10. Microbial Ecology and Applied Microbiology
10.5 Water Pollution and Bioremediation
Learning Objectives
- Explain the relationship between the microbes and water quality and the relevance of biological oxygen demand to water quality
- Describe how the microbes are used in remediating heavy metal pollution
- Describe how microbes are used for sewage treatment in urban wastewater treatment plants and rural septic tanks
- Explain the basis for bioremediation following the Exxon Valdez spill and the reason a similar strategy would not work for the DeepWater Horizon spill
Microbial bioremediation is the use of prokaryotes (or microbial metabolism) to remove pollutants. The oldest example, and the most successful, is sewage treatment, which has been used in one form or another, for thousands of years. However, the connection between disease and contaminated water was only made in the late 1800s. Whether the sewage is buried below an outhouse, in septic tanks or processed in modern wastewater treatment plants, bioremediation of the water is largely, if not entirely, based on microbial degradation of the organics to inorganics. Bioremediation happens naturally in the environment, but has also been used to remove agricultural chemicals (e.g., pesticides, fertilizers) that leach from soil into groundwater and the subsurface. Certain toxic metals and oxides, such as selenium and arsenic compounds, can also be removed from water by bioremediation. The reduction of SeO4-2 to SeO3-2 and to Se0 (metallic selenium) removes selenium ions from water and is catalyzed by bacteria and archaea using the selenium ions are terminal electron acceptors in anaerobic respiration (see Cellular Respiration). As an active ingredient of some pesticides, mercury is used in industry, and is also a by-product of certain processes such as battery production. Methyl mercury is usually present in very low concentrations in natural environments, but it is highly toxic because it accumulates in living tissues. Several species of bacteria can carry out the biotransformation of toxic mercury into nontoxic forms. Bacteria such as Pseudomonas aeruginosa can convert Hg+2 into the less toxic Hg0 through anaerobic respiration.
The Impact of Human Activities on Water Quality
The most deadly form of water pollution, pathogenic microorganisms that cause waterborne diseases, kills almost 2 million people in underdeveloped countries every year. The best strategy for addressing this problem is proper sewage (wastewater) treatment. Untreated sewage is not only a major source of disease, but also a major source of other pollutants, including organics, respiration of which results in increased oxygen consumption, plant nutrients (N and P), and toxic heavy metals.
Types of Water Pollutants
Oxygen-demanding waste is an extremely important pollutant to ecosystems. Any healthy body of water has a gradient of dissolved oxygen which decreases with increasing depth. This “oxycline” is the result of exposure to the atmosphere, and, in the upper euphotic zone, the presence of the oxygenic phototrophs (cyanobacteria and algae) that split water, releasing oxygen as a photosynthetic by-product (see Microbial Phototrophy). The dissolved oxygen is needed by aquatic organisms for cellular respiration. Aerobic heterotrophic bacteria and archaea decompose dead organic matter to CO2, reducing oxygen (O2), the terminal electron acceptor, to water (H2O) (see Cellular Respiration). Too much decaying organic matter in water therefore removes oxygen from water, and from the aquatic and marine animals. The amount of oxygen used by the aerobic prokaryotes is called biological oxygen demand (BOD). The major source of dead organic matter in most natural waters is sewage; grass and leaves are smaller sources. An unpolluted body of water, with respect to oxygen, is a turbulent river that flows through a natural forest. Turbulence continually brings water in contact with the atmosphere where the O2 content is restored. The dissolved oxygen content in such a river ranges from 10 to 14 ppm, BOD is low, and clean-water fish, e.g., bass, trout, and perch dominate. A polluted body water, with respect to oxygen, is a stagnant deep lake in an urban setting with a combined sewer system. This system favours a high input of dead organic carbon from sewage overflows and limited chance for water circulation and contact with the atmosphere. In such a lake, the dissolved O2 content is ≤5 ppm O2, BOD is high, and low O2-tolerant fish, e.g., carp and catfish dominate.
Eutrophication and Algal Blooms
Nitrogen (N) and phosphorous (P) sources are pollutants closely related to oxygen-demanding waste. These are major requirements for cellular growth (see Nutrient Requirements), and are normally present at low levels in unpolluted waters. They are therefore limiting nutrients for primary producers (aquatic plants, and photosynthetic microbes). In fact, they are typically limiting nutrients in soil as well, which explains why N- and P-containing nutrients are major ingredients in most fertilizers. High concentrations of N and P from human sources (mostly agricultural and urban runoff including fertilizer, sewage, and P-based detergent) can cause eutrophication, leading to a bloom, or explosive growth, of algae and cyanobacteria. Thick mats of floating and rooted green or sometimes red algae, as depicted in Figure 10.23, create water pollution, damage the ecosystem by clogging fish gills and blocking sunlight, and damage lake aesthetics by making recreation difficult and creating an eyesore. An estimated 50% of lakes in North America, Europe, and Asia are negatively impacted by eutrophication. These blooms are often large enough to be seen by satellite (see Section 10.6 fig. 10.31)
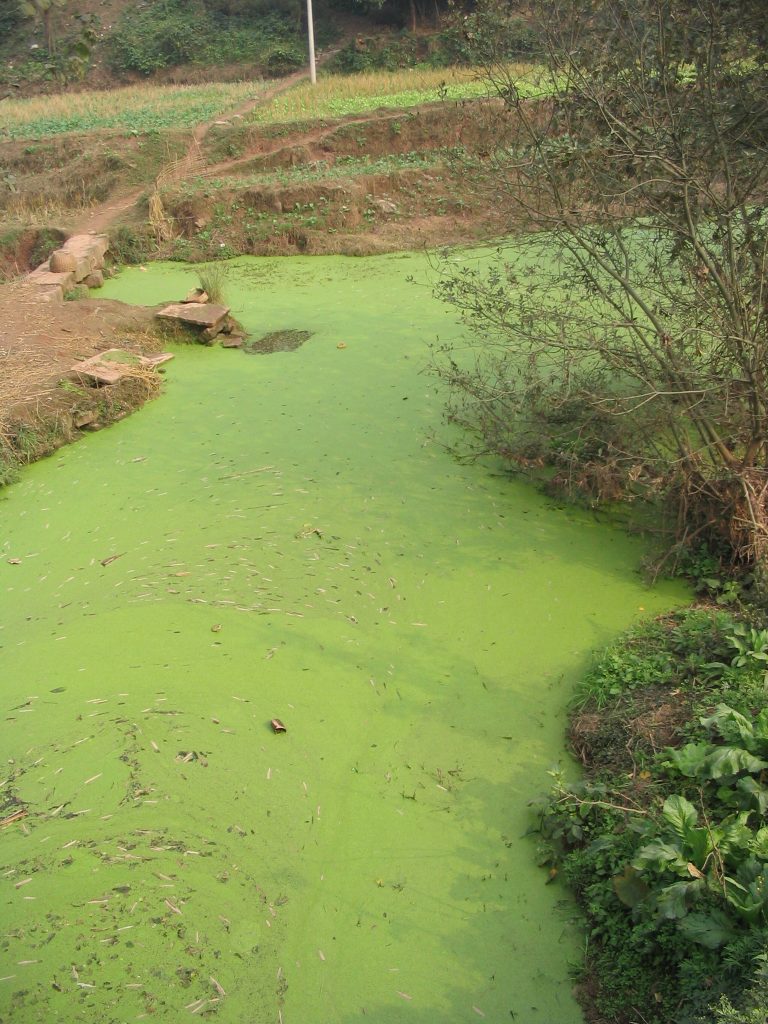
Some species of cyanobacteria and algae (in particular species of dinoflagellates) produce toxins that can kill fish, mammals, and birds, and may cause human illness. In the marine environment, certain dinoflagellates cause harmful algal blooms, referred to as “red tides”, which may be large enough to be seen by satellite (Figure 10.24). These algae secrete potent toxins that can kill fish and other organisms and also accumulate in shellfish. When humans consume shellfish from these waters, they can develop paralytic shellfish poisoning. This is not an infection but an intoxication due to the ingestion of the dinoflagellate toxin, which is not inactivated by cooking. There is no treatment, and severe cases can be fatal. Shellfish beds must be regularly monitored for the presence of the toxins, and harvests are often shut down when it is present, incurring economic costs to the fishery. Cyanobacteria may also form blooms in marine and freshwater ecosystems (see Section 10.6 fig. 10.31), and some species also produce toxins. These toxins (called microcystins) can cause allergic reactions and liver damage when ingested in drinking water or during swimming.
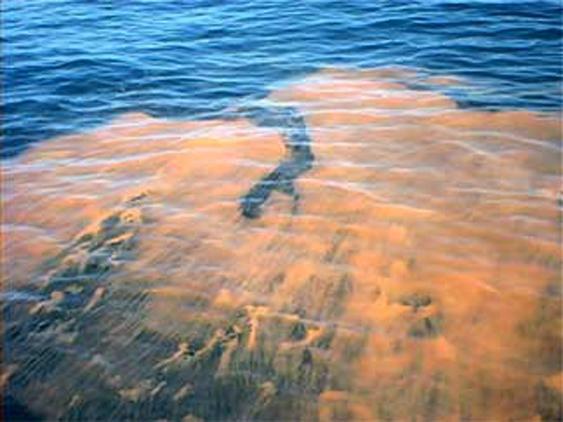
When an algal bloom dies, it becomes oxygen-demanding waste, which can create a hypoxic or dead zone. The size and number of marine hypoxic zones have grown dramatically over the past 50 years (Figure 10.25); this includes a very large dead zone in the Gulf of Mexico – the site of the Deepwater Horizon oil spill. Large scale eutrophication and hypoxia are difficult to combat as the pollution sources are varied and widespread.
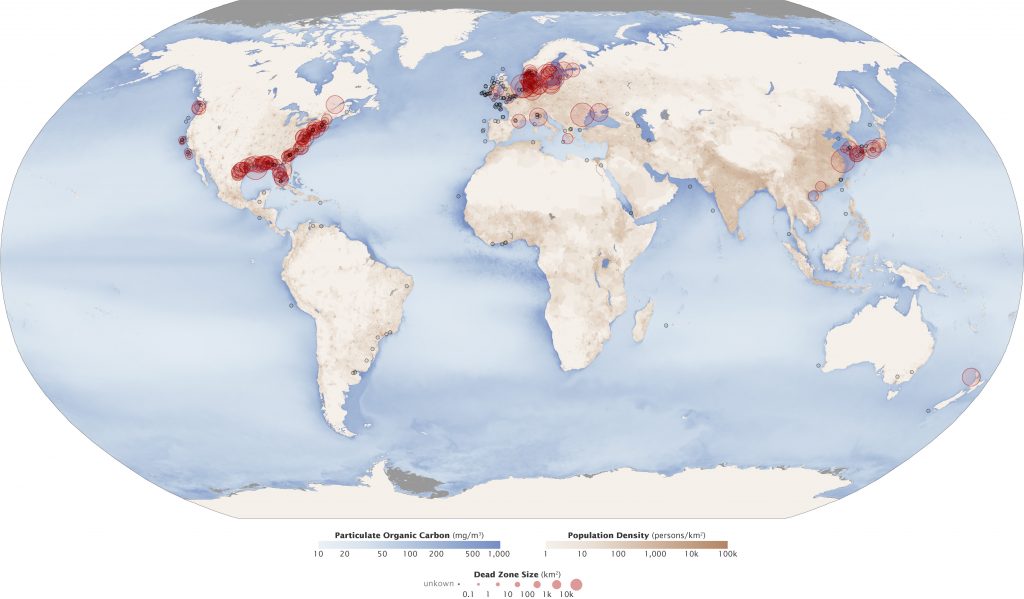
Oil Spills
Oil spills are another kind of organic pollution. These are often the result of supertanker accidents, such as when the Exxon Valdez struck a reef in Prince William Sound, Alaska in 1989. The result was the release of 10 million gallons of oil into the rich ecosystem of offshore south Alaska, leading to massive numbers of animal deaths. The largest marine oil spill was the Deepwater Horizon disaster in 2010, which began with a natural gas explosion at an oil well 65 km offshore of Louisiana (Figure 10.26). It took 3 months to cap the well and resulted in the release of an estimated 200 million gallons of oil.
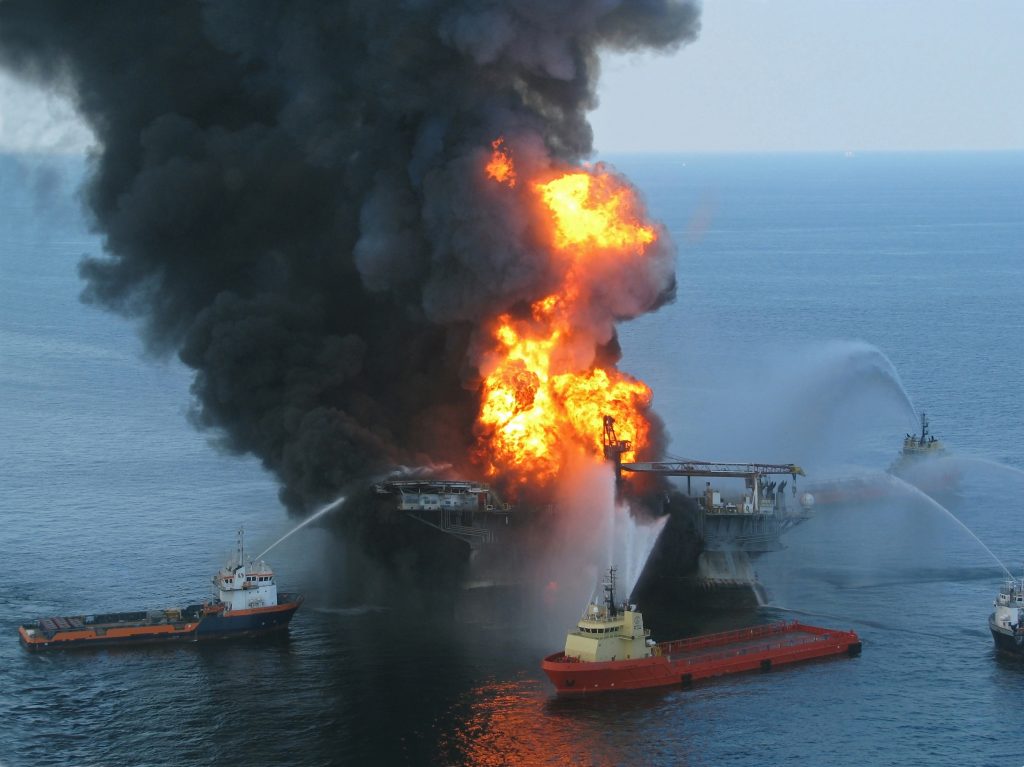
During an oil spill on water, oil floats to the surface because it is less dense than water, and the lightest hydrocarbons evaporate, decreasing the size of the spill but polluting the air. Then, naturally-occurring, hydrocarbon-degrading bacteria proliferate and begin to decompose the remaining oil, in a process that can take many years. After several months, only about 15% of the original volume may remain, but it is in thick asphalt lumps, a form that is particularly harmful to birds, fish, and shellfish. Physical cleanup operations can include skimmer ships that vacuum oil from the water surface, controlled burning and the use of dispersants (detergents that break up oil to accelerate its decomposition). Cleanup following the Exxon Valdez spill included physical strategies, as well as bioremediation.
Pathogens
Pathogens enter water primarily from human and animal faecal waste due to inadequate sewage treatment. In many underdeveloped countries, sewage is discharged into local waters either untreated or after only rudimentary treatment. According to the World Health Organization, in 2008 approximately 880 million people in the world (or 13% of the global population) did not have access to safe drinking water[1]. At the same time, about 2.6 billion people (or 40% of the global population) lived without improved sanitation, which is defined as having access to a public sewage system, septic tank, or even a simple pit latrine. Each year, approximately 1.7 million people die from diarrhoeal diseases associated with unsafe drinking water, inadequate sanitation, and poor hygiene, e.g., hand washing with soap. Almost all of these deaths are in developing countries, and around 90% of them occur among children under the age of 5 (Figure 10.27). Compounding the water crisis is the issue of social justice; poor people more commonly lack clean water and sanitation than wealthy people in similar areas. Globally, improving water, sanitation, and hygiene could prevent up to 9% of all disease and 6% of all deaths.
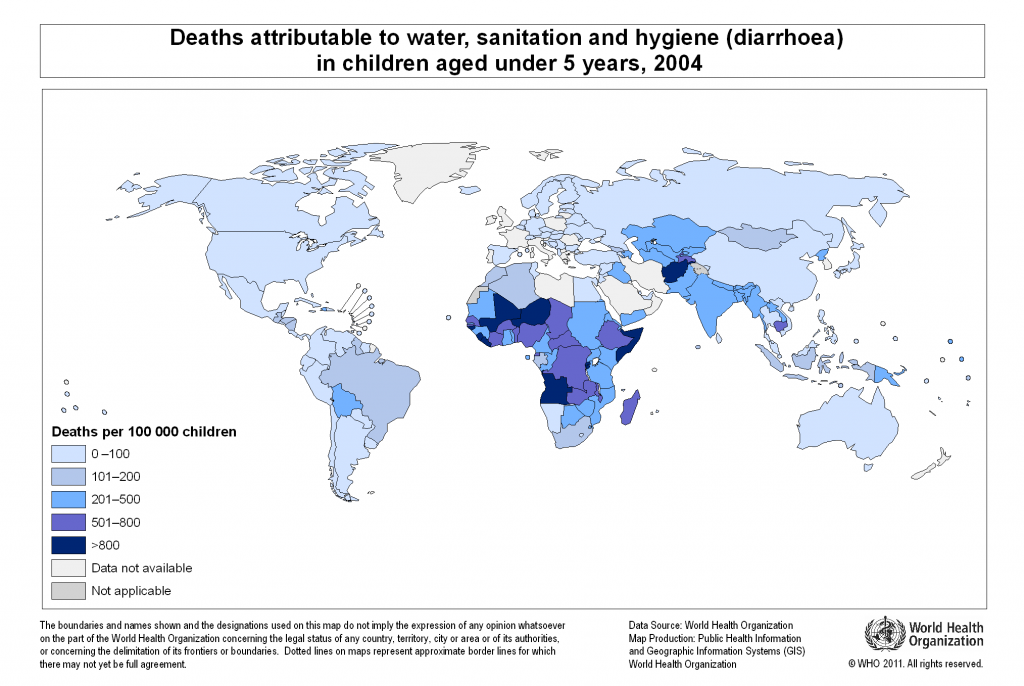
In developed countries untreated sewage discharge can occur from overflows of combined sewer systems, poorly managed livestock factory farms, and leaky or broken sewage collection systems. Water with pathogens can be remediated by adding chlorine or ozone, by boiling, or by treating the sewage in the first place.
- What sources and types of pollution can cause increased BOD?
- Why do certain types of pollution lead to decreased concentrations of dissolved O2, and how does this impact the ecosystem?
- In the marine environment, what are zones of “hypoxia” and why are they increasing in number and size?
Wastewater Treatment
Wastewater treatment is done at a sewage treatment plant in urban areas and through a septic tank system in rural areas.
Wastewater Treatment Plant
The main purpose of a wastewater treatment plant is to remove organic matter (oxygen-demanding waste) and kill bacteria; special methods also can be used to remove plant nutrients and other pollutants. The numerous processing steps at a conventional sewage treatment plant are depicted in Figure 10.28.
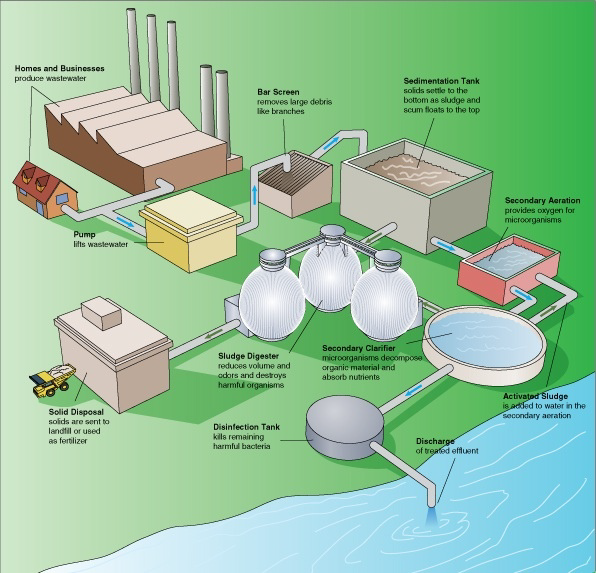
Sewage treatment involves pretreatment (screening and removal of sand and gravel), primary, secondary and tertiary treatment.
Primary Treatment
In primary treatment, sewage is stored in a sedimentation tank where solids (sludge) can settle to the bottom (sediment) and oil and lighter substances can rise to the top. These layers are then removed and the remaining liquid is sent to secondary treatment.
Secondary Treatment
Aerobic bacteria remove the dissolved and suspended organic components of the sewage. Some systems use fixed film systems, where the bacteria grow on filters, and the water passes through them. Suspended growth systems use “activated” sludge, where decomposing bacteria are mixed directly into the sewage. To ensure efficient decomposition, the sewage is aerated.
Tertiary Treatment
Several methods can be used to further disinfect sewage beyond primary and secondary treatment. Sand filtration, where water is passed through a sand filter, can be used to remove particulate matter. Sewage at this stage still has high levels of nitrogen and phosphorus and bacteria are used to remove both. At this stage, nitrogen is almost exclusively in the form of ammonia (NH3). This can be removed aerobically using nitrifying bacteria – lithotrophic bacteria that oxidize the ammonia to nitrite (NO2–) and to nitrate (NO3–). Nitrate is then converted to nitrogen gas anaerobically through denitrification by anaerobic bacteria and archaea. Other purification procedures employ physical and chemical techniques such as filtration, precipitation and ion exchange.
Disinfection
To eliminate any remaining microbes, including viruses, water is treated with chlorine, ozone, ultraviolet light, or bleach, and either discharged to surface waters (usually a local river) or reused for some other purpose, such as irrigation, habitat preservation, and artificial groundwater recharge.
Sludge
Sludge is the concentrated organic solids produced during primary and secondary treatment. It is treated in a variety of ways including landfill disposal, incineration, use as fertilizer, and anaerobic decomposition using sludge digesters. These involve anaerobic bacteria, including fermenters, and methanogenic archaea. The resulting methane (CH4) can be burned (releasing CO2) or used to generate electricity. Residual sludge can be incinerated, or condensed, heated to disinfect, and reused as fertilizer.
Septic systems
A septic tank system is an individual sewage treatment system for homes in rural and even some urban settings. The basic components of a septic tank system (Figure 10.29) include a sewer line from the house, a septic tank (a large container where sludge settles to the bottom and microorganisms decompose the organic solids anaerobically), and the drain field (network of perforated pipes where the clarified water seeps into the soil and is further purified by bacteria). Water pollution problems occur if the septic tank malfunctions, which usually occurs when a system is established in the wrong type of soil or maintained poorly.
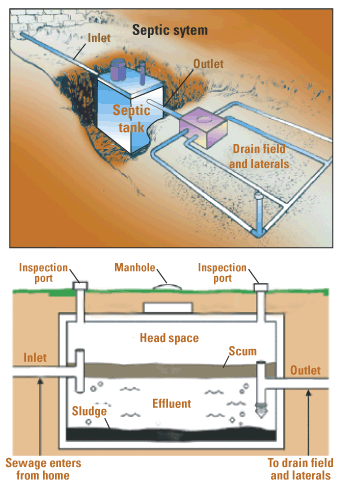
Bioremediation of Oil Spills
Marine bacteria capable of degrading oil exist throughout the world’s oceans. The vast majority are aerobic. Some of these break down the hydrocarbons in oil to smaller subunits; others, such as Alcanivorax borkumensis, produce surfactants that solubilize the oil (making it soluble in water). Some degrade the oil all the way to carbon dioxide. Under ideal conditions, it has been reported that up to 80 percent of the nonvolatile components in oil can be degraded within one year of the spill. Other oil fractions containing aromatic and highly branched hydrocarbon chains are more difficult to remove and remain in the environment for longer periods of time.
In addition to these naturally occurring oil-degrading bacteria, humans have selected and engineered bacteria for increased degradation efficacy and hydrocarbon range. The problem has always been the fact that while the oil represents a massive supply of carbon and energy for the bacteria, it is not enough to support a bloom of these bacteria. As already discussed, nitrogen and phosphorus are typically limiting nutrients in aquatic and marine environments. The most successful example of bioremediation of an oil spill, and the largest example of bioremediation, was in response to the Exxon Valdez spill. Between 1989 and 1991, a total of almost 50,000kg of nitrogen in N-containing fertilizers, was sprayed on oiled beaches (Figure 10.30). In 1992 the cleanup was declared concluded, with the remaining oil left to degrade naturally. This strategy worked because of the specific environmental and geographical conditions, combined with the nature of the oil and its discharge from the tanker.[2]. The oil was spilled was close to the surface, in the relatively enclosed space of Prince William sound, and most ended up on the shore, making it possible to apply the fertilizer and have it remain localized. Although there are also naturally-occurring oil solubilizing and degrading bacteria in the Gulf of Mexico, and the oil composition made it more easily degraded, because of the volume of the spill, combined with the environmental, geographic and oceanographic conditions, bioremediation was not an option for the Deepwater Horizon spill.[3]
Figure 10.30. Prokaryotes and bioremediation. (a) Cleaning up oil after the Exxon Valdez spill in Alaska, workers hosed oil from beaches and then used a floating boom to corral the oil, which was finally skimmed from the water surface. The populations of naturally-occurring oil-solubilizing and degrading bacteria underwent blooms in response to nitrogen fertilization. (b) One of the most catastrophic consequences of oil spills is the damage to fauna. (credit a: modification of work by NOAA; credit b: modification of work by GOLUBENKOV, NGO: Saving Taman)
For an easy-to-read discussion of the bioremediation efforts following the Exxon Valdez, and the challenges related to the Deepwater Horizon spill, read Atlas, R.M. and Hazen, T.C. 2011. Oil biodegradation and bioremediation: a tale of the two worst spills in U.S. history. Environ. Sci. Technol. 45: 6709–6715.
- Describe the 3 stages of a wastewater treatment process
- How does a septic tank purify sewage?
- What was the procedure used for bioremediation of the Exxon Valdez oil spill?
Key Takeaways
- Water pollution from untreated sewage is a major problem in developing countries, particularly for the poor. It leads to significant levels of morbidity and mortality.
- Water pollution in the form of eutrophication results from sewage, agricultural run-off and phosphates from detergents. This can lead to harmful blooms of primary producers.
- Water pollution can be monitored and by measuring the biological oxygen demand.
- Microbial bioremediation is the use of microbial metabolism to remove pollutants. The oldest and most successful example comes from sewage treatment
- Bioremediation has been used to remove agricultural chemicals that leach from soil into groundwater and the subsurface.
- Toxic metals and oxides, such as selenium and arsenic compounds, can also be removed by bioremediation.
- Probably one of the most useful and interesting examples of the use of prokaryotes for bioremediation purposes is the cleanup of oil spills, specifically the Exxon Valdez spill.
Multiple Choice
Short Answer
- How does eutrophication lead to an increase in BOD?
Critical Thinking
- Compare sewage bioremediation in an urban wastewater treatment plant vs a rural septic tank system.
- Identify some of the reasons why fertilization with local limiting nutrients was not an option for bioremediation of the Deepwater Horizon spill.
Media Attributions
- River_algae_Sichuan
- Red_tide
- Aquatic_Dead_Zones
- Deepwater_Horizon_offshore_drilling_unit_on_fire_2010
- Global_wsh_death_under5_2004
- Steps_in_a_typical_wastewater_treatment_process
- Septic system
- Exxon Valdez cleanup
- microbiology sign © Nick Youngson