6.4 Mars
Mars
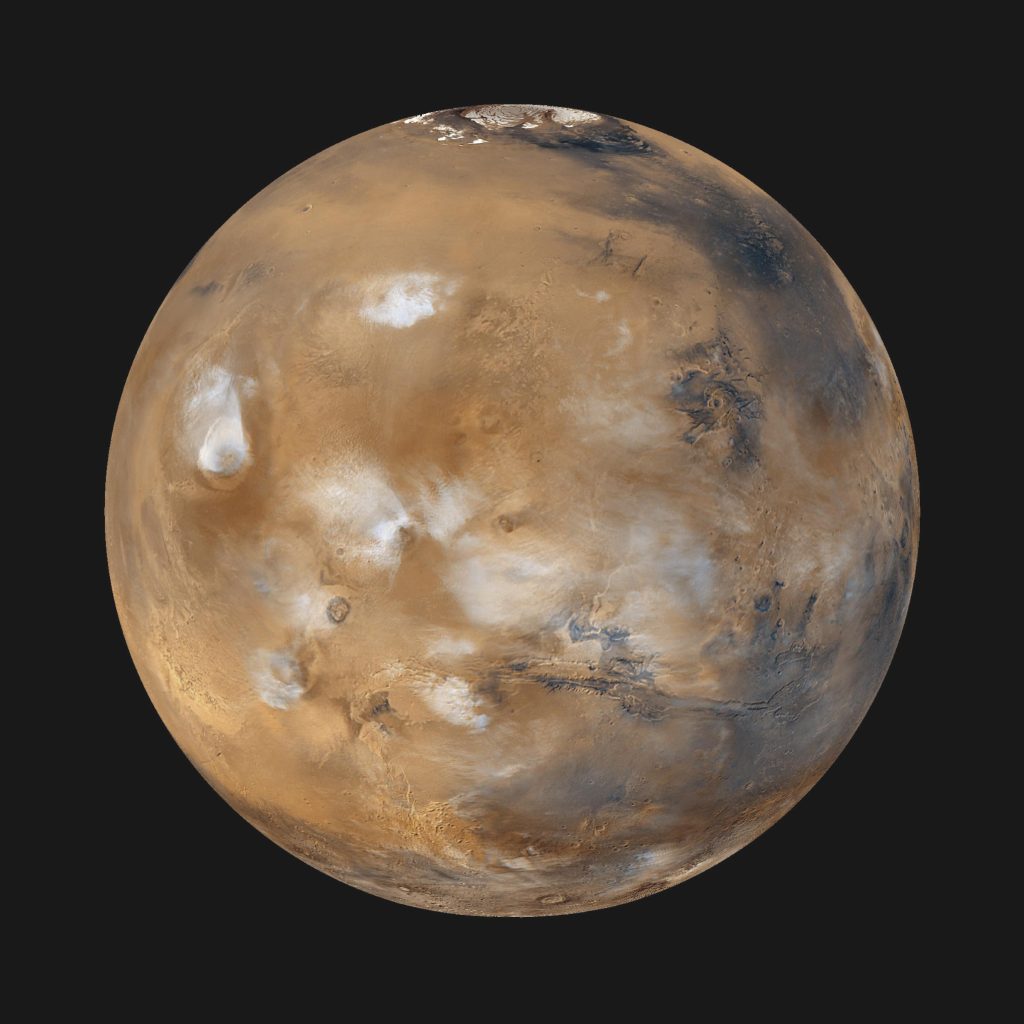
Mars by NASA/JPL/MSSS, NASA JPL Media Licence.
Mars is the fourth planet from the Sun. It is historically called the Red Planet because of its reddish appearance in Earth’s night sky. Mars has some Earth-type characteristics, such as Polar Caps, clouds, and water in at least the ice or solid phase. It was very active volcanically at one time. Numerous spacecraft have successfully explored Mars from orbit and the surface. It is by far the most-visited planet (by spacecraft) in our Solar System, besides Earth.
Astronomers have determined the rotation period of Mars with great accuracy by watching the motion of permanent surface markings; its sidereal day is 24 hours 37 minutes 23 seconds, just a little longer than the rotation period of Earth. This high precision is not obtained by watching Mars for a single rotation, but by noting how many turns it makes over a long period of time. Good observations of Mars date back more than 200 years, a period during which tens of thousands of martian days have passed. As a result, the rotation period can be calculated to within a few hundredths of a second.
The rotational axis of Mars has a tilt of about 25°, similar to the tilt of Earth’s axis. Thus, Mars experiences seasons very much like those on Earth. Because of the longer martian year (almost two Earth years), however, each season there lasts about six of our months.
Mars has a diameter of 6790 kilometres, just over half the diameter of Earth, giving it a total surface area very nearly equal to the continental (land) area of our planet. Its overall density of 3.9 g/cm3 suggests a composition consisting primarily of silicates but with a small metal core. The planet has no global magnetic field, although there are areas of strong surface magnetization that indicate that there was a global field billions of years ago. Apparently, the red planet has no liquid material in its core today that would conduct electricity.
Thanks to the Mars Global Surveyor, we have mapped the entire planet, as shown in Figure 6.11. A laser altimeter on board made millions of separate measurements of the surface topography to a precision of a few metres—good enough to show even the annual deposition and evaporation of the polar caps. Like Earth, the Moon, and Venus, the surface of Mars has continental or highland areas as well as widespread volcanic plains. The total range in elevation from the top of the highest mountain (Olympus Mons) to the bottom of the deepest basin (Hellas) is 31 kilometres.
Mars Map from Laser Ranging
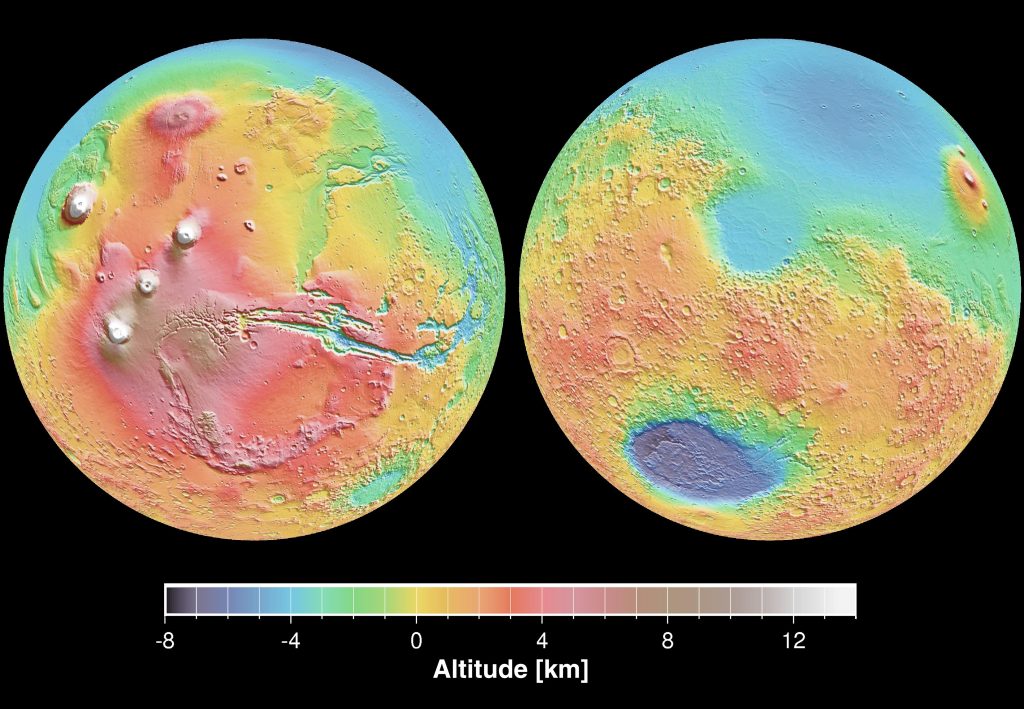
Mars Topography by NASA/JPL, NASA JPL Media Licence.
Approximately half the planet consists of heavily cratered highland terrain, found primarily in the southern hemisphere. The other half, which is mostly in the north, contains younger, lightly cratered volcanic plains at an average elevation about 5 kilometres lower than the highlands. Remember that we saw a similar pattern on Earth, the Moon, and Venus. A geological division into older highlands and younger lowland plains seems to be characteristic of all the terrestrial planets except Mercury.
Lying across the north-south division of Mars is an uplifted continent the size of North America. This is the 10-kilometer-high Tharsis bulge, a volcanic region crowned by four great volcanoes that rise still higher into the martian sky.
The lowland plains of Mars look very much like the lunar maria, and they have about the same density of impact craters. Like the lunar maria, they probably formed between 3 and 4 billion years ago. Apparently, Mars experienced extensive volcanic activity at about the same time the Moon did, producing similar basaltic lavas.
The largest volcanic mountains of Mars are found in the Tharsis area (you can see them in Figure 6.11), although smaller volcanoes dot much of the surface. The most dramatic volcano on Mars is Olympus Mons (Mount Olympus), with a diameter larger than 500 kilometres and a summit that towers more than 20 kilometres above the surrounding plains—three times higher than the tallest mountain on Earth (Figure 6.12). The volume of this immense volcano is nearly 100 times greater than that of Mauna Loa in Hawaii. Placed on Earth’s surface, Olympus would more than cover the entire state of Missouri.
Olympus Mons
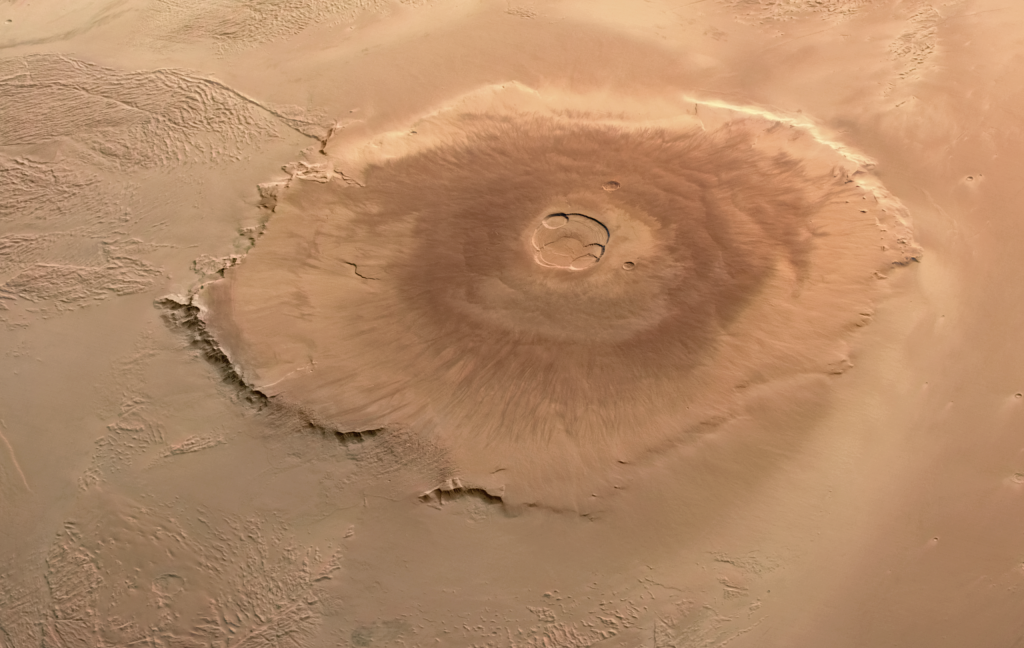
Olympus Mons – ESA Mars Express by ESA/DLR/FUBerlin/AndreaLuck, CC BY 2.0.
Images taken from orbit allow scientists to search for impact craters on the slopes of these volcanoes in order to estimate their age. Many of the volcanoes show a fair number of such craters, suggesting that they ceased activity a billion years or more ago. However, Olympus Mons has very, very few impact craters. Its present surface cannot be more than about 100 million years old; it may even be much younger. Some of the fresh-looking lava flows might have been formed a hundred years ago, or a thousand, or a million, but geologically speaking, they are quite young. This leads geologists to the conclusion that Olympus Mons possibly remains intermittently active today—something future Mars land developers may want to keep in mind.
The Tharsis bulge has many interesting geological features in addition to its huge volcanoes. In this part of the planet, the surface itself has bulged upward, forced by great pressures from below, resulting in extensive tectonic cracking of the crust. Among the most spectacular tectonic features on Mars are the canyons called the Valles Marineris (or Mariner Valleys, named after Mariner 9, which first revealed them to us), which are shown in Figure 6.13. They extend for about 5000 kilometres (nearly a quarter of the way around Mars) along the slopes of the Tharsis bulge. If it were on Earth, this canyon system would stretch all the way from Los Angeles to Washington, DC. The main canyon is about 7 kilometres deep and up to 100 kilometres wide, large enough for the Grand Canyon of the Colorado River to fit comfortably into one of its side canyons. Viewers of the movie “The Martian” can see a recreation of these canyonlands, as the film’s hero takes a long trip through a spectacular (and somewhat exaggerated) presentation of this part of Mars.
Heavily Eroded Canyonlands on Mars
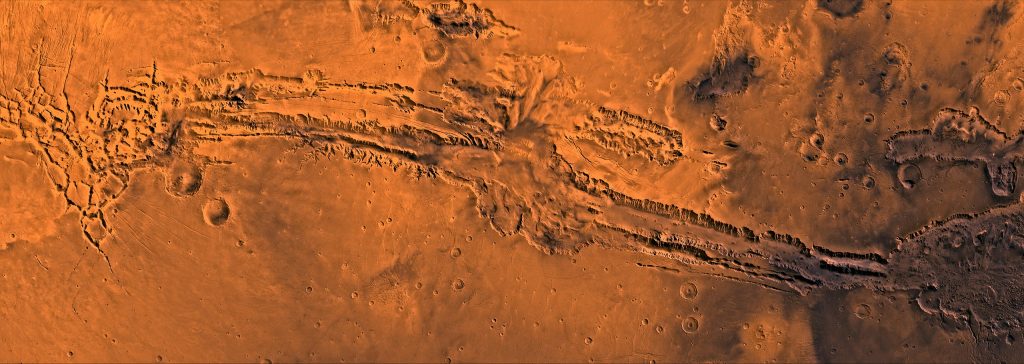
PIA00422: Valles Marineris by NASA/JPL/USGS, NASA JPL Media Licence.
The atmosphere of Mars today has an average surface pressure of only 0.007 bar, less than 1% that of Earth. (This is how thin the air is about 30 kilometres above Earth’s surface.) Martian air is composed primarily of carbon dioxide (95%), with about 3% nitrogen and 2% argon. The proportions of different gases are similar to those in the atmosphere of Venus, but a lot less of each gas is found in the thin air on Mars.
While winds on Mars can reach high speeds, they exert much less force than wind of the same velocity would on Earth because the atmosphere is so thin. The wind is able, however, to loft very fine dust particles, which can sometimes develop planet-wide dust storms. It is this fine dust that coats almost all the surface, giving Mars its distinctive red colour. In the absence of surface water, wind erosion plays a major role in sculpting the martian surface (Figure 6.14).
Wind Erosion on Mars
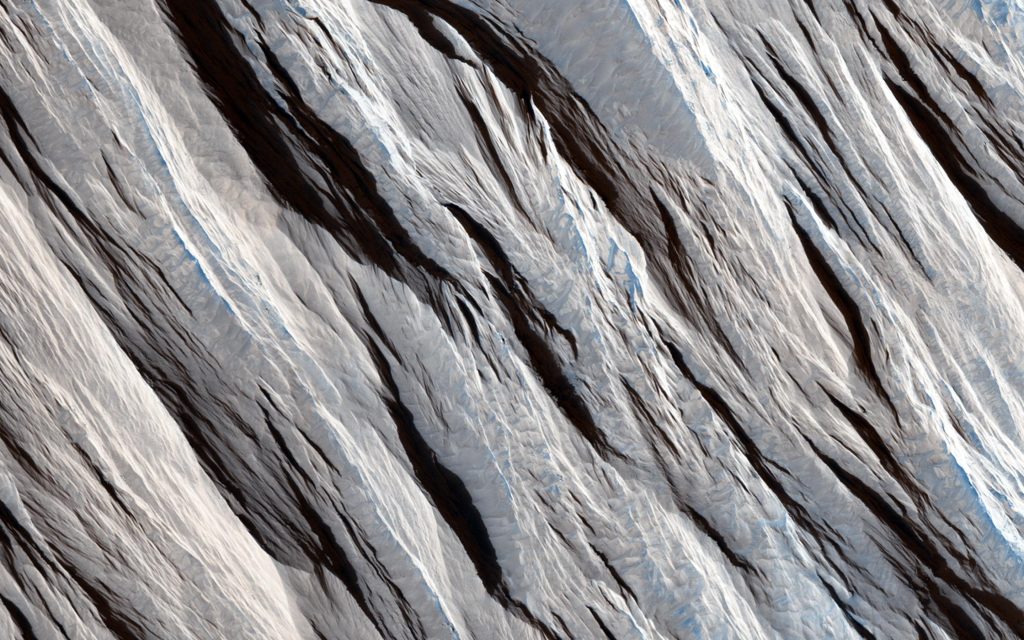
On the Beauty of Yardangs by NASA/JPL-Caltech/Univ. of Arizona, NASA JPL Media Licence.
The issue of how strong the winds on Mars can be plays a big role in the 2015 hit movie The Martian in which the main character is stranded on Mars after being buried in the sand in a windstorm so great that his fellow astronauts have to leave the planet so their ship is not damaged. Astronomers have noted that the martian winds could not possibly be as forceful as depicted in the film because the air pressure is so low. In most ways, however, the depiction of Mars in this movie is remarkably accurate.
Although the atmosphere contains small amounts of water vapor and occasional clouds of water ice, liquid water is not stable under present conditions on Mars. Part of the problem is the low temperatures on the planet. But even if the temperature on a sunny summer day rises above the freezing point, the low pressure means that liquid water still cannot exist on the surface, except at the lowest elevations. At a pressure of less than 0.006 bar, the boiling point is as low or lower than the freezing point, and water changes directly from solid to vapor without an intermediate liquid state (as does “dry ice,” carbon dioxide, on Earth). However, salts dissolved in water lower its freezing point, as we know from the way salt is used to thaw roads after snow and ice forms during winter on Earth. Salty water is therefore sometimes able to exist in liquid form on the martian surface, under the right conditions.
Several types of clouds can form in the martian atmosphere. First there are dust clouds, discussed above. Second are water-ice clouds similar to those on Earth. These often form around mountains, just as happens on our planet. Finally, the CO2 of the atmosphere can itself condense at high altitudes to form hazes of dry ice crystals. The CO2 clouds have no counterpart on Earth, since on our planet temperatures never drop low enough (down to about 150 K or about –125 °C) for this gas to condense.
Through a telescope, the most prominent surface features on Mars are the bright polar caps, which change with the seasons, similar to the seasonal snow cover on Earth. We do not usually think of the winter snow in northern latitudes as a part of our polar caps, but seen from space, the thin winter snow merges with Earth’s thick, permanent ice caps to create an impression much like that seen on Mars (Figure 6.15).
Martian North Polar Cap
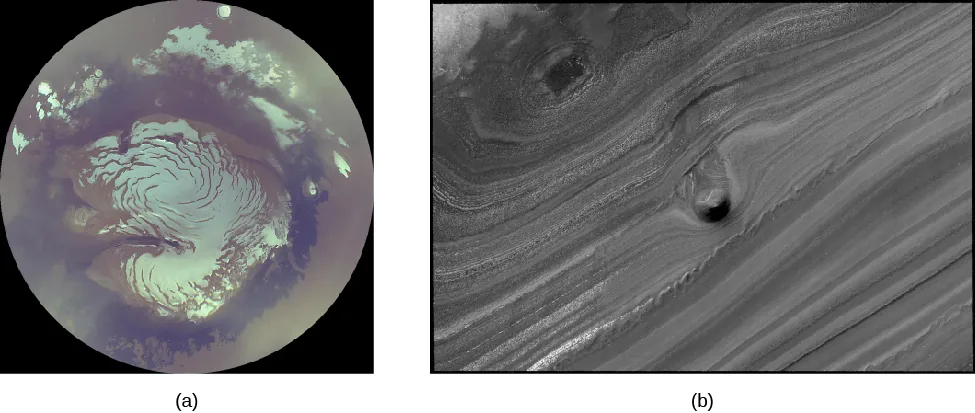
(a): PIA01928: Mars Polar Cap During Transition Phase Instrument Checkout by NASA/JPL/MSSS, NASA Media Licence.
(b): PIA11231: Unusual Mound in North Polar Layered Deposits by NASA/JPL-Caltech/University of Arizona, NASA Media Licence.
The seasonal caps on Mars are composed not of ordinary snow but of frozen CO2 (dry ice). These deposits condense directly from the atmosphere when the surface temperature drops below about 150 K. The caps develop during the cold martian winters and extend down to about 50° latitude by the start of spring.
Quite distinct from these thin seasonal caps of CO2 are the permanent or residual caps that are always present near the poles. The southern permanent cap has a diameter of 350 kilometres and is composed of frozen CO2 deposits together with a great deal of water ice. Throughout the southern summer, it remains at the freezing point of CO2, 150 K, and this cold reservoir is thick enough to survive the summer heat intact.
The northern permanent cap is different. It is much larger, never shrinking to a diameter less than 1000 kilometres, and is composed of water ice. Summer temperatures in the north are too high for the frozen CO2 to be retained. Measurements from the Mars Global Surveyor have established the exact elevations in the north polar region of Mars, showing that it is a large basin about the size of our own Arctic Ocean basin. The ice cap itself is about 3 kilometres thick, with a total volume of about 10 million km3 (similar to that of Earth’s Mediterranean Sea). If Mars ever had extensive liquid water, this north polar basin would have contained a shallow sea. There is some indication of ancient shorelines visible, but better images will be required to verify this suggestion.
Images taken from orbit also show a distinctive type of terrain surrounding the permanent polar caps, as shown in Figure 6.15. At latitudes above 80° in both hemispheres, the surface consists of recent layered deposits that cover the older cratered ground below. Individual layers are typically ten to a few tens of metres thick, marked by alternating light and dark bands of sediment. Probably the material in the polar deposits includes dust carried by wind from the equatorial regions of Mars.
What do these terraced layers tell us about Mars? Some cyclic process is depositing dust and ice over periods of time. The time scales represented by the polar layers are tens of thousands of years. Apparently the martian climate experiences periodic changes at intervals similar to those between ice ages on Earth. Calculations indicate that the causes are probably also similar: the gravitational pull of the other planets produces variations in Mars’ orbit and tilt as the great clockwork of the solar system goes through its paces.
The Phoenix spacecraft landed near the north polar cap in summer (Figure 6.16). Controllers knew that it would not be able to survive a polar winter, but directly measuring the characteristics of the polar region was deemed important enough to send a dedicated mission. The most exciting discovery came when the spacecraft tried to dig a shallow trench under the spacecraft. When the overlying dust was stripped off, they saw bright white material, apparently some kind of ice. From the way this ice sublimated over the next few days, it was clear that it was frozen water.
Evaporating Ice on Mars
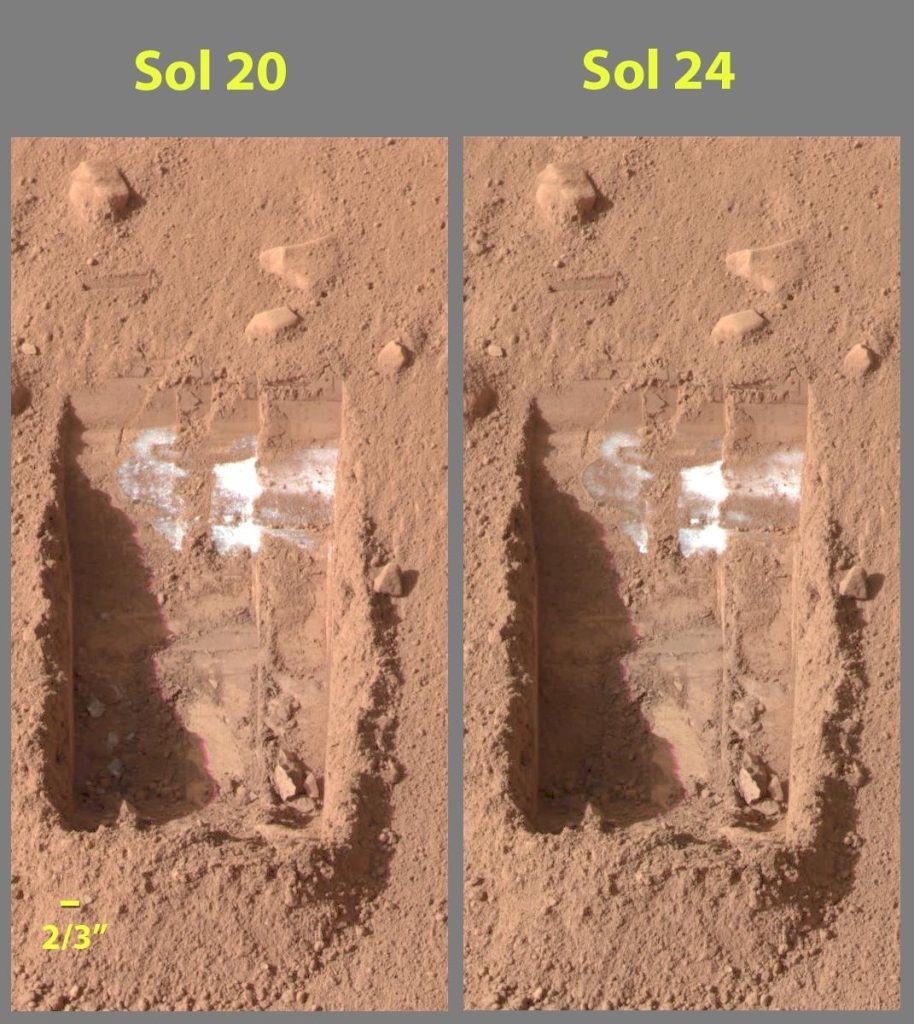
Disappearing Ice In Colour by NASA/JPL-Caltech/University of Arizona/Texas A&M University, NASA Media Licence.
Example 6.1
Comparing the Amount of Water on Mars and Earth
It is interesting to estimate the amount of water (in the form of ice) on Mars and to compare this with the amount of water on Earth. In each case, we can find the total volume of a layer on a sphere by multiplying the area of the sphere ([latex]4\pi R^2[/latex]) by the thickness of the layer. For Earth, the ocean water is equivalent to a layer [latex]3[/latex] km thick spread over the entire planet, and the radius of Earth is [latex]6.378\times 10^6[/latex] m.
For Mars, most of the water we are sure of is in the form of ice near the poles. We can calculate the amount of ice in one of the residual polar caps if it is (for example) [latex]2[/latex] km thick and has a radius of [latex]400[/latex] km (the area of a circle is [latex]\pi R^2[/latex]).
Solution
The volume of Earth’s water is therefore the area
[latex]\begin{align*}4\pi R^2&=4\pi(6.378\times 10^6)^2\\&=5.1\times 10^14 m^2\end{align*}[/latex]
multiplied by the thickness of [latex]3000\;\text{m}[/latex]:
[latex]\begin{align*}5.1\times 10^14\;\text{m}^2\times 3000\;\text{m} = 1.5\times 10^18\;\text{m}^3\end{align*}[/latex]
This gives [latex]1.5\times 10^18 m^3[/latex] of water. Since water has a density of [latex]1[/latex] ton per cubic meter ([latex]1000\;\text{kg/m}^3[/latex]), we can calculate the mass:
[latex]\begin{align*}1.5\times 10^18 m^3\times 1\;\text{ton/m}^3=1.5\times 10^18\;\text{tons}\end{align*}[/latex]
For Mars, the ice doesn’t cover the whole planet, only the caps; the polar cap area is
[latex]\pi R^2=\pi(4\times 10^5\;\text{m})^2=5\times 10^11\;\text{m}^2[/latex]
(Note that we converted kilometres to metres.)
The volume = area × height, so we have:
[latex](2\times 10^3\;\text{m})(5\times 10^11\;\text{m}^2)=1\times 10^15\;\text{m}^3=10^15\;\text{m}^3[/latex]
Therefore, the mass is:
[latex]10^15\;\text{m}^3\times 1\;\text{ton/m}^3=10^15\;\text{tons}[/latex]
This is about [latex]0.1\%[/latex] that of Earth’s oceans.
Exercise 6.1
A better comparison might be to compare the amount of ice in the Mars polar ice caps to the amount of ice in the Greenland ice sheet on Earth, which has been estimated as [latex]2.85\times 10^15\;\text{m}^3[/latex]. How does this compare with the ice on Mars?
Solution
The Greenland ice sheet has about [latex]2.85[/latex] times as much ice as in the polar ice caps on Mars. They are about the same to the nearest power of [latex]10[/latex].
Although no bodies of liquid water exist on Mars today, evidence has accumulated that rivers flowed on the red planet long ago. Two kinds of geological features appear to be remnants of ancient watercourses, while a third class—smaller gullies—suggests intermittent outbreaks of liquid water even today. We will examine each of these features in turn.
In the highland equatorial plains, there are multitudes of small, sinuous (twisting) channels—typically a few metres deep, some tens of metres wide, and perhaps 10 or 20 kilometres long (Figure 6.17). They are called runoff channels because they look like what geologists would expect from the surface runoff of ancient rain storms. These runoff channels seem to be telling us that the planet had a very different climate long ago. To estimate the age of these channels, we look at the cratering record. Crater counts show that this part of the planet is more cratered than the lunar maria but less cratered than the lunar highlands. Thus, the runoff channels are probably older than the lunar maria, presumably about 4 billion years old.
The second set of water-related features we see are outflow channels (Figure 6.17) are much larger than the runoff channels. The largest of these, which drain into the Chryse basin where Pathfinder landed, are 10 kilometres or more wide and hundreds of kilometres long. Many features of these outflow channels have convinced geologists that they were carved by huge volumes of running water, far too great to be produced by ordinary rainfall. Where could such floodwater have come from on Mars?
Runoff and Outflow Channels

(a): Photo No. A85-0760-3 by NASA, NASA Media Licence.
(b): PIA02094: Water: Sustained Flow by NASA/JPL/MSSS, NASA Media Licence.
As far we can tell, the regions where the outflow channels originate contained abundant water frozen in the soil as permafrost. Some local source of heating must have released this water, leading to a period of rapid and catastrophic flooding. Perhaps this heating was associated with the formation of the volcanic plains on Mars, which date back to roughly the same time as the outflow channels.
Note that neither the runoff channels nor the outflow channels are wide enough to be visible from Earth, nor do they follow straight lines. They could not have been the “canals” Percival Lowell imagined seeing on the red planet.
The third type of water feature, the smaller gullies, was discovered by the Mars Global Surveyor (Figure 6.18). The Mars Global Surveyor’s camera images achieved a resolution of a few metres, good enough to see something as small as a truck or bus on the surface. On the steep walls of valleys and craters at high latitudes, there are many erosional features that look like gullies carved by flowing water. These gullies are very young: not only are there no superimposed impact craters, but in some instances, the gullies seem to cut across recent wind-deposited dunes. Perhaps there is liquid water underground that can occasionally break out to produce short-lived surface flows before the water can freeze or evaporate.
Gullies on the Wall of Garni Crater
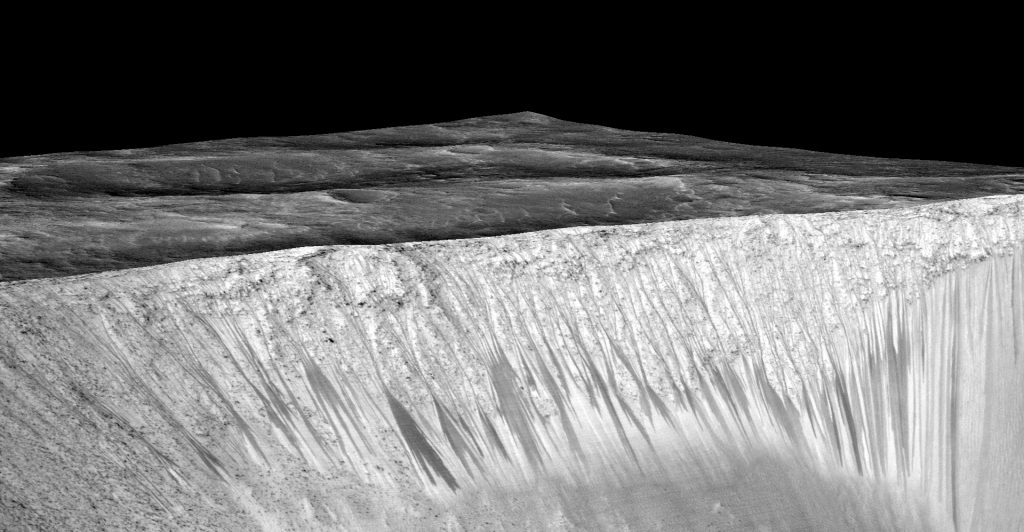
Dark, Recurring Streaks on Walls of Garni Crater on Mars by NASA/JPL-Caltech/Univ. of Arizona, NASA Media Licence.
The gullies also have the remarkable property of changing regularly with the martian seasons. Many of the dark streaks (visible in Figure 6.18) elongate within a period of a few days, indicating that something is flowing downhill—either water or dark sediment. If it is water, it requires a continuing source, either from the atmosphere or from springs that tap underground water layers (aquifers.) Underground water would be the most exciting possibility, but this explanation seems inconsistent with the fact that many of the dark streaks start at high elevations on the walls of craters.
Additional evidence that the dark streaks (called by the scientists recurring slope lineae) are caused by water was found in 2015 when spectra were obtained of the dark streaks (Figure 6.19). These showed the presence of hydrated salts produced by the evaporation of salty water. If the water is salty, it could remain liquid long enough to flow downstream for distances of a hundred metres or more, before it either evaporates or soaks into the ground. However, this discovery still does not identify the ultimate source of the water.
Evidence for Liquid Water on Mars
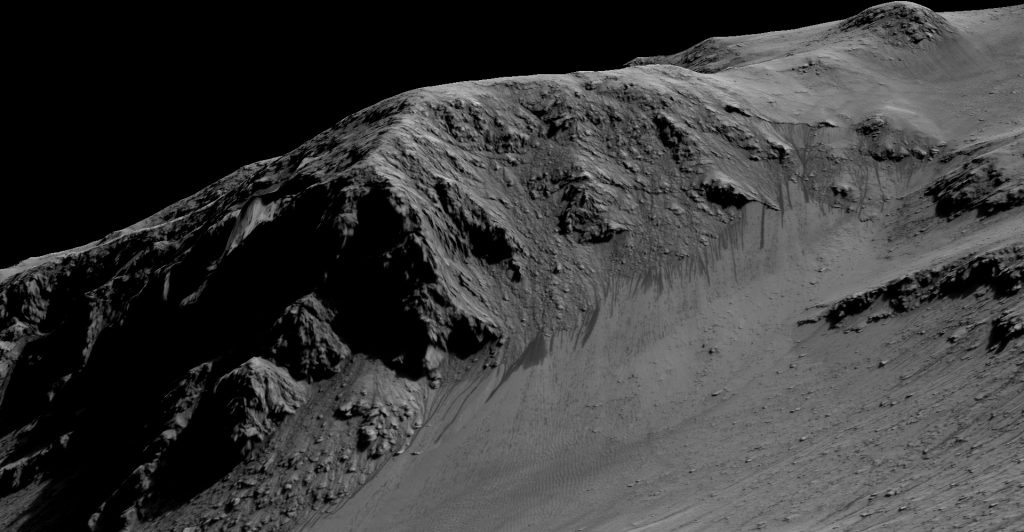
Recurring “Lineae” on Slopes at Horowitz Crater by NASA/JPL-Caltech/Univ. of Arizona, NASA Media Licence.
Deimos
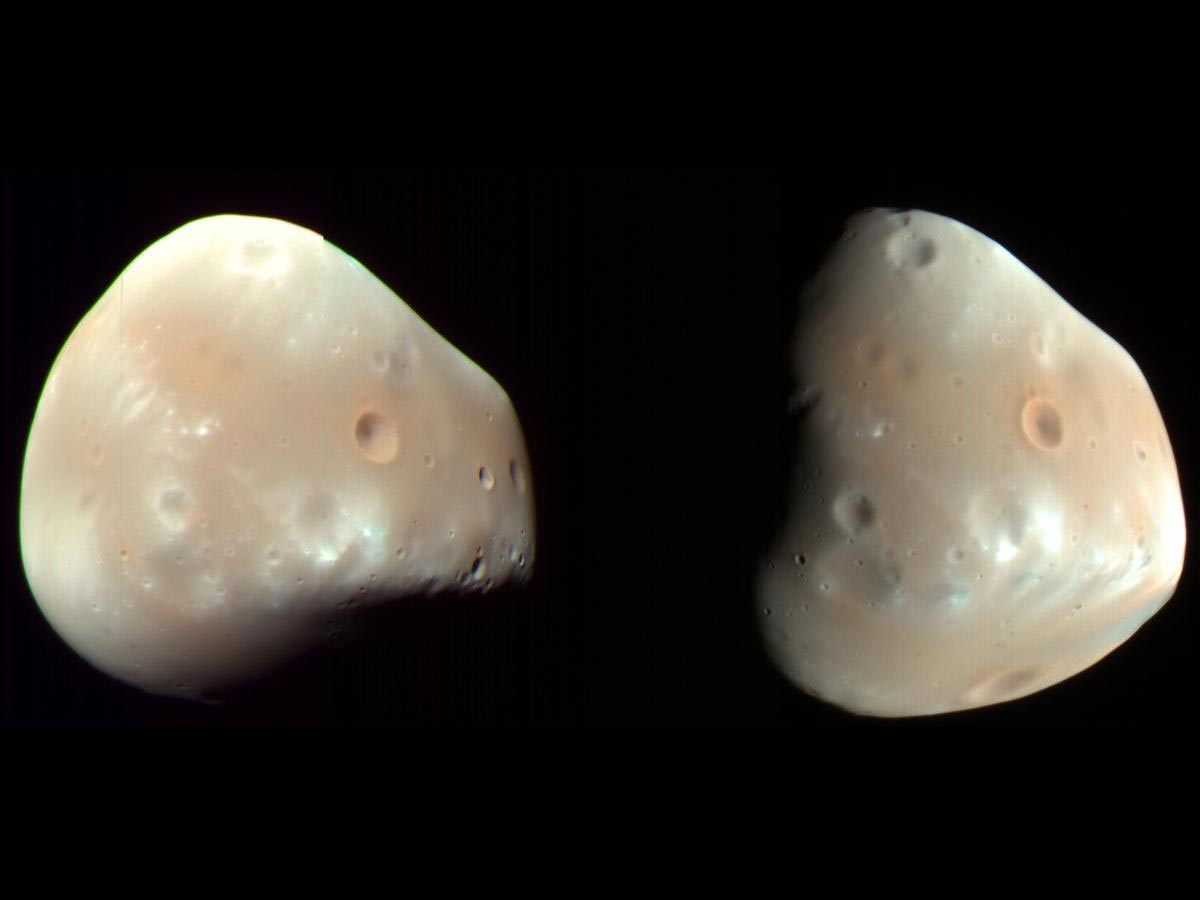
Colour-enhanced views of Deimos by NASA/JPL-Caltech/Univ. of Arizona, NASA Media Licence.
Phobos
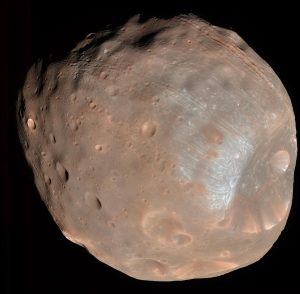
Colour-enhanced views of Phobos by NASA/JPL-Caltech/Univ. of Arizona, NASA Media Licence.
Attribution
“10.1 The Nearest Planets: An Overview“, “10.4 The Geology of Mars“, and “10.5 Water and Life on Mars” from Astronomy 2e by Andrew Fraknoi, David Morrison, Sidney C. Wolff, © OpenStax – Rice University is licensed under a Creative Commons Attribution 4.0 International License, except where otherwise noted.
“Planet Mars” and “Martian Moons” from Introduction to Astronomy by Lumen Learning is licensed under a Creative Commons Attribution 4.0 International License, except where otherwise noted.