to nylon-6,6 except that instead of the amide links joining chains of carbon atoms together, they join benzene rings. The two monomers are benzene-1,4-dicarboxylic acid and 1,4-diaminobenzene (Figure 27.6h.). If you line these up and remove water between the -COOH and -NH2 groups in the same way as we did with nylon-6,6, you get the structure of Kevlar (Figure 27.6i.).
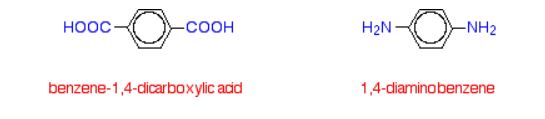
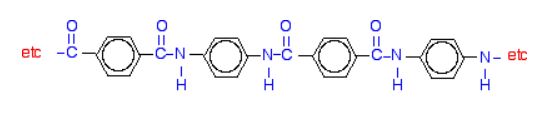
Kevlar is a registered trademark of DuPont. Kevlar’s first commercial use was as a replacement for steel in racing tires. Kevlar is typically spun into ropes or fibres. The material has a high tensile strength-to-weight ratio (it is about 5 times stronger than an equal weight of steel), making it useful for many applications from bicycle tires to sails to body armour. The material owes much of its strength to hydrogen bonds between polymer chains. These bonds form between the carbonyl group oxygen atom (which has a partial negative charge due to oxygen’s electronegativity) on one monomer and the partially positively charged hydrogen atom in the N–H bond of an adjacent monomer in the polymer structure (see dashed line in Figure 27.6j.). There is additional strength derived from the interaction between the unhybridized p orbitals in the six-membered rings, called aromatic stacking.
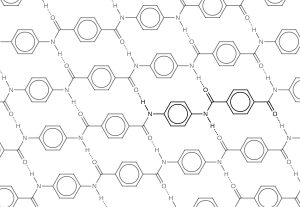
Kevlar may be best known as a component of body armour, combat helmets, and face masks. Since the 1980s, the US military has used Kevlar as a component of the PASGT (personal armour system for ground troops) helmet and vest. Kevlar is also used to protect armoured fighting vehicles and aircraft carriers. Civilian applications include protective gear for emergency service personnel such as body armour for police officers and heat-resistant clothing for fire fighters. Kevlar based clothing is considerably lighter and thinner than equivalent gear made from other materials (Figure 27.6k.). In addition to its better-known uses, Kevlar is also often used in cryogenics for its very low thermal conductivity (along with its high strength). Kevlar maintains its high strength when cooled to the temperature of liquid nitrogen (–196 °C).
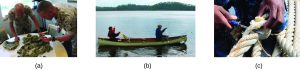
Polyesters including Polyethylene Terephthalate
Polyesters, such as Dacron, are another important condensation polymer. They arise from the reaction of carboxylic acid and an alcohol.
- n HO-X-OH + n HO2C-Y-CO2H → [O-X-O2C-Y-C(O)]n + (3n-2) H2O
The synthesis of Dacron (a polyester) is shown in Figure 27.6l. Polyethylene terephthalate (sometimes written poly(ethylene terephthalate)), commonly abbreviated PET, PETE, or the obsolete PETP or PET-P, is the most common thermoplastic polymer resin of the polyester family and is used in fibres for clothing, containers for liquids and foods, thermoforming for manufacturing, and in combination with glass fibre for engineering resins. PETE is recycling code number 1.
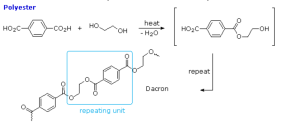
The majority of the world’s PET production is for synthetic fibers (in excess of 60%), with bottle production accounting for about 30% of global demand. In the context of textile applications, PET is referred to by its common name, polyester, whereas the acronym PET is generally used in relation to packaging. Polyester makes up about 18% of world polymer production and is the fourth-most-produced polymer after polyethylene (PE), polypropylene (PP) and polyvinyl chloride (PVC).
Phenolics including Phenol-Formaldehyde and Related Resins
Phenolics are polymers made from phenol (hydroxybenzene). Bakelite is a phenolic polymer made from phenol and formaldehyde (methanal). It is a thermosetting polymer with extensive cross linking between polymer strands (Hein et al, 2014). Bakelite (Figure 26.7m.) was patented on December 7, 1909. The creation of a synthetic plastic was revolutionary for its electrical nonconductivity and heat-resistant properties in electrical insulators, radio and telephone casings and such diverse products as kitchenware, jewelry, pipe stems, children’s toys, and firearms. In recent years the “retro” appeal of old Bakelite products has made them collectible. Bakelite was designated a National Historic Chemical Landmark on November 9, 1993, by the American Chemical Society in recognition of its significance as the world’s first synthetic plastic.
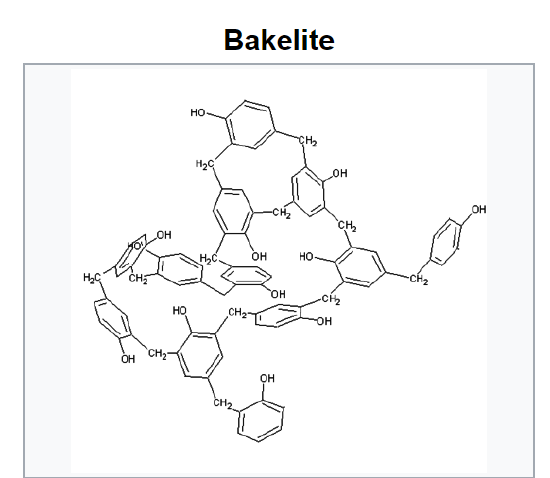
Melamine (Figure 27.6n.) is an organic compound with the formula C3H6N6. This white solid is a trimer of cyanamide, with a 1,3,5-triazine skeleton. Like cyanamide, it contains 67% nitrogen by mass, and its derivatives have fire retardant properties due to its release of nitrogen gas when burned or charred. Melamine can be combined with formaldehyde and other agents to produce melamine resins. Such resins are characteristically durable thermosetting plastic used in high pressure decorative laminates such as Formica, melamine dinnerware, laminate flooring, and dry erase boards. Melamine foam is used as insulation, soundproofing material and in polymeric cleaning products, such as Magic Eraser.
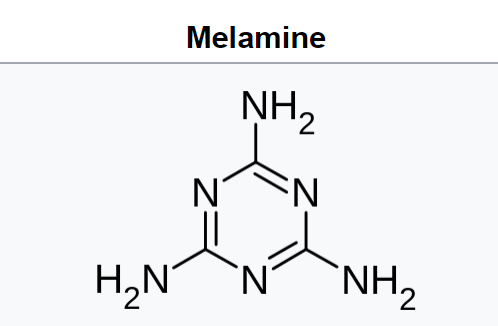
Polyurethanes
Polyurethane (PUR and PU) is a polymer composed of organic units joined by carbamate (urethane) links. While most polyurethanes are thermosetting polymers that do not melt when heated, thermoplastic polyurethanes are also available. Polyurethanes are in the class of compounds called reaction polymers, which include epoxies, unsaturated polyesters, and phenolics. Polyurethanes are produced by reacting an isocyanate containing two or more isocyanate groups per molecule (R−(N=C=O)n) with a polyol containing on average two or more hydroxyl groups per molecule (R′−(OH)n) in the presence of a catalyst or by activation with ultraviolet light (Figure 27.6o.).
Polyurethanes are used in the manufacture of high-resilience foam seating, rigid foam insulation panels, microcellular foam seals and gaskets, durable elastomeric wheels and tires (such as roller coaster, escalator, shopping cart, elevator, and skateboard wheels), automotive suspension bushings, electrical potting compounds, high performance adhesives, surface coatings and surface sealants, synthetic fibers (e.g., Spandex), carpet underlay, hard-plastic parts (e.g., for electronic instruments), condoms, and hoses.
Fully reacted polyurethane polymer is chemically inert. No exposure limits have been established in the U.S. by OSHA (Occupational Safety and Health Administration) or ACGIH (American Conference of Governmental Industrial Hygienists). It is not regulated by OSHA for carcinogenicity. However, polyurethane polymer is a combustible solid and can be ignited if exposed to an open flame. Decomposition from fire can produce significant amounts of carbon monoxide and hydrogen cyanide, in addition to nitrogen oxides, isocyanates, and other toxic products. Because of the flammability of the material, it has to be treated with flame retardants (at least in case of furniture), almost all of which are considered harmful. California later issued Technical Bulletin 117 2013 which allowed most polyurethane foam to pass flammability tests without the use of flame retardants. Green Science Policy Institute states: “Although the new standard can be met without flame retardants, it does NOT ban their use. Consumers who wish to reduce household exposure to flame retardants can look for a TB117-2013 tag on furniture and verify with retailers that products do not contain flame retardants.” Liquid resin blends and isocyanates may contain hazardous or regulated components. Isocyanates are known skin and respiratory sensitizers. Additionally, amines, glycols, and phosphate present in spray polyurethane foams present risks. Exposure to chemicals that may be emitted during or after application of polyurethane spray foam (such as isocyanates) are harmful to human health and therefore special precautions are required during and after this process.
In the United States, additional health and safety information can be found through organizations such as the Polyurethane Manufacturers Association (PMA) and the Center for the Polyurethanes Industry (CPI), as well as from polyurethane system and raw material manufacturers. Regulatory information can be found in the Code of Federal Regulations Title 21 (Food and Drugs) and Title 40 (Protection of the Environment). In Europe, health and safety information is available from ISOPA, the European Diisocyanate and Polyol Producers Association.
Polycarbonates
Polycarbonates (PC) are a group of thermoplastic polymers containing carbonate groups (−O−(C=O)−O−) in their chemical structures. The main polycarbonate material is produced by the reaction of bisphenol A (BPA) and phosgene COCl2. The overall reaction is shown in Figure 27.6p.
Polycarbonates used in engineering are strong, tough materials, and some grades are optically transparent. They are easily worked, molded, and thermoformed. Because of these properties, polycarbonates find many applications. A balance of useful features, including temperature resistance, impact resistance and optical properties, positions polycarbonates between commodity plastics and engineering plastics.
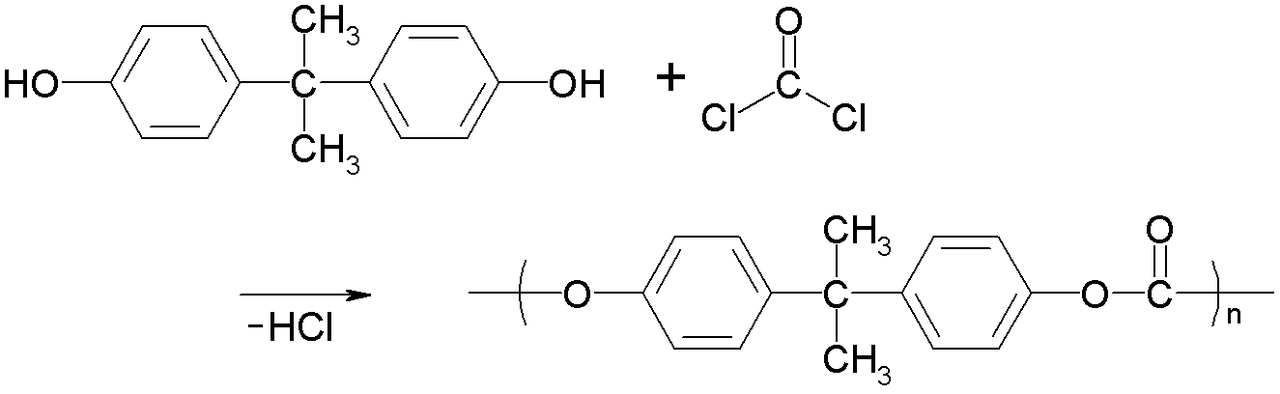
Polycarbonate is mainly used for electronic applications that capitalize on its collective safety features. Being a good electrical insulator and having heat-resistant and flame-retardant properties. The second largest consumer of polycarbonates is the construction industry, e.g., for dome lights, flat or curved glazing, and sound walls, which all use extruded flat solid or multiwall sheet, or corrugated sheet. A major application of polycarbonate is the production of Compact Discs, DVDs, and Blu-ray Discs.
Attribution & References
- “10.5: Condensation Polymers” In Map: Chemistry for Changing Times (Hill and McCreary) by Marisa Alviar-Agnew (Sacramento City College) & LibreTexts, licensed under CC BY-NC-SA 4.0. Attributions from original source:
- William Reusch, Professor Emeritus (Michigan State U.), Virtual Textbook of Organic Chemistry
- Stephen Lower, Professor Emeritus (Simon Fraser U.) Chem1 Virtual Textbook
- Marisa Alviar-Agnew (Sacramento City College)
- Jim Clark (Chemguide.co.uk)
- Wikipedia
- “18.4 Amines and Amides” In General Chemistry 1 & 2 by Rice University, a derivative of Chemistry (Open Stax) by Paul Flowers, Klaus Theopold, Richard Langley & William R. Robinson and is licensed under CC BY 4.0. Access for free at Chemistry (OpenStax)
References cited in text
Hein, M., Pattison, S., Arena, S., & Best, L. R. (2014). Introduction to General, Organic, and Biochemistry (11th ed.). Wiley.
Nagitarvik, R. & Rayner-Canham, G. (2022, Fall). Composites in Inuit life: What was old is new again. Chem 13 News Magazine.
two different monomers combine with the loss of a small molecule, usually water
arise from the reaction of carboxylic acid and an alcohol.
commonly abbreviated PET, PETE, or Dacron
polymers made from phenol (hydroxybenzene)
polymer composed of organic units joined by carbamate (urethane) links
group of thermoplastic polymers containing carbonate groups in their chemical structures