Appendix 6: Organic Reaction Mechanisms
Chlorination of Methane
In the chlorination of methane, we see a particular property of hydrogen atoms in organic molecules in general. They are labile, meaning they can be easily exchanged for other things. In this case, the bonding pair of the C-H bond moves to one Chlorine atom in the Cl2 while the Cl-Cl bonding pair jumps to form a new bond between the other Cl and the Carbon atom.
Cracking Reaction:
The cracking reaction involves the homolitic cleavage of a C-C bond. This reaction needs a catalyst to function with any efficiency. This means that the two electrons are divided evenly between the two atoms. This results in two fragments, each with a lone electron (dangling bond). The two fragments are radicals and are not very stable. To stabilize the pair, a hydrogen homolitically cleaves from a carbon neighboring one of the two carbons used to be bonded together leaves that carbon and migrates over to the other fragment to complete the bonding on that fragment. The two carbons on the fragment from which the hydrogen left now form a double C=C bond and all electrons are paired up again. See the diagram below for a visual representation.
Note that the double bond could have formed on the 4-carbon fragment as well, to produce 1-butene and pentane in more or less equal amounts. So, each bond broken in a cracking reaction could form two sets of compounds for a total of 4 products.
When HCl is added to an alkene or an alkyne, the pi electrons from the multiple bond form a new bond with the proton on the HCl, leaving behind a carbocation where the ene was and a chloride ion, which then combine in a second step to make the product. Because, in this case, the ene was symmetric, it does not matter which side of the double bond the chloride ends up on, the product will be the same.
Bromine addition to an alkene or alkyne works the same way as the HCl addition, except this time, one Br atom ends up on each carbon from the ene group.
The hydrogenation reaction will not occur without catalysis. In this mechanism, a sheet of metal acts as a catalyst. The molecules both adsorb onto the surface of the metal (blue rectangle). The hydrogen bonds easily break in this condition and they then migrate to the pi electrons in the alkene (or alkyne). The product then desorbs off the metal surface. This is an example of heterogeneous catalysis. The catalyst is not the same phase as the reactants.
Markovnikov Reactions
In the addition of an acid (say, HCl) to an ‘ene’ group, the proton (electrophile) first attacks the p electrons of the double bond, forming a new s bond with one of the carbons, leaving the other carbon with one too few electrons (carbocation). Then, in the second step the nucleophile Cl– attacks the carbocation, filling it’s empty orbital and forming a new bond.
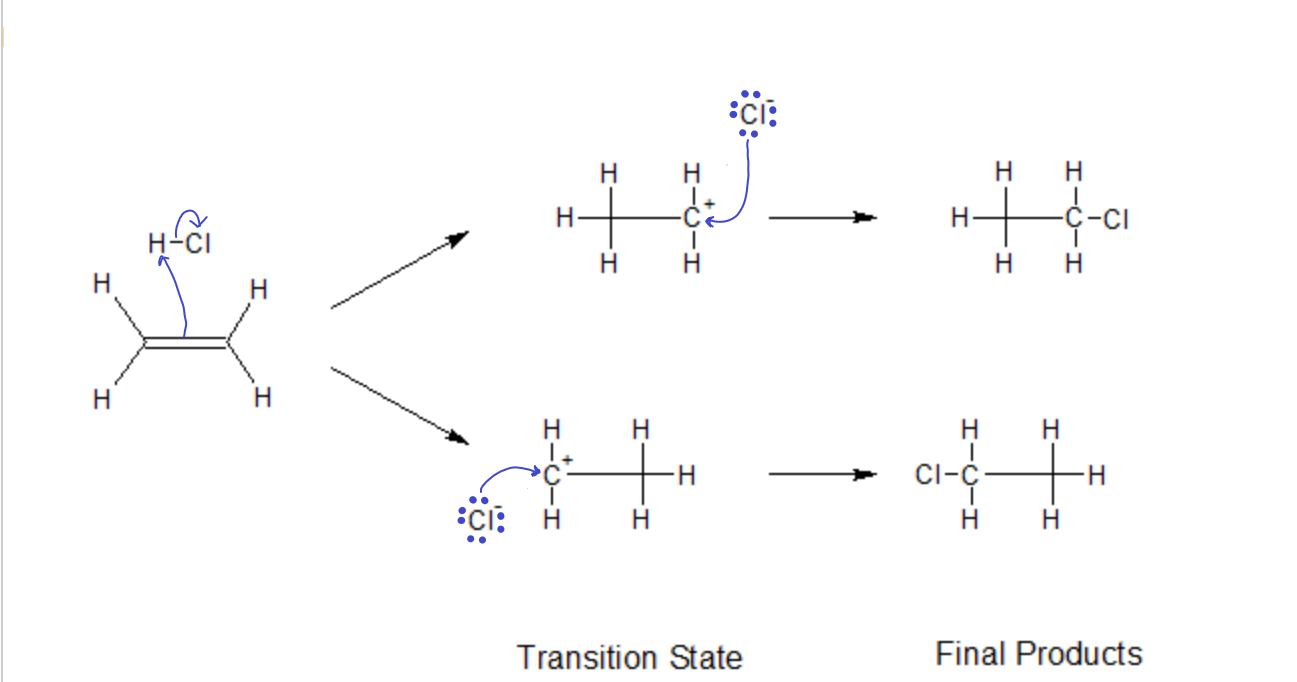
The transition state in both these first steps have to be stable enough to stick around long enough for the Nucleophile to attack. If it were to decompose too quickly, the reaction would never reach completion. Since both sides of the ‘ene’ group are identical in this example, both products will be equally likely. Here, they are indistinguishable anyway.
In the second step, the reactive part of the transition state is the carbocation (it has the charge). Anything we do to stabilize the carbocation (reduce the charge) will result in that particular reaction path being favored because it will help the transition state ‘stick around’ long enough for the second step to happen.
If we put on one of the carbons a substituent that pushes electron density onto that carbon, then that carbon will be stabilized if it becomes the carbocation. If, we put an electron-withdrawing (highly electronegative) substituent on a carbon then it will be destabilized as a carbocation. It turns out that R groups (alkyl groups) are electron donating (they have lower electronegativity than a Carbon with double bonds) while a halogen would be electron withdrawing (it has high electronegativity).
Thus, for a hydrocarbon-only compound with an asymmetrically substituted ‘ene’ group, the carbon with more R groups will be stabilized relative to the carbon with more hydrogens (since the R group will push electron density onto the carbocation, distributing the positive charge and stabilizing it.)
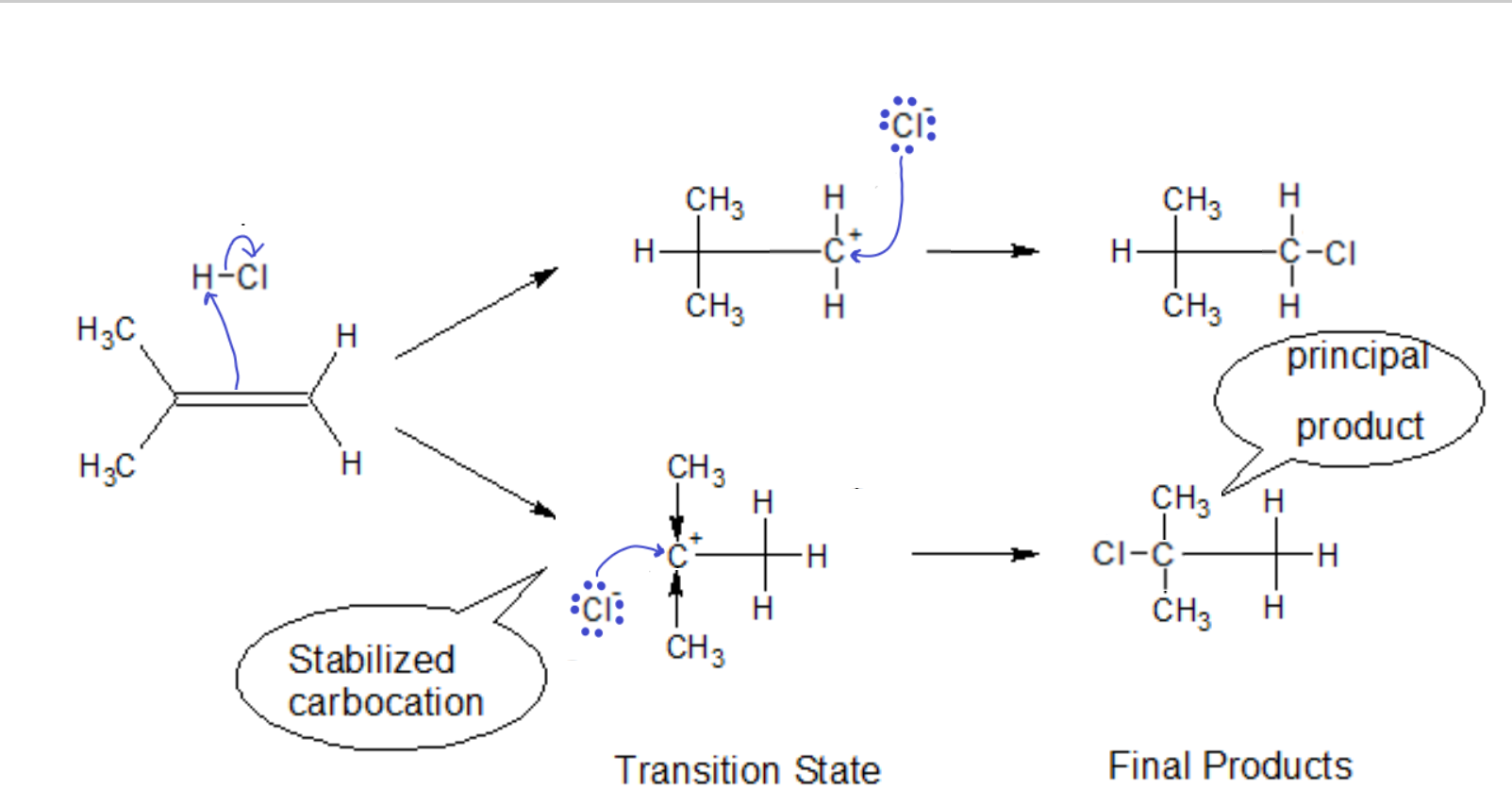
In the case of non-alkyl groups on the ‘ene’ carbon, we must first see if it is electron donating (like an alkyl) or electron withdrawing. If we have Cl as a substituent, the opposite reaction path becomes favored. The highly electronegative Cl tries to pull electron density away from the already positively charged carbon. The carbocation will be thusly destabilized, i.e., the transition state will quickly dissociate back to the starting materials, making the second step of that reaction path less likely to occur.
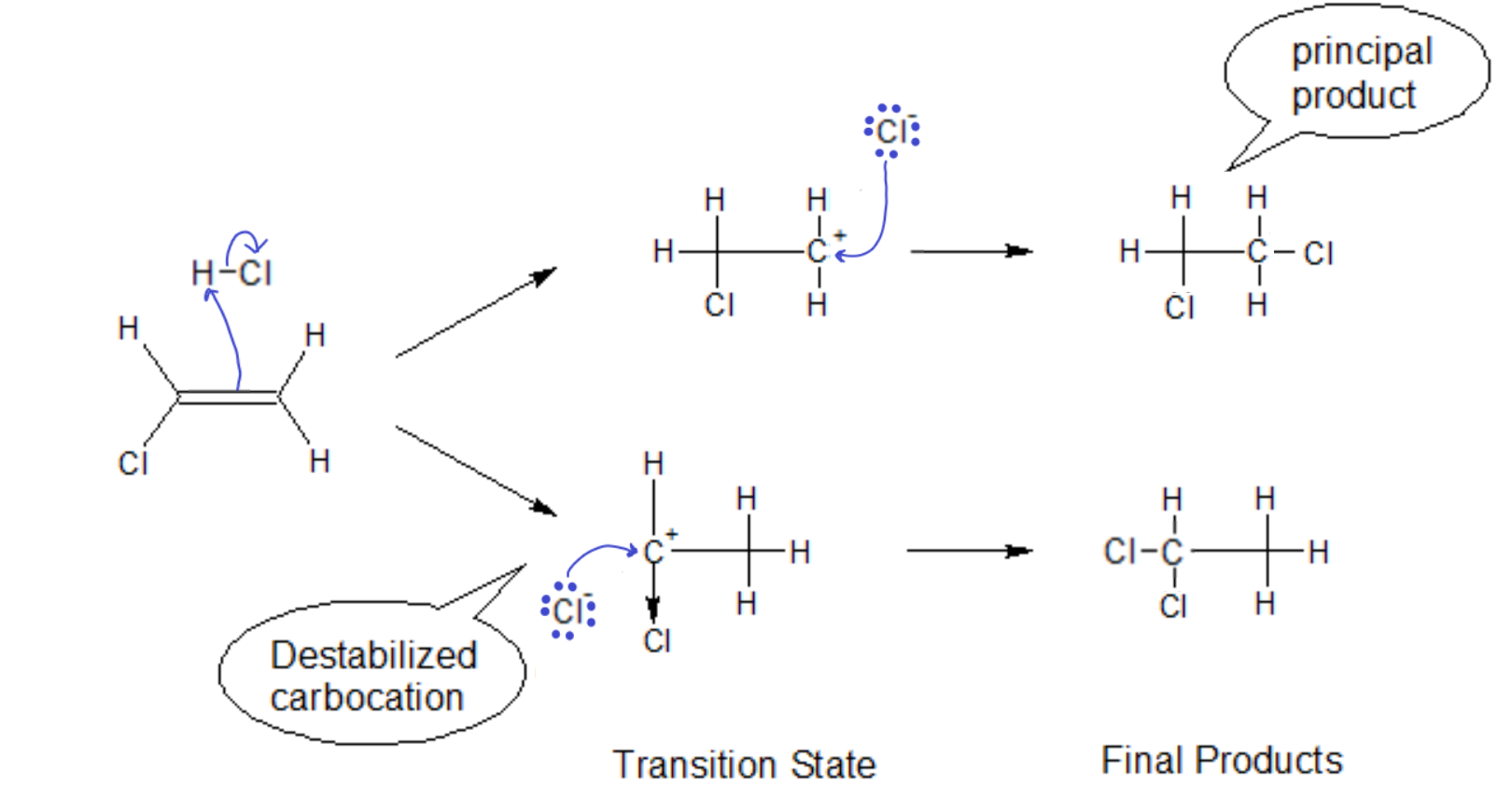
Markovnikov’s Rule NEW FORM: In the addition of an acid to an alkene, the proton will go to the side that leaves behind the more stabilized carbocation.
Now, using this new definition, both reactions 2 and 3 can be seen as obeying Markovnikov’s rule. There is no anti-Markovnikov reaction.