5.1 An Inventory of the Solar System
The solar system consists of the Sun and many smaller objects: the planets, their moons and rings, and such “debris” as asteroids, comets, and dust. Decades of observation and spacecraft exploration have revealed that most of these objects formed together with the Sun about 4.5 billion years ago. They represent clumps of material that condensed from an enormous cloud of gas and dust. The central part of this cloud became the Sun, and a small fraction of the material in the outer parts eventually formed the other objects.
During the past 50 years, we have learned more about the solar system than anyone imagined before the space age. In addition to gathering information with powerful new telescopes, we have sent spacecraft directly to many members of the planetary system. (Planetary astronomy is the only branch of our science in which we can, at least vicariously, travel to the objects we want to study.) With evocative names such as Voyager, Pioneer, Curiosity, and Pathfinder, our robot explorers have flown past, orbited, or landed on every planet, returning images and data that have dazzled both astronomers and the public. In the process, we have also investigated two dwarf planets, hundreds of fascinating moons, four ring systems, a dozen asteroids, and several comets (smaller members of our solar system that we will discuss later).
Our probes have penetrated the atmosphere of Jupiter and landed on the surfaces of Venus, Mars, our Moon, Saturn’s moon Titan, the asteroids Eros and Itokawa, and the Comet Churyumov-Gerasimenko (usually referred to as 67P). Humans have set foot on the Moon and returned samples of its surface soil for laboratory analysis as shown in Figure 5.1. We have even discovered other places in our solar system that might be able to support some kind of life.
Astronauts on the Moon
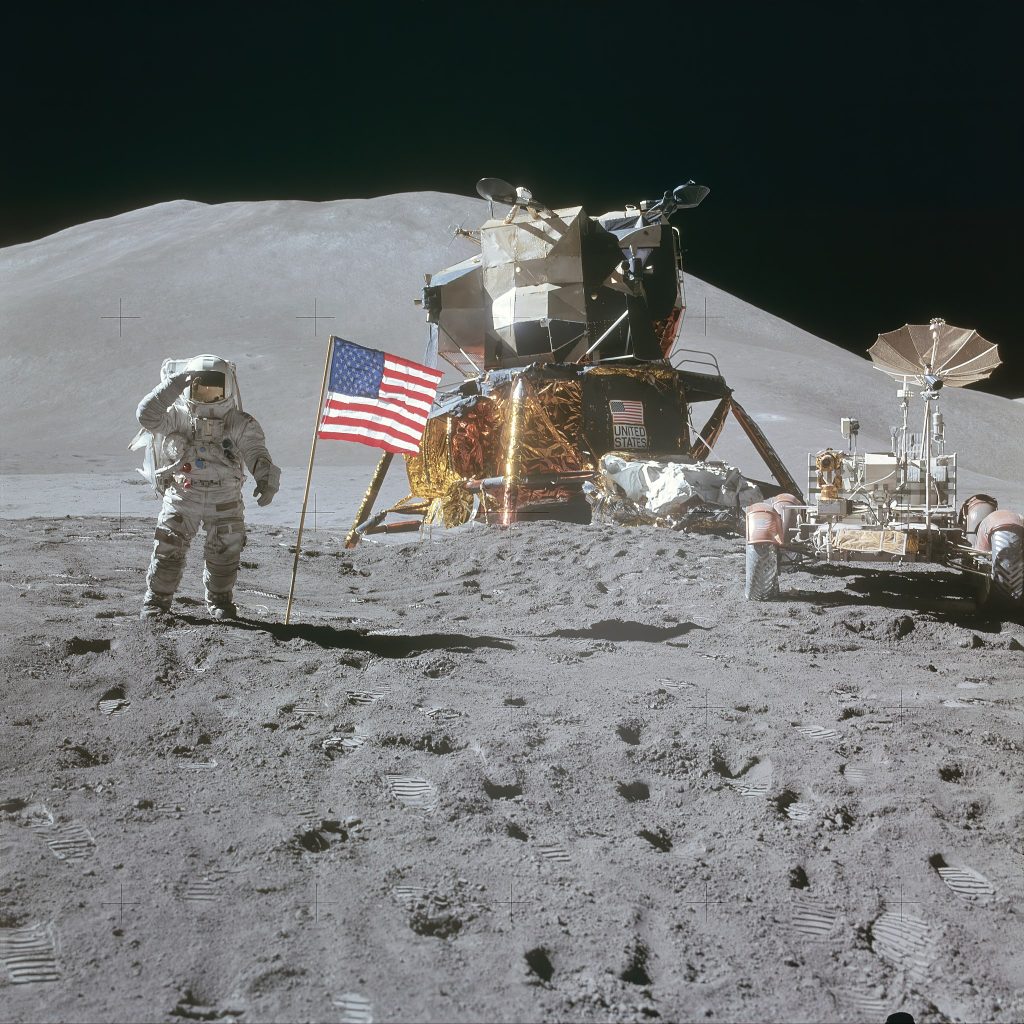
Apollo 15 flag, rover, LM, Irwin by NASA/David R. Scott, modified by Bammesk, NASA Media Licence.
An Inventory
The Sun, a star that is brighter than about 80% of the stars in the Galaxy, is by far the most massive member of the solar system, as shown in Table 5.1. It is an enormous ball about 1.4 million kilometres in diameter, with surface layers of incandescent gas and an interior temperature of millions of degrees. The Sun will be discussed in later chapters as our first, and best-studied, example of a star.
Object | Percentage of Total Mass of Solar System |
---|---|
Sun | 99.80 |
Jupiter | 0.10 |
Comets | 0.0005–0.03 (estimate) |
All other planets and dwarf planets | 0.04 |
Moons and rings | 0.00005 |
Asteroids | 0.000002 (estimate) |
Cosmic dust | 0.0000001 (estimate) |
Table 5.1 also shows that most of the material of the planets is actually concentrated in the largest one, Jupiter, which is more massive than all the rest of the planets combined. Astronomers were able to determine the masses of the planets centuries ago using Kepler’s laws of planetary motion and Newton’s law of gravity to measure the planets’ gravitational effects on one another or on moons that orbit them. Today, we make even more precise measurements of their masses by tracking their gravitational effects on the motion of spacecraft that pass near them.
Beside Earth, five other planets were known to the ancients—Mercury, Venus, Mars, Jupiter, and Saturn—and two were discovered after the invention of the telescope: Uranus and Neptune. The eight planets all revolve in the same direction around the Sun. They orbit in approximately the same plane, like cars travelling on concentric tracks on a giant, flat racecourse. Each planet stays in its own “traffic lane,” following a nearly circular orbit about the Sun and obeying the “traffic” laws discovered by Galileo, Kepler, and Newton. Besides these planets, we have also been discovering smaller worlds beyond Neptune that are called trans-Neptunian objects or TNOs as shown in Figure 5.2. The first to be found, in 1930, was Pluto, but others have been discovered during the twenty-first century. One of them, Eris, is about the same size as Pluto and has at least one moon (Pluto has five known moons.) The largest TNOs are also classed as dwarf planets, as is the largest asteroid, Ceres. To date, more than 1750 of these TNOs have been discovered.
Each of the planets and dwarf planets also rotates (spins) about an axis running through it, and in most cases the direction of rotation is the same as the direction of revolution about the Sun. The exceptions are Venus, which rotates backward very slowly (that is, in a retrograde direction), and Uranus and Pluto, which also have strange rotations, each spinning about an axis tipped nearly on its side. We do not yet know the spin orientations of Eris, Haumea, and Makemake.
The four planets closest to the Sun (Mercury through Mars) are called the inner or terrestrial planets. Often, the Moon is also discussed as a part of this group, bringing the total of terrestrial objects to five. (We generally call Earth’s satellite “the Moon,” with a capital M, and the other satellites “moons,” with lowercase m’s.) The terrestrial planets are relatively small worlds, composed primarily of rock and metal. All of them have solid surfaces that bear the records of their geological history in the forms of craters, mountains, and volcanoes as shown in Figure 5.3.
Surface of Mercury
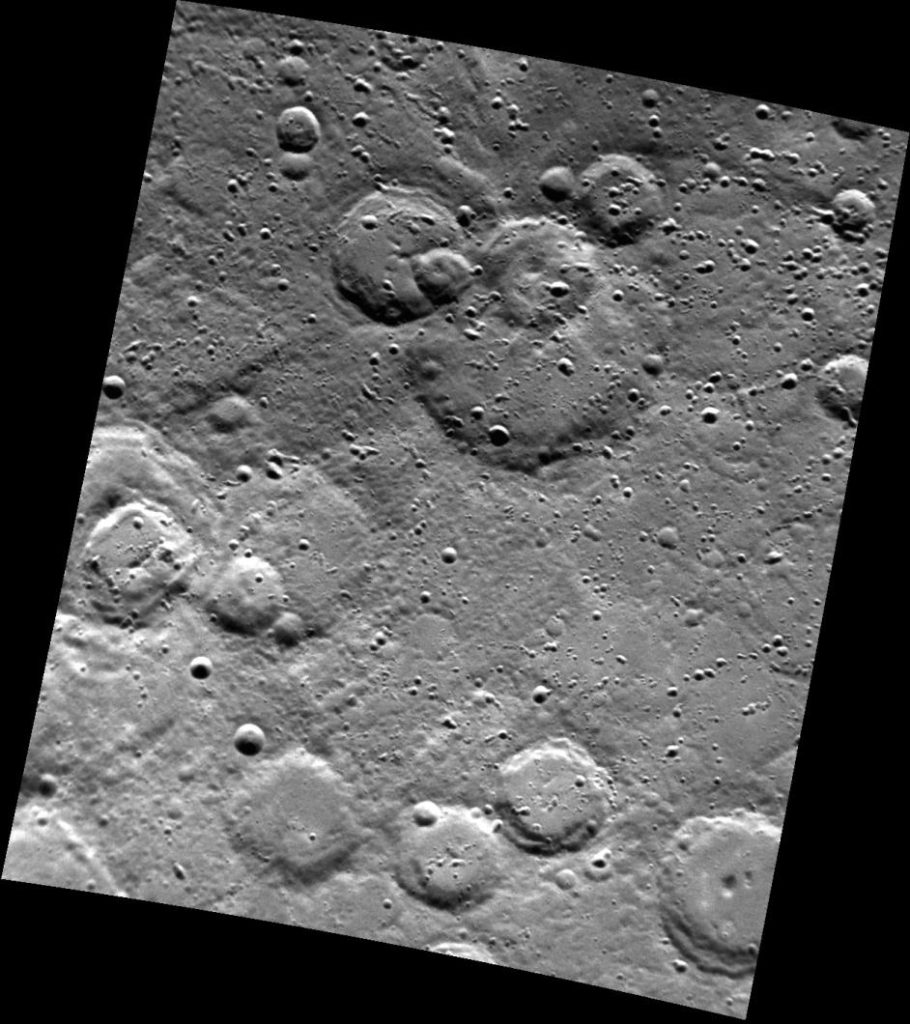
PIA16757 by NASA/John Hopkins University Applied Physics Laboratory/Carnegie Institution of Washington, JPL Media Licence.
The next four planets (Jupiter through Neptune) are much larger and are composed primarily of lighter ices, liquids, and gases. We call these four the jovian planets (after “Jove,” another name for Jupiter in mythology) or giant planets—a name they richly deserve. They are shown in Figure 5.4. More than 1400 Earths could fit inside Jupiter, for example. These planets do not have solid surfaces on which future explorers might land. They are more like vast, spherical oceans with much smaller, dense cores.
The Four Giant Planets

First and Farthest by NASA, Solar System Exploration, NASA Media Licence.
Near the outer edge of the system lies Pluto, which was the first of the distant icy worlds to be discovered beyond Neptune (Pluto was visited by a spacecraft, the NASA New Horizons mission, in 2015. Figure 5.5 is the image it captured during its visit). Table 5.2 summarizes some of the main facts about the planets.
Pluto Close-up
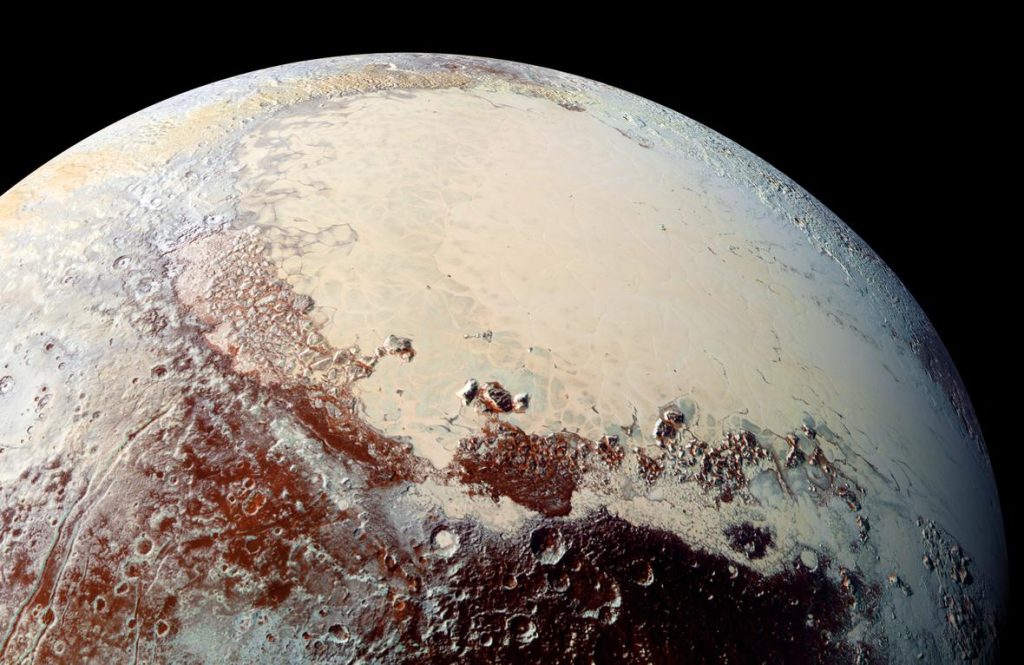
Sputnik Planum, in Color by NASA/Johns Hopkins University Applied Physics Laboratory/Southwest Research Institute, JPL Media Licence.
Name | Distance from Sun | Revolution Period | Diameter | Mass | Density |
---|---|---|---|---|---|
(AU) | (y) | (km) | (1023 kg) | (g/cm3) | |
Mercury | 0.39 | 0.24 | 4,878 | 3.3 | 5.4 |
Venus | 0.72 | 0.62 | 12,120 | 48.7 | 5.2 |
Earth | 1.00 | 1.00 | 12,756 | 59.8 | 5.5 |
Mars | 1.52 | 1.88 | 6,787 | 6.4 | 3.9 |
Jupiter | 5.20 | 11.86 | 142,984 | 18,991 | 1.3 |
Saturn | 9.54 | 29.46 | 120,536 | 5686 | 0.7 |
Uranus | 19.18 | 84.07 | 51,118 | 866 | 1.3 |
Neptune | 30.06 | 164.82 | 49,660 | 1030 | 1.6 |
Example 5.1
Comparing Densities
Let’s compare the densities of several members of the solar system. The density of an object equals its mass divided by its volume. The volume ([latex]V[/latex]) of a sphere (like a planet) is calculated using the equation
where [latex]\pi[/latex] (the Greek letter pi) has a value of approximately [latex]3.14[/latex]. Although planets are not perfect spheres, this equation works well enough. The masses and diametres of the planets are given in Table 5.2. Let’s use Saturn’s moon Mimas as our example, with a mass of [latex]4\times 10^19[/latex] kg and a diameter of approximately [latex]400[/latex] km (radius, [latex]200\;\text{km}= 2\times 10^5\;\text{m}[/latex]).
Solution
The volume of Mimas is
Density is mass divided by volume:
Note that the density of water in these units is 1000 kg/m3, so Mimas must be made mainly of ice, not rock.
Exercise 5.1
Calculate the average density of our own planet, Earth. Show your work. How does it compare to the density of an ice moon like Mimas? See Table 5.2 for data.
Solution
For a sphere,
[latex]\begin{align*}\text{density}=\frac{\text{mass}}{\left(\frac{4}{3}\pi\:R^{3}\right)}{\text{kg/m}}^{3}\end{align*}[/latex]
For Earth, then,
[latex]\begin{align*}\text{density}=\frac{6\phantom{\rule{0.2em}{0ex}}\times\phantom{\rule{0.2em}{0ex}}{10}^{24}\phantom{\rule{0.2em}{0ex}}\text{kg}}{4.2\phantom{\rule{0.2em}{0ex}}\times\phantom{\rule{0.2em}{0ex}}2.6\phantom{\rule{0.2em}{0ex}}\times\phantom{\rule{0.2em}{0ex}}{10}^{20}\phantom{\rule{0.2em}{0ex}}{\text{m}}^{3}}=5.5\phantom{\rule{0.2em}{0ex}}\times\phantom{\rule{0.2em}{0ex}}{10}^{3}\phantom{\rule{0.2em}{0ex}}{\text{kg/m}}^{3}\end{align*}[/latex]
This density is four to five times greater than Mimas’. In fact, Earth is the densest of the planets.
Attribution
“7.1 Overview of Our Planetary System” from Douglas College Astronomy 1105 by Douglas College Department of Physics and Astronomy, is licensed under a Creative Commons Attribution 4.0 International License, except where otherwise noted. Adapted from Astronomy 2e.