10. Microbial Ecology and Applied Microbiology
10.4 Biotechnology
Learning Objectives
- Explain the concept of biotechnology and describe some of the earliest examples
- Describe the biotechnological applications of rhizobia, Baculoviruses, Bacillus thuringiensis and Agrobacterium tumifaciens
- Describe the role of genetic modification in agriculture
- Describe some of the diverse uses of microbes in the pharmaceutical industry and medicine
- Distinguish between probiotic bacteria, Faecal Microbial Transplants (FMT) and Microbial Ecosystem Therapy (MET)
Human life is only possible due to the action of microbes, both those in the environment and those species that call us home. Internally, they help us digest our food, produce vital nutrients for us, protect us from pathogenic microbes, and help train our immune systems to function properly. In fact, prokaryotes provide essential services not just to humans, but to all other organisms. Atmospheric nitrogen (N2), can be “fixed,” or converted into ammonia (NH3) by certain species of bacteria and archaea, but not by any eukaryotes, despite the fact that nitrogen is a major macroelement for all life, and in limiting concentrations in terrestrial environments.
Humans have been consuming fermented foods and beverages like beer, wine, bread, yogurt, cheese, and pickled vegetables for all of recorded history. Discoveries from several archeological sites suggest that even prehistoric people took advantage of fermentation to preserve and enhance the taste of food. Archaeologists studying pottery jars from a Neolithic village in China found that people were making a fermented beverage from rice, honey, and fruit as early as 7000 BC.[1] While it is likely that people first learned about fermentation by accident—perhaps by drinking old milk that had curdled or old grape juice that had fermented—they later learned to harness the power of fermentation to make products like bread, cheese, and wine.
According to the United Nations Convention on Biological Diversity, biotechnology is “any technological application that uses biological systems, living organisms, or derivatives thereof, to make or modify products or processes for specific use.”[2] The concept of “specific use” involves some sort of commercial application. Genetic engineering, artificial selection, antibiotic production, and cell culture are current topics of study in biotechnology and will be described in later chapters.
In more recent times, with the advent of recombinant DNA technology, genomics and our increasing understanding of the essential role of the microbes in any ecosystem, including that of the human gut, biotechnology is finding increasing uses in agriculture and medicine. In agriculture it can provide an organic means of fertilization, enhance resistance to disease, pests, and environmental stress to improve both crop yield and quality. Our understanding and use of probiotics has taken on new meaning with medical interventions to restore balance to the gut microbiota. And knowledge of the genetic makeup of our species combined with our ability to manipulate and fix mutant genes now provides methods to correct genetic diseases where the the nature of the mutation has been identified.
Early Biotechnology: Cheese, Bread, Wine, Beer, and Yogurt
Cheese production began around 4,000 to 7,000 years ago when humans began to breed animals and process their milk. Fermentation in this case preserves nutrients: Milk will spoil relatively quickly, but when processed as cheese, it is more stable. As for beer, the oldest records of brewing are about 6,000 years old and were an integral part of the Sumerian culture. Evidence indicates that the Sumerians discovered fermentation by chance. Wine has been produced for about 4,500 years, and evidence suggests that cultured milk products, like yogurt, have existed for at least 4,000 years. Only in recent years has the practice of pasteurization of yogurt been supplanted by the potential value of the lactic acid bacteria, or probiotic bacteria, used to make the yogurt (Figure 10.17).
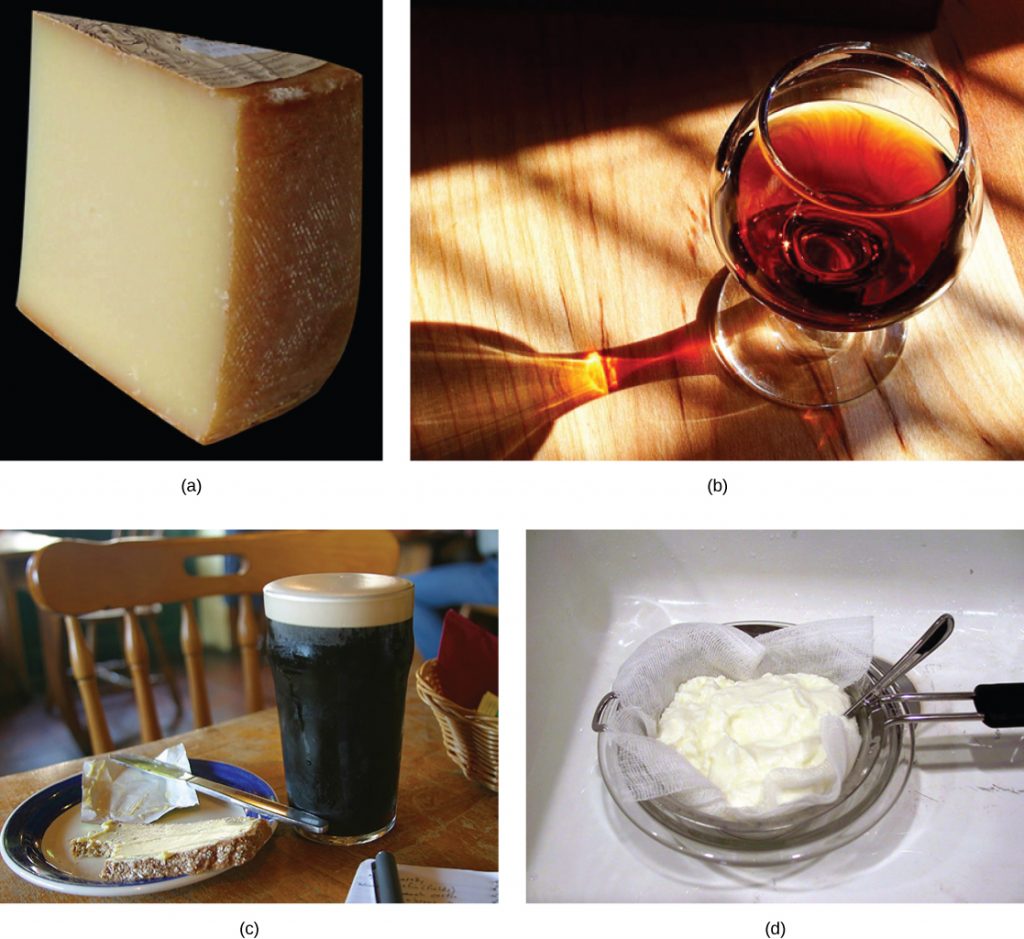
Transgenic Plants
Manipulating the DNA of plants (creating genetically modified organisms, or GMOs) has helped to create desirable traits such as disease resistance, herbicide, and pest resistance, as well as increased nutritional value and shelf life (Figure 10.18). Plants are the most important source of food for the human population. Using selective breeding practices, farmers developed ways to select for plant varieties with desirable traits long before modern-day biotechnology practices were established.
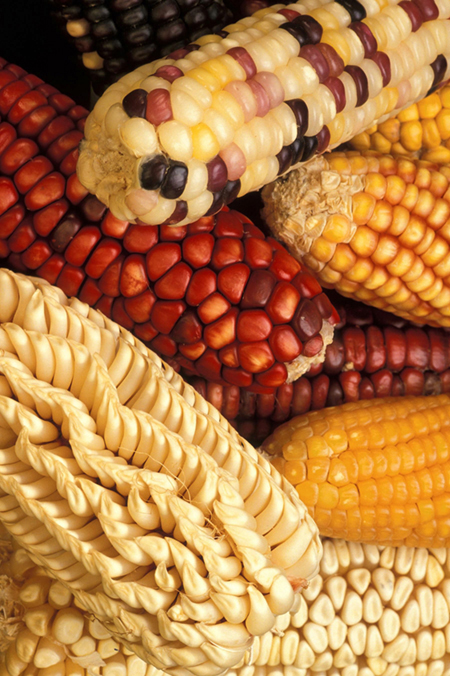
Transgenic plants have received DNA from other species. Because they contain unique combinations of genes and are not restricted to the laboratory, transgenic plants and other GMOs are closely monitored by government agencies to ensure that they are fit for human consumption and do not endanger other plant and animal life. Because foreign genes can spread to other species in the environment, particularly in the pollen and seeds of plants, extensive testing is required to ensure ecological stability. Staples like corn, potatoes, and tomatoes were the first crop plants to be genetically engineered.
Transformation of Plants Using Agrobacterium tumefaciens
In plants, galls are tumours caused by the bacterium Agrobacterium tumefaciens. In nature, A. tumefaciens has a tumour-inducing plasmid, the Ti plasmid, containing genes that integrate into the infected plant cell’s genome.By manipulating the plasmid to carry foreign genes for desired traits, researchers have exploited the natural transfer of DNA from Agrobacterium to introduce DNA fragments of their choice into plant.
Organic and Biological Insecticides
The Gram positive bacterium Bacillus thuringiensis (Bt) produces protein crystals that are toxic to many insect species that feed on plants. Within a few hours of consuming the toxin, the insect stops feeding on the plant and death occurs within a couple of days. The toxin can be purified from the bacterium, dried to a powder, and applied to crops. More recently, the gene for the crystal toxin has been cloned from the bacterium and introduced into plants, allowing plants to produce their own Bt toxin. The toxin is safe for the environment and non-toxic to mammals (including humans). As a result, it has been approved for use by organic farmers as a natural insecticide. There is some concern, however, that insects may evolve resistance to the Bt toxin in the same way that bacteria have evolved resistance to antibiotics.
Baculoviruses are insecticidal viruses belonging to the Baculoviridae family. These have found broad use as organic insecticides. The family currently consists of over 600 recognized species, each of which is highly host-specific, infecting at most, a few closely-related species of Lepidoptera (butterflies and moths), Hymenoptera (sawflies), and Diptera (mosquitoes) insect clades.[3] Baculoviruses have two forms: the budded form that allows the virus to spread within the host cells, and the occlusion-derived virus found in large polyhedral occlusion bodies (OBs). Infection of a susceptible host begins with the consumption of OBs (Figure 10.19). The protein matrix of the OB dissolves upon exposure to the alkaline midgut of the insect, releasing the viral particles and degradative enzymes that break down the peritrophic membrane lining the midgut, allowing the virus to penetrate the midgut epithelial cells. The virus replicates and spreads within the host as budded virus, but during the late stages of infection, occlusion bodies form and the degradative enzymes that facilitated the initial penetration of the host then cause host cell lysis and ultimately, the dissolution of the insect. These OBs are highly stable and are the basis for biocontrol of a variety of insect pests including Gypsy Moth larvae (Lymantria dyspar).
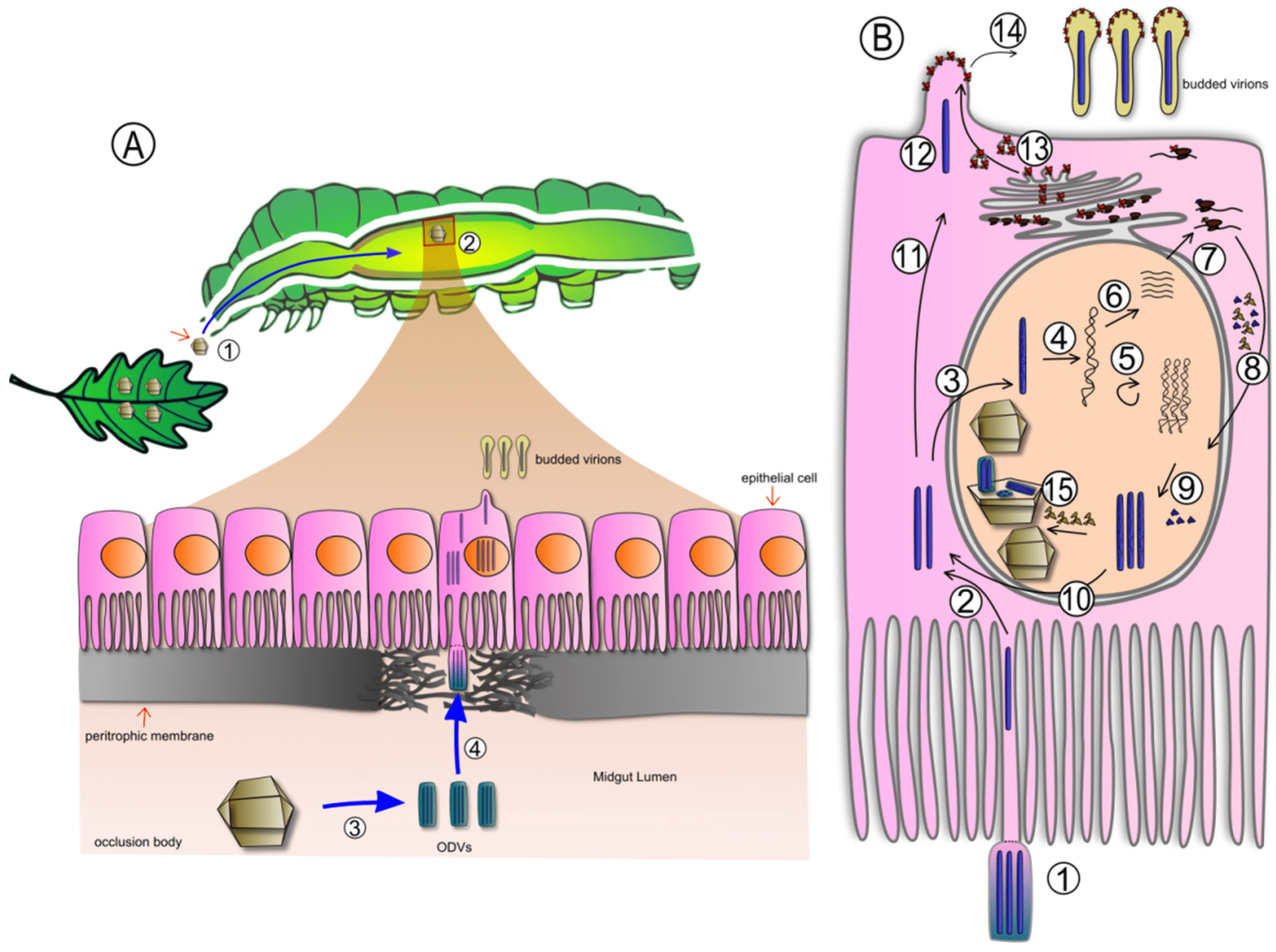
Rhizobia and Biofertilization
Nitrogen is an integral component of two of the major cellular polymers: nucleic acids and proteins. It is usually the most limiting element in terrestrial ecosystems, with atmospheric nitrogen, N2, providing the largest pool of available nitrogen. However, eukaryotes cannot use atmospheric nitrogen to synthesize macromolecules. Fortunately, nitrogen can be “fixed” or converted into a more accessible form, ammonia (NH3), either biologically or abiotically.
Abiotic nitrogen fixation occurs as a result of physical processes such as lightning or by industrial processes. Biological nitrogen fixation is carried out exclusively by various species of diazotrophic bacteria and archaea (see Biosynthesis). After photosynthesis, diazotrophy is arguably the most important biological process on Earth. Nitrogen fixation is a series of highly endergonic redox reactions requiring the hydrolysis of large amounts of ATP:
N2 + 16ATP + 8e− + 8H+ → 2NH3 + 16ADP + 16Pi + H2
The diazotrophs fix approximately 100 to 180 million metric tons per year, which contributes about 65 percent of the nitrogen used in agriculture. Cyanobacteria are the most important nitrogen fixers in aquatic environments. In soil, members of the genera Clostridium and Azotobacter are examples of free-living, nitrogen-fixing bacteria. Other bacteria, the rhizobia, live symbiotically with the roots of legume plants. Symbionts may fix more nitrogen in soils than free-living organisms by a factor of 10. The rhizobia form nodules on legume plant roots: specialized structures in which nitrogen fixation occurs (Figure 10.20). Nitrogenase, the enzyme that fixes nitrogen, is inactivated by oxygen, however within the nodule is a form of plant haemoglobin called leghaemoglobin, which binds O2, protecting the nitrogenase.
Symbiotic nitrogen fixation provides a natural and inexpensive plant fertilizer: it reduces atmospheric nitrogen to ammonia, which the plants can use as a nitrogen source. The use of rhizobia and legumes is an excellent alternative to chemical fertilization and is of special interest to sustainable agriculture, which seeks to minimize the use of chemicals and conserve natural resources. Through symbiotic nitrogen fixation, the plant benefits from using an endless source of nitrogen: the atmosphere. The bacteria benefit from the carbohydrates produced by the plant during photosynthesis and having a protected niche. In addition, the soil benefits from being naturally fertilized, preventing the nitrogenous run-off associated with chemical fertilizers and the resulting eutrophication of local aquatic and marine ecosystems.
Why are legumes so important? Some of the most important legumes consumed by humans are soybeans, peanuts, peas, chickpeas, and beans. Other legumes, such as alfalfa, are used to feed cattle.
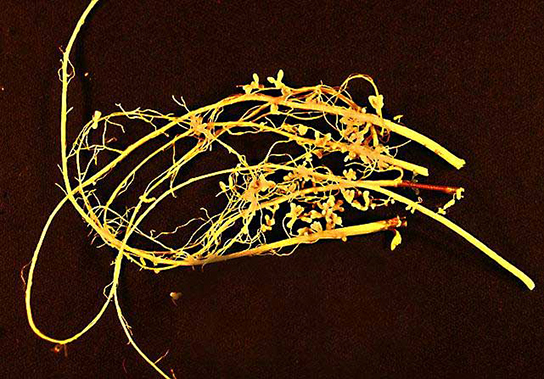
- List some of the most ancient types of biotechnology and some of the more recent ones
- What role does the bacterium Agrobacterium tumifaciens play in biotechnology?
- What is the relevance of the rhizobia to plant growth and agriculture?
Production of Vaccines, Antibiotics, and Hormones
Traditional vaccination strategies use weakened or inactive forms of microorganisms, viruses or toxins to stimulate the immune system. Modern techniques use specific genes of microorganisms cloned into vectors and mass-produced in bacteria to make large quantities of specific substances, “antigens”, to stimulate the immune system. The antigen is then used as a vaccine. Vaccines are described in more detail in Section 19.5.
Antibiotics are naturally produced by microorganisms such as fungi; penicillin is perhaps the most well-known example, produced by the mould Penicillium notatum. Other antibiotics are naturally produced by different strains of the actinomycete bacteria. Certain antibiotics are synthesized, or chemically modified, in the organic chemistry lab. Antibiotics are described in more detail in various sections of Chapter 15.
Recombinant DNA technology was used to produce large-scale quantities of the human hormone insulin in E. coli as early as 1978. Previously, it was only possible to treat diabetes with pig insulin, which caused allergic reactions in many humans because of differences in the insulin molecule. In addition, human growth hormone (HGH) is used to treat growth disorders in children. The HGH gene was cloned from a cDNA (complementary DNA) library and inserted into E. coli cells by cloning it into a bacterial vector. Some of these are described further in Chapter 13: Modern Applications of Microbial Genetics.
Genetic Diagnosis and Gene Therapy
Mutations in the BRCA genes may increase the likelihood of developing breast and ovarian cancers in women and some other cancers in women and men. A woman with breast cancer can be screened for these mutations. If one of the high-risk mutations is found, female relatives may also be screened for that mutation. Genetic testing is also offered for fetuses (or embryos with in vitro fertilization) to determine the presence or absence of disease-causing genes in families with specific debilitating diseases. Knowledge of the genetic basis for heritable diseases continues to increase, and with that knowledge, the potential for correcting the defect through gene therapy.
Gene therapy is a genetic engineering technique that, in its simplest form, involves the introduction of a non-mutated gene at a random location in the genome to provide a source of a functional protein. The non-mutated gene is usually introduced into diseased cells as part of a vector transmitted by a virus, such as an adenovirus, that can infect the host cell and deliver the foreign DNA into the genome of the targeted cell (Figure 10.21). To date, gene therapies have been primarily experimental procedures in humans. A few of these experimental treatments have been successful, but the methods may be important in the future as the factors limiting its success are resolved. Gene therapy is discussed in more detail in Section 13.4.
MICRO CONNECTIONS: “C. diff” Infections and Microbial Ecosystem Therapy
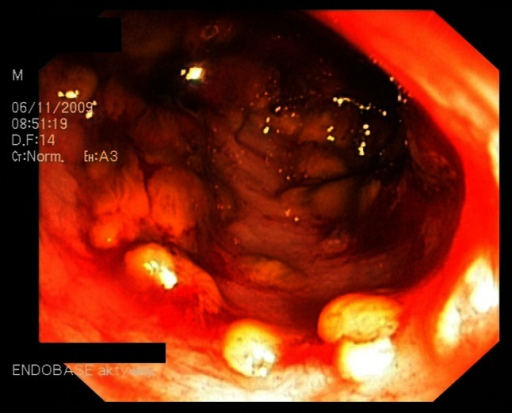
- Describe some of the pharmaceutical applications of microbes.
- What is gene therapy and how do microbes play a role?
- What is FMT and MET?
Key Takeaways
- The oldest examples of the deliberate harnessing of microbial processes are the fermented foods and beverages that have been part of our diet for millenia
- Antibiotics may be produced naturally by actinobacteria and various moulds
- Vaccines use inactivated microbes, microbial structures or toxins as antigens to generate immunity
- Hormones and vaccines are examples of products obtained by recombinant DNA technology.
- The mutualistic relationship of the Rhizobia with legumes has been exploited as a natural alternative to fertilization, by providing fixed nitrogen, in the bioavailable form of NH3, to the plant.
- Transgenic organisms possess DNA from a different species, usually generated by molecular cloning techniques.
- Transgenic plants can be created using modified Ti plasmids of Agrobacterium tumefaciens and have been created to improve the characteristics of crop plants. Bt corn is one example.
- Gene therapy involves the correction of a genetic defect through incorporation of a functioning gene into the genome of individuals with a heritable disease
Multiple Choice
Short Answer
- What is Bt corn?
- How do legumes provide the necessary conditions for N-fixation by rhizobia?
Critical Thinking
- Compare and contrast probiotics, FMT and MET.
- Describe 3 diverse uses of microorganisms in the pharmaceutical industry.
- Why are Baculovirus OBs so useful as insecticides?
Media Attributions
- Microbial food products
- Maize
- Baculovirus life cycle
- Rhizobia root nodules
- Pseudomembranous colitis
- P.E. McGovern et al. “Fermented Beverages of Pre- and Proto-Historic China.” Proceedings of the National Academy of Sciences of the United States of America 1 no. 51 (2004):17593–17598. doi:10.1073/pnas.0407921102. ↵
- http://www.cbd.int/convention/articles/?a=cbd-02, United Nations Convention on Biological Diversity: Article 2: Use of Terms. ↵
- Haase, S., Sciocco-Cap, A., and Romanowski, V. "Baculovirus Insecticides in Latin America: Historical Overview, Current Status and Future Perspectives." PLoS One. 2017 Dec 18;12(12):e0189680. doi: 10.1371/journal.pone.0189680. ↵