9.9 Ventilation in Acute Respiratory Distress Syndrome
Acute Respiratory Distress Syndrome (ARDS) is a complex clinical syndrome that can become a severe and life-threatening condition that affects the lungs, leading to acute respiratory failure. It is characterized by rapid and profound inflammation of the alveoli, which makes it difficult for oxygen to enter the bloodstream and carbon dioxide to be eliminated properly. While there is extensive literature available on the pathophysiology of ARDS, we will focus on ventilation management strategiesin this book .
Ventilating patients with ARDS represents one of the biggest challenges for clinicians in the ICU. Even tough advances in mechanical ventilation and critical care medicine have been made in the past years, mortality due to ARDS in hospitals is still high. The intricate nature of maintaining adequate oxygenation in these patients has spurred extensive research into achieving optimal PEEP, with the ultimate goal of opening the lung and improving respiratory function. The concept of “open lung ventilation” is sometimes intertwined with ARDS ventilation, as some clinicians strive to maximize lung recruitment and enhance gas exchange in these critically ill patients. Emphasizing this close relationship between open lung ventilation and ARDS management underscores the vital importance of refining ventilation strategies to enhance patient outcomes. To differentiate ARDS from other pathologies with similar presentation, an international consensus group of critical care experts has established the most up to date definition of the disease. Because this group met in Berlin, Germany, this definition is known as the Berlin definition of ARDS, 2012.
ARDS Berlin Definition |
|
---|---|
Timing | Within 1 week of original insult or new or worsening respiratory symptoms |
Chest Imaging | Bilateral opacities not fully explained by effusions, lobar/ lung collapse, or nodules. |
Origin of edema |
Respiratory Failure not fully explained by cardiac failure or fluid overload. Need objective assessment to exclude hydrostatic edema if no risk factors are present |
Oxygenation |
[latex]\small{\textbf{Mild: }200\text{ mmHg}<\text{PaO}_2/\text{FiO}_2\leq300\text{ mmHg with PEEP or CPAP}\geq5\text{ cmH}_2\text{O}}[/latex] [latex]\small{\textbf{Moderate: }100\text{ mmHg}<\text{PaO}_2/\text{FiO}_2\leq200\text{ mmHg with PEEP or CPAP}\geq5\text{ cmH}_2\text{O}}[/latex] [latex]\small{\textbf{Severe: }\text{PaO}_2/\text{FiO}_2<100\text{ mmHg with PEEP or CPAP}\geq5\text{ cmH}_2\text{O}}[/latex] |
Data Source: The ARDS Definition Task Force, 2012. |
The initial management of ARDS, of course, involves addressing and stabilizing the underlying disease that triggered its onset. Current guidelines from the ARDS network recommend a cautious approach in managing the injured lung, utilizing ventilation techniques characterized by low tidal volume and pressure known as Lung Protective Ventilation.
Key Takeaway
The goals of mechanical ventilation in the management of ARDS are to support oxygenation, avoid circulatory compromise, and avoid ventilator-induced lung injury. Lung protective ventilation includes guidelines that attempt achievement of these goals.
The therapeutic approach is based on the severity of lung damage and injury. As soon as lung injury is suspected, low tidal volume should be considered (on average [latex]6\text{ mL/Kg}[/latex], with a range from [latex]\text{4 - 8 mL/Kg IBW}[/latex]). As the disease progresses, and oxygenation worsens, it becomes crucial to find optimal PEEP and titrate FiO2 to maintain [latex]\text{PaO}_2>55\text{ mmHg}[/latex]. For more information, please refer to the previous section, covering methods for finding optimal PEEP. The low volumes and pressure used with lung protective ventilation can become uncomfortable for patients, leading to patient-ventilator asynchrony. This ventilation strategy may also lead to respiratory acidosis with higher than normal PaCO2, often leading clinicians to allow permissive hypercapnia in certain cases.
As clinicians learn more about management of ARDS, research brings new evidence into practice, so guidelines and recommendations change often, and the definition of ARDS is being challenged to include patients treated with high flow nasal cannula prior to intubation. The European Society of Intensive Care Medicine has recently published the latest guidelines.
ESICM 2023 Recommendations for ARDS Ventilation
• Use low tidal volume ventilation [latex]\text{(4 - 8 mL/Kg IBW)}[/latex]
• Do not use prolonged high-pressure recruitment maneuvers
• Use prone position to reduce mortality
• Do not routinely use continuous infusions of neuromuscular blockade
• Refer patients who meet criteria for ECMO (extracorporeal membrane oxygenation) to ECMO centers
Please read the following article for more information on ESICM guidelines for ARDS:
Prevention really is the best medicine ! Whenever possible, intubation and ventilation should be avoided. Early recognition and diagnostic is important. For non-intubated patients with acute hypoxemic respiratory failure not due to cardiogenic pulmonary
edema or chronic lung disease, High-flow nasal oxygen (HFNO) is recommended versus conventional oxygen therapy to reduce risk of intubation.
So, how do we ventilate ARDS patients?
There is no strong evidence to support one ventilation mode versus the other. However, in order to follow the recommended guidelines for lung protective ventilation and observe patient safety, the ventilation mode employed for each patient must deliver the tidal volume recommended of [latex]\text{4 - 8 mL/Kg IBW}[/latex] with minimal pressure while ensuring adequate gas exchange. Refer to Chapter 5 for a review of basic ventilation modes.
Airway Pressure Release Ventilation (APRV)
One ventilation mode we have not yet covered, often referred to as an advanced mode, plays a role in ventilating patients with ARDS— Airway Pressure Release Ventilation (APRV). This mode has shown promising results in managing patients with oxygenation challenges, as it facilitates alveolar recruitment, thereby promoting the “open lung concept.”
APRV’s efficacy stems from its ability to enhance alveolar recruitment, allowing for the optimal expansion of collapsed lung areas. By periodically releasing airway pressure, APRV permits the lungs to reach a more natural equilibrium, promoting better gas exchange and oxygenation.
The evidence supporting the success of APRV in ARDS patients is substantial. This ventilation mode has proven effective in enhancing oxygenation and respiratory compliance, ultimately contributing to improved patient outcomes.
The terminology we are accustomed to using when describing ventilation modes changes slightly in APRV.
Ventilation Settings in APRV |
---|
[latex]P_{high}\text{: 25 - 35 cmH}_2\text{O (keep <40 cmH}_2\text{O)}[/latex] |
[latex]P_{low}\text{: 0 - 5 cmH}_2\text{O}[/latex] |
[latex]T_{high}\text{: 5.2 - 5.6 s}[/latex] |
[latex]T_{low}\text{: 0.5 - 1.0 s}[/latex] |
[latex]\text{FiO}_2\text{ based on SpO}_2\text{, PaO}_2\text{, P/F}[/latex] |
APRV can be described as a pressure controlled mode that uses inverse ratio ventilation. While up to this point we discussed [latex]\text{I:E}[/latex] ratio of [latex]\text{1:2}[/latex] or [latex]\text{1:3}[/latex], in APRV, we are looking at [latex]\text{I:E}[/latex] ratio of [latex]\text{4:1}[/latex] or higher. Now, if you try to follow this pattern for your own breathing, inhale for 5 seconds and exhale for 1, then inhale again for 5 seconds and repeat, you will soon become uncomfortable. Advances in technology allowed ventilator manufactures to add features that allow patients to breathe spontaneously when this mode is used.

The concept behind this approach is to elevate the mean airway pressure to open the lung effectively. With this ventilation mode, the ventilator establishes a higher pressure level, which serves as the baseline for all breaths. This baseline pressure, known as [latex]P_{high}[/latex], plays a crucial role in increasing mean airway pressure by extending the inspiratory time.
To facilitate gas exchange and CO2 removal from the lungs, the ventilator allows a controlled release of the high pressure to a predetermined lower pressure for a brief period, referred to as [latex]P_{low}[/latex]. In this context, we replace conventional ventilation settings such as inspiratory time [latex](T_i)[/latex] with Time high [latex](T_{high})[/latex] and expiratory time with Time low [latex](T_{low})[/latex].
You will remember from discussing conventional ventilation modes, such as pressure control and volume control, that the length of the expiratory time was set so to avoid air trapping (intrinsic PEEP). If you look closely at the above diagram depicting the pressure-time waveform of APRV, you will realize that time for expiration (release) is very short, likely causing air trapping. APRV, in fact, relies on intrinsic PEEP to maintain alveolar recruitment (open lung).
It is worth noting that this mode does not rely on setting a specific respiratory rate. Instead, it allows patients to take spontaneous breaths during both [latex]T_{high}[/latex] and [latex]T_{low}[/latex], thereby contributing to the elevation of mean airway pressure. This approach particularly benefits patients who are capable of spontaneous breathing. However, in cases where patients are unable to breathe spontaneously, the respiratory rate is determined by the settings of [latex]T_{high}[/latex] and [latex]T_{low}[/latex].
There is also no setting for tidal volume in APRV. Similar to any pressure controlled mode, tidal volume is determined by pressure—the setting [latex](\Delta P)[/latex].
Example
For a patient who is unable to take spontaneous breaths, respiratory rate is determined by [latex]T_{high}[/latex] and [latex]T_{low}[/latex] settings:
[latex]\begin{array}{ccc}T_{high}=5.5\text{ s and }T_{low}=0.5\text{ s}&\text{TCT}=T_{high}+T_{low}=6\text{ s}\end{array}[/latex]
We know [latex]\text{TCT}=\frac{60}{RR}[/latex]
[latex]\begin{align*}\text{TCT}&=\small{\frac{60}{RR}}\\RR&=\small{\frac{60}{\text{TCT}}}\\RR&=\small{\frac{60}{6}}\\RR&=10\text{ bpm}\end{align*}[/latex]
Here is a video demonstration of APRV ventilation:
Object Lesson
Imagine the movement of air in and out of the lungs like the ocean with a high tide and low tide. Start with [latex]P_{high}[/latex] as the high tide. At certain time intervals, the tide retreats briefly to a lower level [latex](P_{low})[/latex]. During this “low tide” phase, gas exchange and CO2 removal occur, giving the lungs a chance to “breathe.”
Just as the tide constantly shifts, APRV continues this rhythmic cycle of elevated pressure [latex](P_{high})[/latex] followed by a brief release [latex](P_{low})[/latex], encouraging spontaneous breaths like waves lapping at the shore. This natural flow, driven by patient effort, further contributes to optimizing lung function and increasing mean airway pressure.
Ventilation Adjustments and Troubleshooting in APRV
Just like in conventional ventilation, the primary objectives of maintaining ventilation with APRV are twofold: to restore a balanced acid-base status and to ensure the comfort of the patient. Naturally, the ultimate aim remains to liberate the patient from mechanical ventilation as swiftly as is feasible.
To manage oxygenation problems, consider increasing mean airway pressure (MAP). Refer to the pressure-time waveform for a visual understanding of parameters that impact MAP.
To improve oxygenation:
- Increase [latex]\Delta P[/latex], by increasing [latex]P_{high}[/latex]
- Decrease [latex]P_{low}[/latex] (if not already at zero)
- Increase [latex]T_{high}[/latex]
- Decrease [latex]T_{low}[/latex]
- Decrease sedation to allow spontaneous breathing
FiO2 should be kept at minimum; however, this parameter can be manipulated when MAP cannot be increased safely.
As oxygenation improves, consider decreasing MAP, in a “drop and stretch” style approach. “Drop” (decrease) [latex]\Delta P[/latex] and “stretch” (increase) [latex]T_{high}[/latex] to reduce the number of releases. Consider changing the mode to a spontaneous mode such as pressure support ventilation when [latex]P_{high}[/latex] is around [latex]\text{15 - 16 cmH}_2\text{O}[/latex].
Ventilation (pACO2) is influenced by minute volume, which is determined by tidal volume and respiratory rate. To make adjustments that impact ventilation in APRV, consider changing:
- Tidal volume moved with pressure releases [latex](\Delta P)[/latex] (Ex. if PaCO2 is high, consider increasing [latex]\Delta P[/latex])
- Number of pressure releases
- Allow spontaneous breaths (to add to minute volume)
Note that spontaneous breaths can be supported with pressure support ventilation.
Additional Supportive Therapy in ARDS
The heterogeneity of ARDS with the subsequent impairment in gas exchange, especially oxygenation, often requires additional measures to supporting ventilation.
Prone ventilation is a technique that has been shown to improve oxygenation and outcomes in certain cases of respiratory failure. It involves placing the patient in a face-down (prone) position on the bed, as opposed to the usual supine (face-up) position.
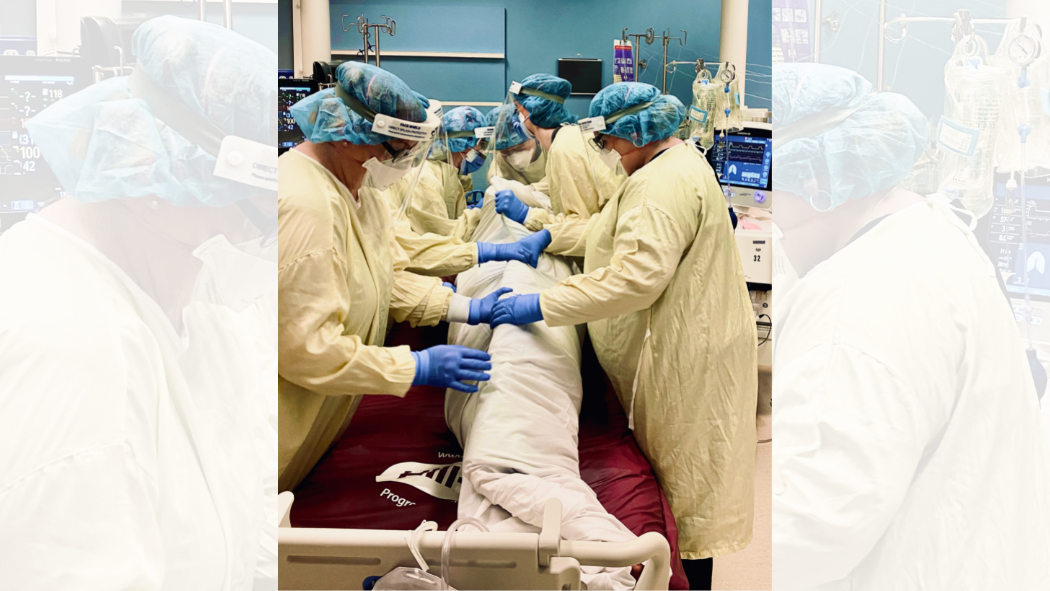
The primary goal of prone ventilation is to enhance oxygenation and gas exchange by redistributing the ventilation and perfusion patterns in the lungs. Prone positioning helps to recruit under-ventilated lung regions and improve the matching of lung ventilation with blood flow, thus increasing oxygen uptake and reducing shunting. For a quick review of Ventilation/perfusion patterns in the lungs, see Chapter 7.1.
By enhancing gas exchange, prone positioning can help alleviate ventilator-induced lung injury and decrease the risk of complications associated with prolonged mechanical ventilation.
Prone ventilation requires specialized training and expertise to perform safely and effectively. It necessitates close monitoring of the patient’s vital signs, airway, and pressure points to prevent complications such as pressure sores, endotracheal tube displacement, or hemodynamic instability. Additionally, patient sedation and paralysis may be required during the positioning procedure to ensure patient safety and tolerance. This technique is considered a valuable adjunct therapy in the management of severe respiratory failure and has demonstrated positive outcomes in selected patient populations.
Inhaled vasodilators may be used as supportive therapy in an attempt to increase perfusion and blood flow around the lungs to improve gas exchange. In severe cases, Extracorporeal Membrane Oxygenation (ECMO) may be indicated. For details on Inhaled Vasodilators and ECMO, please refer to specialized respiratory care textbooks, such as the latest edition of Egan’s Fundamentals of Respiratory Care.
“ESICM 2023 Recommendations for ARDS Ventilation” is adapted from ESICM guidelines on acute respiratory distress syndrome: definition, phenotyping and respiratory support strategies by Giacomo Grasselli, Carolyn S. Calfee, and Luigi Camporota from Intensive Care Medicine, Vol. 49, Issue 7 which is licensed under a Creative Commons Attribution-NonCommercial 4.0 International (CC BY-NC 4.0), except where otherwise noted.