24.4 Chemical Properties of Aldehydes and Ketones
Learning Objectives
By the end of this section, you will be able to:
- Explain the formation of aldehydes and ketones.
- Describe the typical reactions that take place with aldehydes and ketones.
Organic functional groups can be converted into other functional groups through reactions. A map of some of the more common reactions to convert functional groups can be found in Section 19.6 – General Reactions of Carbon in Infographic 19.6a.
Preparation of Aldehydes and Ketones
Aldehydes are commonly prepared by the oxidation of alcohols whose –OH functional group is located on the carbon atom at the end of the chain of carbon atoms in the alcohol, as shown in Figure 24.4a.

Alcohols that have their –OH groups in the middle of the chain are necessary to synthesize a ketone, which requires the carbonyl group to be bonded to two other carbon atoms, as shown in Figure 24.4b.

An alcohol with its –OH group bonded to a carbon atom that is bonded to no or one other carbon atom will form an aldehyde. An alcohol with its –OH group attached to two other carbon atoms will form a ketone. If three carbons are attached to the carbon bonded to the –OH, the molecule will not have a C–H bond to be replaced, so it will not be susceptible to oxidation.
The oxidation of alcohols to aldehydes and ketones was previously discussed in Section 23.4 Reactions of Alcohols and displayed in Infographic 23.4a.
Oxidation of Aldehydes and Ketones
Aldehydes and ketones are much alike in many of their reactions, owing to the presence of the carbonyl functional group in both. They differ greatly, however, in one most important type of reaction: oxidation. Aldehydes are readily oxidized to carboxylic acids, whereas ketones resist oxidation, as shown in Figure 24.4c.
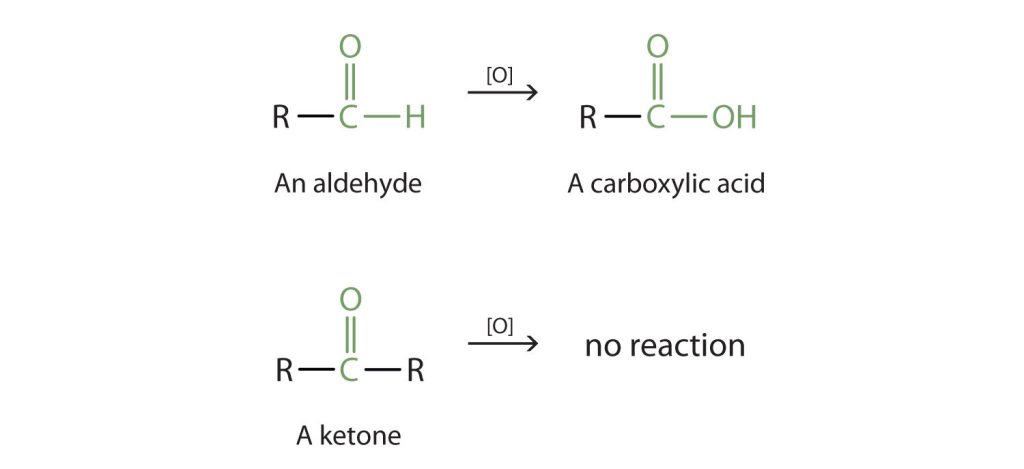
The aldehydes are, in fact, among the most easily oxidized of organic compounds. They can easily be oxidized by oxygen (O2) in air to carboxylic acids:
\[2RCHO + O_2 \rightarrow 2RCOOH \label{14.10.1} \]
The ease of oxidation helps chemists identify aldehydes. A sufficiently mild oxidizing agent can distinguish aldehydes not only from ketones but also from alcohols. Tollens’ reagent, for example, is an alkaline solution of silver (Ag+) ion complexed with ammonia (NH3), which keeps the Ag+ ion in solution.
\[H_3N—Ag^+—NH_3 \label{14.10.2} \]
When Tollens’ reagent oxidizes an aldehyde, the Ag+ ion is reduced to free silver (Ag), as shown in Figure 24.4d.
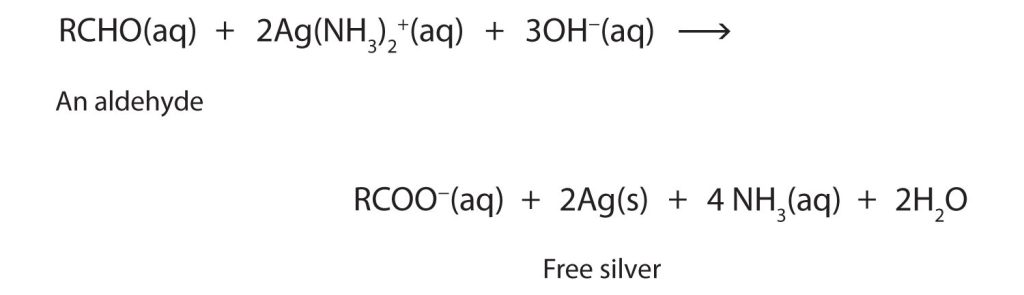
Deposited on a clean glass surface, the silver produces a mirror, as shown in Figure 24.4e. Ordinary ketones do not react with Tollens’ reagent.
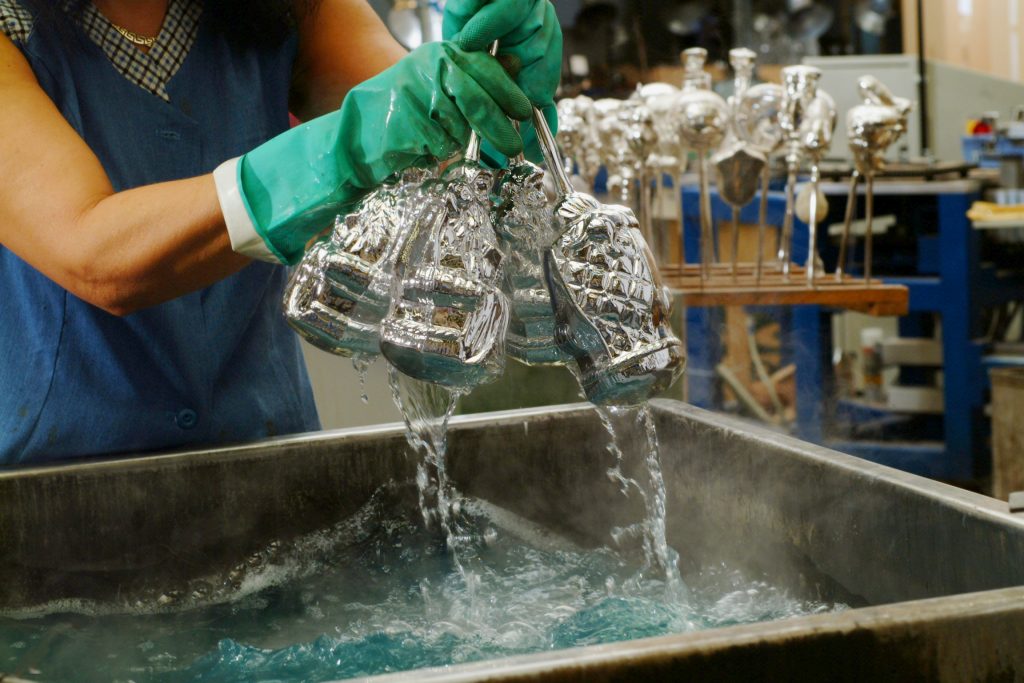
Spotlight on Everyday Chemistry: Silver Mirrors
The Tollens’ reaction is used to identify the presence of an aldehyde and also used in the production of mirrors. Infographic 24.4a. shows the details.
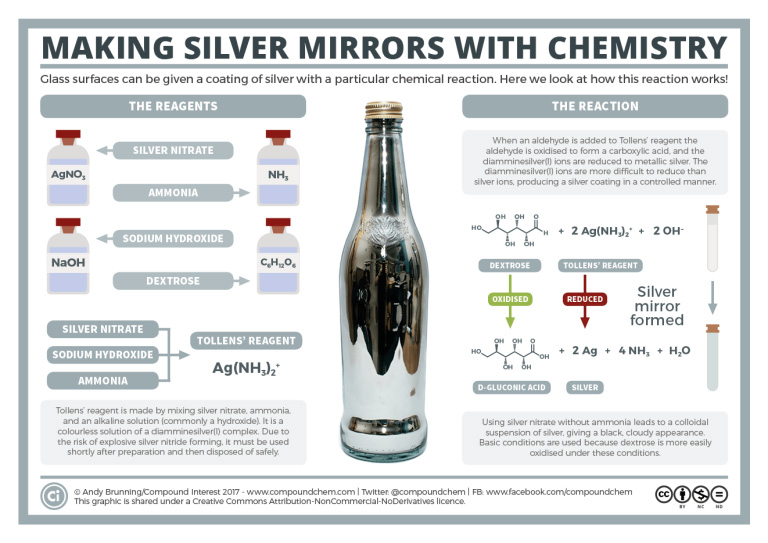
Other Oxidation Tests
The oxidation of aldehydes can be confirmed using the Tollens’ reagent (explained above) which produces a silver mirror finish on the reaction vessel. Other tests that confirm the oxidation of an aldehyde use the Benedict’s reagent or the Fehling’s test. Both of these require the presence of a copper ion in solution that changes colour when an aldehyde is present.
With the Benedict’s reagent, complexed copper (II) ions are reduced to copper (I) ions that form a brick-red precipitate (copper (I) oxide) (Figure 24.4f. and Figure 24.4g.). The Fehling’s test contains copper (II) ions complexed with tartrate ions and results in the same changes as with the Benedict’s reagent.
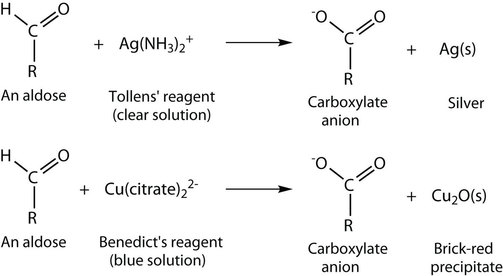
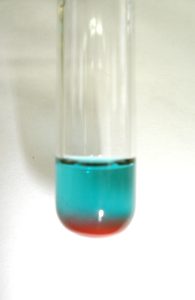
Although ketones resist oxidation by ordinary laboratory oxidizing agents, they undergo other chemical reactions such as reduction, addition, and combustion, as do aldehydes.
Reduction of Aldehydes and Ketones
The most general method for preparing alcohols, both in the laboratory and in living organisms, is by reduction of a carbonyl compound. Just as reduction of an alkene adds hydrogen to a carbon-carbon double bond to produce an alkene, the reduction of an aldehyde or ketone add hydrogen to the carbon-oxygen double bond to give an alcohol. All kinds of carbonyl compounds can be reduced, including aldehydes, ketones, carboxylic acids, and esters.
![Reduction of a carbonyl group (where the oxygen is depicted in a pink colour) to an alcohol (the OH group is depicted in a pink colour). The reducing agent used is [H]](https://ecampusontario.pressbooks.pub/app/uploads/sites/3009/2023/12/reduction.jpg)
Aldehydes are easily reduced to give primary alcohols, and ketones are reduced to give secondary alcohols.

Dozens of reagents are used in the laboratory to reduce aldehydes and ketones, depending on the circumstances, but sodium borohydride, NaBH4, is usually chosen because of its safety and ease of handling (Figure 24.4j.). Lithium aluminum hydride, LiAlH4, is another reducing agent often used but is much more reactive and much more dangerous than NaBH4 (Figure 24.4k.).
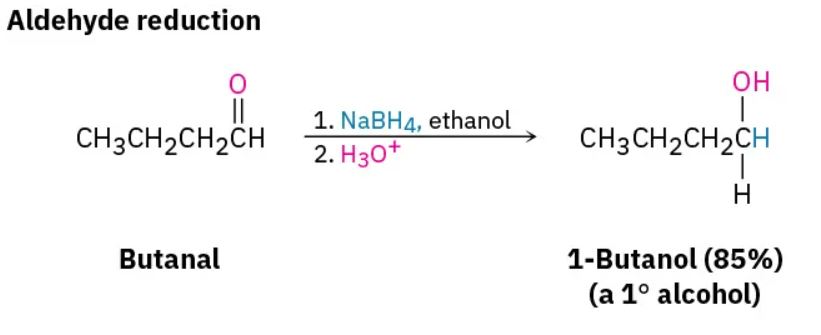
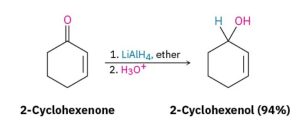
Example 24.4a
What carbonyl compounds would you reduce to obtain the following alcohols?
Solution:
Identify the target alcohol as primary, secondary, or tertiary. A primary alcohol can be prepared by reduction of an aldehyde, an ester, or a carboxylic acid; a secondary alcohol can be prepared by reduction of a ketone; and a tertiary alcohol can’t be prepared by reduction.
(a) The target molecule is a secondary alcohol, which can be prepared only by reduction of a ketone. Either NaBH4 or LiAlH4 can be used.
(b) The target molecule is a primary alcohol, which can be prepared by reduction of an aldehyde, an ester, or a carboxylic acid. LiAlH4 is needed for the ester and carboxylic acid reductions.
Source: Organic Chemistry (OpenStax), CC BY-NC-SA 4.0.
Exercise 24.4a
Draw the carbonyl compound that when reduced will form the following alcohols.
Check Your Answers:[1]
Source: Exercise 24.4a is adapted by Samantha Sullivan Sauer from Organic Chemistry (OpenStax) on Libre Texts, images created using Biovia Draw, CC BY-NC-SA.
Oxidation and reduction are paired reactions in that one reverses the results of the other. Examining the oxidation states of carbon as it gets oxidized helps to understand the changes. In Figure 24.4l., methane is oxidized step-by-step to methanol, then methanal, then methanoic acid, then carbon dioxide. Carbon dioxide cannot be further oxidized. In Figure 24.4m., the functional groups of carbon are ranked based on the oxidation state of carbon with alkanes being the most reduced and carboxylic acid derivatives being the most oxidized.
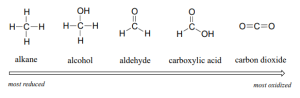
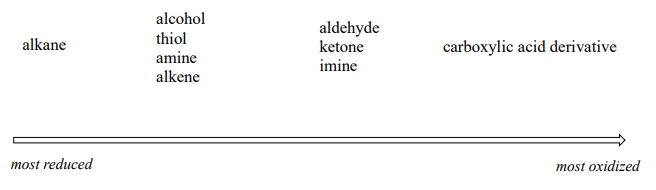
Example 24.4b
Methane represents the completely reduced form of an organic molecule that contains one carbon atom. Sequentially replacing each of the carbon-hydrogen bonds with a carbon-oxygen bond would lead to an alcohol, then an aldehyde, then a carboxylic acid, and, finally, carbon dioxide:
What are the oxidation numbers for the carbon atoms in the molecules shown here?
Solution:
In this example, we can calculate the oxidation number for the carbon atom in each case (note how this would become difficult for larger molecules with additional carbon atoms and hydrogen atoms, which is why organic chemists use the definition dealing with replacing C–H bonds with C–O bonds).
For CH4, the carbon atom carries a –4 oxidation number (the hydrogen atoms are assigned oxidation numbers of +1 and the carbon atom balances that by having an oxidation number of –4).
For the alcohol (in this case, methanol), the carbon atom has an oxidation number of –2 (the oxygen atom is assigned –2, the four hydrogen atoms each are assigned +1, and the carbon atom balances the sum by having an oxidation number of –2; note that compared to the carbon atom in CH4, this carbon atom has lost two electrons so it was oxidized).
For the aldehyde, the carbon atom’s oxidation number is 0 (–2 for the oxygen atom and +1 for each hydrogen atom already balances to 0, so the oxidation number for the carbon atom is 0).
For the carboxylic acid, the carbon atom’s oxidation number is +2 (two oxygen atoms each at –2 and two hydrogen atoms at +1).
For carbon dioxide, the carbon atom’s oxidation number is +4 (here, the carbon atom needs to balance the –4 sum from the two oxygen atoms).
Exercise 24.4b
Indicate whether the marked carbon atoms in the three molecules below are oxidized or reduced relative to the marked carbon atom in ethanol:
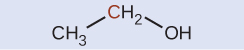
There is no need to calculate oxidation states in this case; instead, just compare the types of atoms bonded to the marked carbon atoms:
Check Your Answers: [2]
Exercise and image source: Chemistry (OpenStax), CC BY 4.0
Addition of Aldehydes and Ketones
Addition of Alcohol
One of the most important examples of an addition reaction in biochemistry is the addition of an alcohol to a ketone or aldehyde. When an alcohol adds to an aldehyde, the result is called a hemiacetal; when an alcohol adds to a ketone the resulting product is a hemiketal (Figure 24.4n). The prefix ‘hemi’ (half) is used in each term because addition of a second alcohol can occur resulting in species called acetals and ketals. The conversion of an alcohol and aldehyde (or ketone) to a hemiacetal (or hemiketal) is a reversible process.
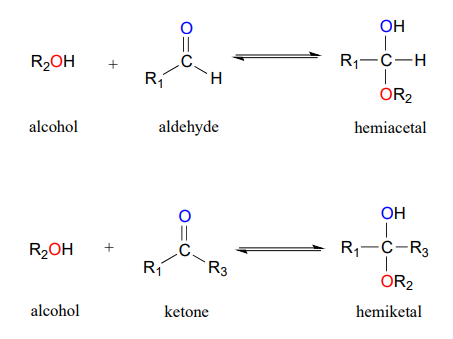
The generalized mechanism for the process is shown in Figure 24.4o. Focus on the connection made between the aldehyde and the alcohol.
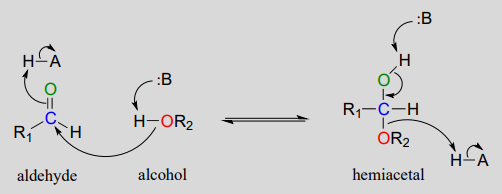
Addition of Water
Aldehydes and ketones, when in aqueous solution, exist in equilibrium with their hydrate form. A hydrate forms as the result of a water molecule adding to the carbonyl carbon of the aldehyde or ketone (Figure 24.4p.). Although you should be aware that aldehyde and ketone groups may exist to a considerable extent in their hydrated forms when in aqueous solution (depending upon their structure), they are usually drawn in their non-hydrated form for the sake of simplicity.
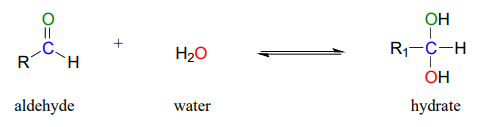
Addition of HCN
Hydrogen cyanide (HCN) adds across the carbon-oxygen double bond in aldehydes and ketones to produce compounds known as hydroxynitriles or cyanohydrins. For example, with ethanal (an aldehyde) you get 2-hydroxypropanenitrile (Figure 24.4q.). With propanone (a ketone) you get 2-hydroxy-2-methylpropanenitrile (Figure 24.4r.).
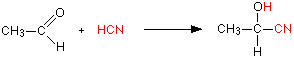
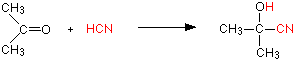
These are examples of nucleophilic addition. The carbon-oxygen double bond is highly polar, and the slightly positive carbon atom is attacked by the cyanide ion acting as a nucleophile (Figure 24.4s.).

This is considered to be a reversible addition. Remember that HCN or hydrogen cyanide contains a carbon-nitrogen triple bond (HC≡N). This triple bond is open itself to addition or reduction reactions.
RCH=O + H–C≡N RCH(OH)CN (a cyanohydrin)
Spotlight on Everyday Chemistry: Millipede Defense
The cyanohydrin from benzaldehyde is named mandelonitrile (Figure 24.4t.).
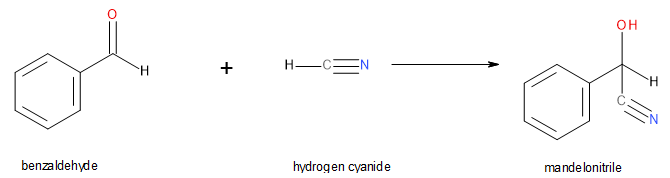
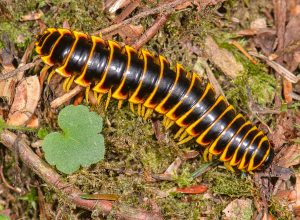
The reversibility of cyanohydrin formation is put to use by the millipede Apheloria corrugata (Figure 24.4u.) in a remarkable defense mechanism. This arthropod releases mandelonitrile from an inner storage gland into an outer chamber, where it is enzymatically broken down into benzaldehyde and hydrogen cyanide before being sprayed at an enemy.
Watch Aldehyde and Ketone Reactions – Hydrates, Acetals, & Imines: Crash Course Organic Chemistry #29 – YouTube (13 min). Note not all parts of the video are relevant to this text.
Video Source: Crash Course. (2021, June 9). Aldehyde and Ketone Reactions – Hydrates, Acetals, & Imines: Crash Course Organic Chemistry #29 – YouTube [Video]. YouTube.
Attribution & References
Except where otherwise noted, this page is adapted by Gregory A. Anderson and Samantha Sullivan Sauer from
- “15.3: Properties of Aldehydes and Ketones” In Map: Fundamentals of General Organic and Biological Chemistry (McMurry et al.), CC BY-NC-SA 3.0, a remixed version of Basics of GOB (Ball et al.), CC BY-NC-SA 4.0 which is a LibreTexts version of Introduction to Chemistry: GOB (v. 1.0), CC BY-NC-SA 3.0
- “14.10: Properties of Aldehydes and Ketones” In Basics of General, Organic, and Biological Chemistry (Ball et al.)by David W. Ball, John W. Hill, and Rhonda J. Scott via LibreTexts, CC BY-NC-SA 4.0./ A LibreTexts version of Introduction to Chemistry: GOB (v. 1.0), CC BY-NC 3.0.
- 17.5: Alcohols from Carbonyl Compounds- Reduction In Organic Chemistry (OpenStax via LibreTexts) by John McMurray, a LibreTexts version of Organic Chemistry (OpenStax). Access for free at Organic Chemistry (OpenStax).
- “10.3 Hemiacetals, Hemiketals, and Hydrates” In Organic Chemistry with a Biological Emphasis (Soderberg) by Tim Soderberg, CC BY-NC-SA 4.0. / A derivative of Organic Chemistry with a Biological Emphasis Volume I, CC BY-NC-SA 4.0.
- “11.2.5 Addition of HCN to Aldehydes and Ketones” and “16.2: Oxidation of Aldehydes” In Purdue: Chem 26605: Organic Chemistry II (Lipton) is shared under a CC BY-NC-SA 4.0 license and was authored, remixed, and/or curated by LibreTexts. / A derivative of The Addition of Hydrogen Cyanide to Aldehydes and Ketones and “Oxidation of Aldehydes and Ketones” In Addition Reactions by Jim Clark, CC BY-NC 4.0.
- “Reversible Addition Reactions of Aldehydes and Ketones” by William Reusch In Supplemental Modules (Organic Chemistry) , CC BY-NC-SA 4.0
- “17.5: Alcohols from Carbonyl Compounds – Reduction” In Organic Chemistry (OpenStax via LibreTexts) by John McMurray, a LibreTexts version of Organic Chemistry (OpenStax). Access for free at Organic Chemistry (OpenStax).
- “7.6: Properties of Monosaccharides” In CHEM100 Victor Valley College by LibreTexts, CC BY-NC-SA 4.0 . / A derivative of “16.5: Properties of Monosaccharides In Basics of GOB Chemistry (Ball et al.), a derivative of Introduction to Chemistry: GOB (v. 1.0), CC BY-NC 3.0
- “15.2: Oxidation and Reduction of Organic Compounds – An Overview” by Organic Chemistry with a Biological Emphasis (Soderberg) by Tim Soderberg, CC BY-NC-SA 4.0. / A derivative of Organic Chemistry with a Biological Emphasis Volume I, CC BY-NC-SA 4.0.
- “20.3 Aldehydes, Ketones, Carboxylic Acids, and Esters” In Chemistry (OpenStax), CC BY 4.0, access for free at Chemistry (OpenStax)
-
Only one potential answer is shown for each question. There may be other possible answers.
↵
-
- reduced (bond to oxygen atom replaced by bond to hydrogen atom);
- oxidized (one bond to hydrogen atom replaced by one bond to oxygen atom);
- oxidized (2 bonds to hydrogen atoms have been replaced by bonds to an oxygen atom)
↵