8. Microbial Catabolism
8.6 Lithotrophy
Learning Objectives
- Explain the general process of chemolithotrophy and describe how it differs from chemoheterotrophy
- Identify the most common electron donors and acceptors for chemolithotrophs
- Explain the process of reverse electron flow and how/why is it used by some chemolithoautotrophs
Chemolithotrophy is the oxidation of inorganic chemicals for the generation of energy and is another form of cellular respiration. These organisms are exclusively bacteria and archaea. The electron sources for their electron transport system are inorganic and, as with the heterotrophs, ATP is generated by oxidative phosphorylation. Although lithotrophs may perform aerobic or anaerobic respiration, because of energy constraints, they are typically aerobic.
Inorganic Energy Sources
Chemolithotrophs use a variety of inorganic compounds as electron donors, with the most common substances being hydrogen gas, sulphur compounds (such as sulphide and sulphur), nitrogen compounds (such as ammonium and nitrite), and ferrous iron.
Hydrogen oxidizers
These organisms oxidize hydrogen gas (H2) with the use of a hydrogenase enzyme. Both aerobic and anaerobic hydrogen oxidizers exist, with the aerobic organisms eventually reducing oxygen to water. The sulphate-reducing bacterium Desulfovibrio is an example of a hydrogen oxidizer; these organisms can also use organics as their energy source. Because the very negative standard reduction potential of hydrogen gas, the of anaerobic respiration using sulphate as a terminal electron acceptor is still substantial. This is in contrast to the iron and nitrogen oxidizers (Figure 8.27).
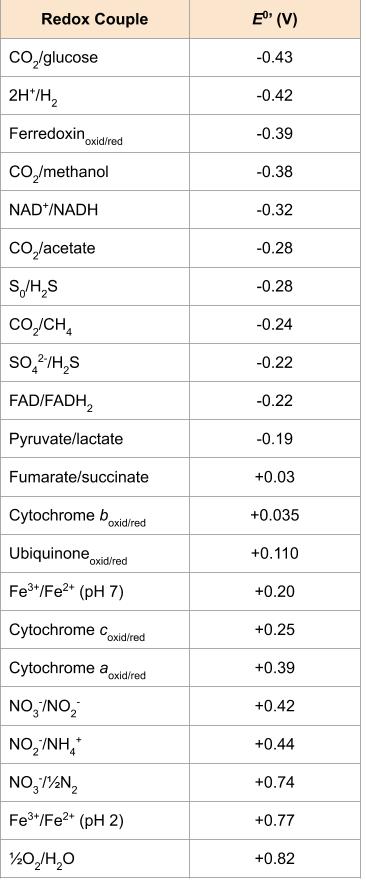
Sulphur oxidizers
As a group, these organisms are capable of oxidizing a wide variety of reduced and partially reduced sulphur compounds such as hydrogen sulphide (H2S), elemental sulphur (S0), thiosulphate (S2O32-), and sulphite (SO32-). Sulphate (SO42-) is frequently a by-product of the oxidation. Often the oxidation occurs in a stepwise fashion with the help of the sulphite oxidase enzyme. Bacteria and archaea associated with acid mine drainage (AMD) typically oxidize reduced sulphur compounds producing sulphuric acid. The resulting acidic pH causes oxidized minerals to precipitate, exacerbating the environmental impact of their activities (Figure 8.28).
Iron oxidizers
These organisms oxidize ferrous iron (Fe2+) to ferric iron (Fe3+). As shown in the Figure 2, Fe2+ has such a positive standard reduction potential, the bioenergetics are not extremely favourable, even using oxygen as a final electron acceptor. Acidithiobacillus ferrooxidans, an AMD bacterium, oxidizes both atoms of the solid mineral FeS2 (also known as iron pyrite or “fool’s gold).
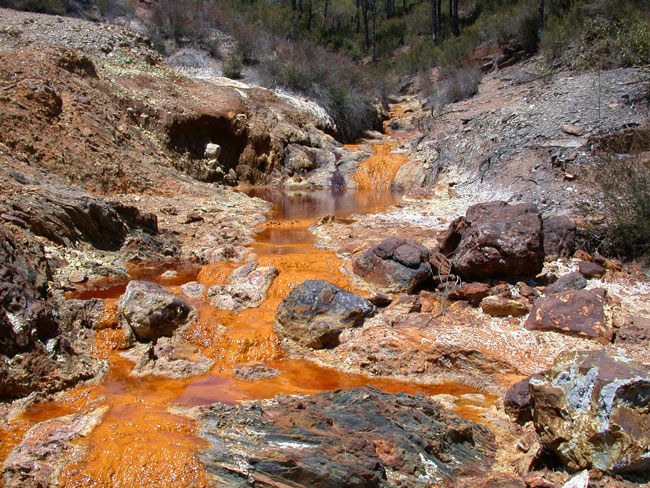
Nitrogen oxidizers: nitrifiers & comammox bacteria
The aerobic oxidation of ammonia (NH3) is generally performed by nitrifying bacteria and archaea in a two-step process, where one group oxidizes ammonia to nitrite (NO2–) and the second group oxidizes the nitrite to nitrate (NO3–). The best characterized nitrifiers are bacterial species in the genera Nitrosomonas (aerobic ammonia oxidizers) and Nitrobacter (aerobic nitrite oxidizers). Nitrifiers are typically often found living together in soil or in water systems, and their combined processes release nitrogen gas (N2) back to the atmosphere (Figure 8.27).
Quite recently, some bacterial species in the genus Nitrospira have been found to oxidize ammonia all the way to N2. This is an aerobic process. Because they are capable of the complete oxidation of ammonia, these bacteria are called comammox bacteria (complete ammonia oxidation). Sequence data indicates that these organisms acquired the ability to oxidize ammonia to nitrate by a relatively recent horizontal gene transfer event.
Anammox
Anammox or anaerobic ammonia oxidation is a relatively recently discovered process performed by anaerobic lithotrophic bacteria. These organisms utilize nitrogen compounds as both electron acceptor and electron donor. Ammonia (NH3) is oxidized anaerobically using nitrite (NO2–), in a somewhat complex electron transport process that yields nitrogen gas (N2) as the oxidized end-product. The reactions occur within the anammoxasome, a specialized internal membrane-bound structure which constitutes 50-70% of the total cell volume. Anammox bacteria are found in the phylum Planctomycetes and the freshwater species are of interest for their application in sewage treatment. In the marine environment, microbial ecologists and oceanographers are concerned about implications of growing hypoxic zones and a potential loss of fixed nitrogen through increased anammox.
- For the redox couple Fe2+/Fe3+, identify which would be an energy donor, which would be an electron acceptor for anaerobic respiration, and explain how you identify the donor vs the acceptor.
- Distinguish between the nitrifiers and comammox bacteria
Lithotrophic Electron Transport System
Electrons enter the lithotrophic ETS by direct oxidation of an inorganic energy source. In the case of insoluble inorganic minerals, components on the cell surface and/or associated with the outer membrane and periplasmic space transfer electrons from the extracellular mineral to the ETS in the plasma membrane, as shown in Figure 8.29.
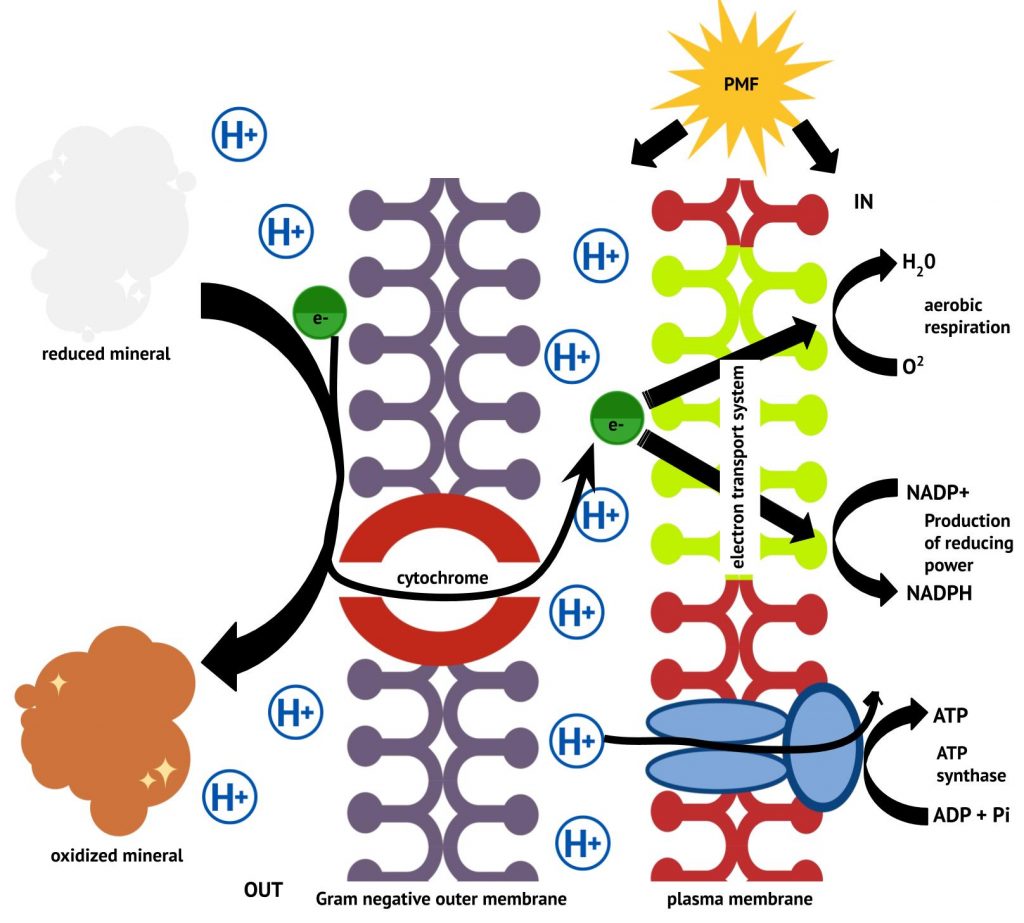
Electron acceptors
Lithotrophy can occur aerobically or anaerobically, however, based on its strongly positive redox potential (i.e. its position at the very bottom of the redox tower), the best electron acceptor is oxygen. This creates the biggest distance between the electron donor and the electron acceptor, and given that most inorganic energy sources are not particularly good energy sources (Figure 8.27), lithotrophs are very often aerobic. Using a non-oxygen acceptor allows chemolithotrophs to have greater diversity and the ability to live in a wider variety of environments, although they sacrifice energy production.
Chemolithoautotrophs vs chemolithoheterotrophs
Most chemolithotrophs are autotrophs (chemolithoautotrophs), where they fix atmospheric carbon dioxide to assemble the organic compounds that they need. These organisms require both ATP and reducing power (i.e. NADH/NADPH) in order to ultimately convert the oxidized molecule CO2 into a greatly reduced organic compound, like glucose. If a chemolithoautotroph is using an electron donor with a higher redox potential than NAD+/NADP, they must use reverse electron flow to push electrons back up the electron tower. This is energetically unfavourable to the cell, consuming energy from the proton motive force to drive electrons in a reverse direction back through the ETC.
Some microbes are chemolithoheterotrophs, using an inorganic chemical for their energy and electron needs, but relying on organic chemicals in the environment for their carbon needs. These organisms are also called mixotrophs, since they require both inorganic and chemical compounds for their growth and reproduction. in the case of Desulfovibrio, as described above, these sulphate-reducing bacteria can grow heterotrophically, or they can use hydrogen as an energy source. In either case, their carbon source is organic.
- Explain why most lithotrophs are aerobic.
- Define the term “reverse electron transport”.
- Describe some of the shared features, and differences between, electron transport systems of lithotrophs and those of heterotrophs.
Key Takeaways
- Lithotrophs oxidize inorganics for energy and reducing power and are usually lithoautotrophs, obtaining carbon by fixing CO2
- Mixotrophs are lithoheterotrophs: their energy and reducing power come from inorganics but their carbon comes from organics
- Lithotrophy is a form of respiration, with ATP arising by oxidative phosphorylation
- The most common electron donors are H2, Fe2+, H2S, NH3, NO2–
- ETS components on the cell surface/in the Gram negative outer membrane extract electrons from extracellular minerals and pass them to the ETS in the plasma membrane
- Because of the relatively positive standard reduction potential (
) of their energy sources, lithotrophs are often aerobic
- Because of the relatively positive standard reduction potential (
) of their energy sources, lithotrophs generally must use ATP and reverse electron flow through their ETS to synthesize NAD(P)H from NAD(P)+ + H+
- ATP yields from lithotrophy are much reduced compared to heterotrophs
- Bacteria in the phylum Planctomycetes are anammox bacteria; they oxidize NH3 anaerobically in an anammoxosome, releasing N2
Multiple Choice
Short Answer
- Why are the sulphur-oxidizing lithotrophs typically acidophiles?
- What is the major difference between a nitrifying bacterium and a comammox bacterium?
Critical Thinking
- Explain why an organism like Desulfovibrio could grow as an anaerobic lithotroph, while Acidithiobacillus ferrooxidans growing on FeSO4 would be an obligate aerobe.
- Explain why most lithotrophs, with the exception of those that oxidize H2, must expend energy to make the reduced cofactors for biosynthesis.
Media Attributions
- Detailed redox tower
- Acidophilic archaea
- Lithotrophic oxidation of insoluble materials – Corrected