6.3 The Moon: Surface, Interior, and Origin
The Moon has only one-eightieth the mass of Earth and about one-sixth Earth’s surface gravity—too low to retain an atmosphere. The Moon may be seen in Figure 6.3. Moving molecules of a gas can escape from a planet just the way a rocket does, and the lower the gravity, the easier it is for the gas to leak away into space. While the Moon can acquire a temporary atmosphere from impacting comets, this atmosphere is quickly lost by freezing onto the surface or by escape to surrounding space. The Moon today is dramatically deficient in a wide range of volatiles, those elements and compounds that evaporate at relatively low temperatures. Some of the Moon’s properties are summarized in Table 6.1, along with comparative values for Mercury.
Two Sides of the Moon
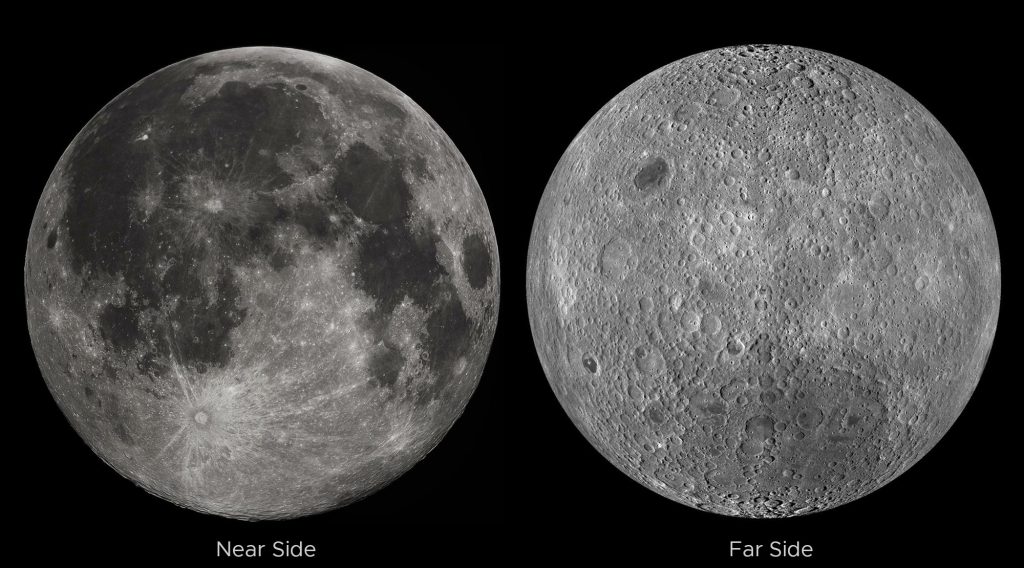
The Moon’s near side and far side. by NASA LRO / Jatan Mehta , NASA Media Licence.
Property | Moon | Mercury |
---|---|---|
Mass (Earth = 1) | 0.0123 | 0.055 |
Diameter (km) | 3476 | 4878 |
Density (g/cm3) | 3.3 | 5.4 |
Surface gravity (Earth = 1) | 0.17 | 0.38 |
Escape velocity (km/s) | 2.4 | 4.3 |
Rotation period (days) | 27.3 | 58.65 |
Surface area (Earth = 1) | 0.27 | 0.38 |
If you look at the Moon through a telescope, you can see that it is covered by impact craters of all sizes. The most conspicuous of the Moon’s surface features—those that can be seen with the unaided eye and that make up the feature often called “the man in the Moon”—are vast splotches of darker lava flows.
Centuries ago, early lunar observers thought that the Moon had continents and oceans and that it was a possible abode of life. They called the dark areas “seas” (maria in Latin, or mare in the singular, pronounced “mah ray”). Their names, Mare Nubium (Sea of Clouds), Mare Tranquillitatis (Sea of Tranquility), and so on, are still in use today. In contrast, the “land” areas between the seas are not named. Thousands of individual craters have been named, however, mostly for great scientists and philosophers such as the one in Figure 6.4. Among the most prominent craters are those named for Plato, Copernicus, Tycho, and Kepler. Galileo only has a small crater, however, reflecting his low standing among the Vatican scientists who made some of the first lunar maps.
We know today that the resemblance of lunar features to terrestrial ones is superficial. Even when they look somewhat similar, the origins of lunar features such as craters and mountains are very different from their terrestrial counterparts. The Moon’s relative lack of internal activity, together with the absence of air and water, make most of its geological history unlike anything we know on Earth.
Sunrise on the Central Mountain Peaks of Tycho Crater, as Imaged by the NASA Lunar Reconnaissance Orbiter.
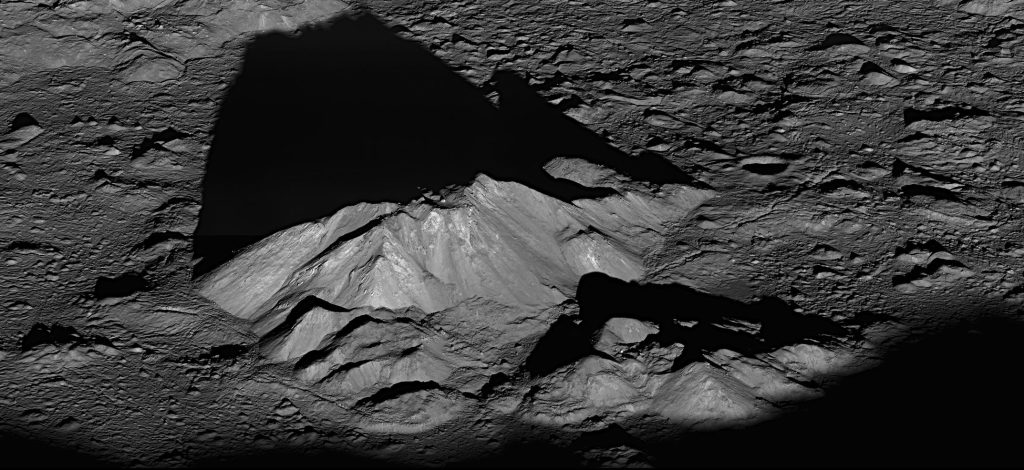
Tycho Crater’s Central Peak on the Moon by NASA Goddard/Arizona State University, NASA Media Licence.
Lunar History
To trace the detailed history of the Moon or of any planet, we must be able to estimate the ages of individual rocks. Once lunar samples were brought back by the Apollo astronauts, the radioactive dating techniques that had been developed for Earth were applied to them. The solidification ages of the samples ranged from about 3.3 to 4.4 billion years old, substantially older than most of the rocks on Earth.
Most of the crust of the Moon (83%) consists of silicate rocks called anorthosites; these regions are known as the lunar highlands. They are made of relatively low-density rock that solidified on the cooling Moon like slag floating on the top of a smelter. Because they formed so early in lunar history (between 4.1 and 4.4 billion years ago), the highlands are also extremely heavily cratered, bearing the scars of all those billions of years of impacts by interplanetary debris as shown in Figure 6.5.
Lunar Highlands
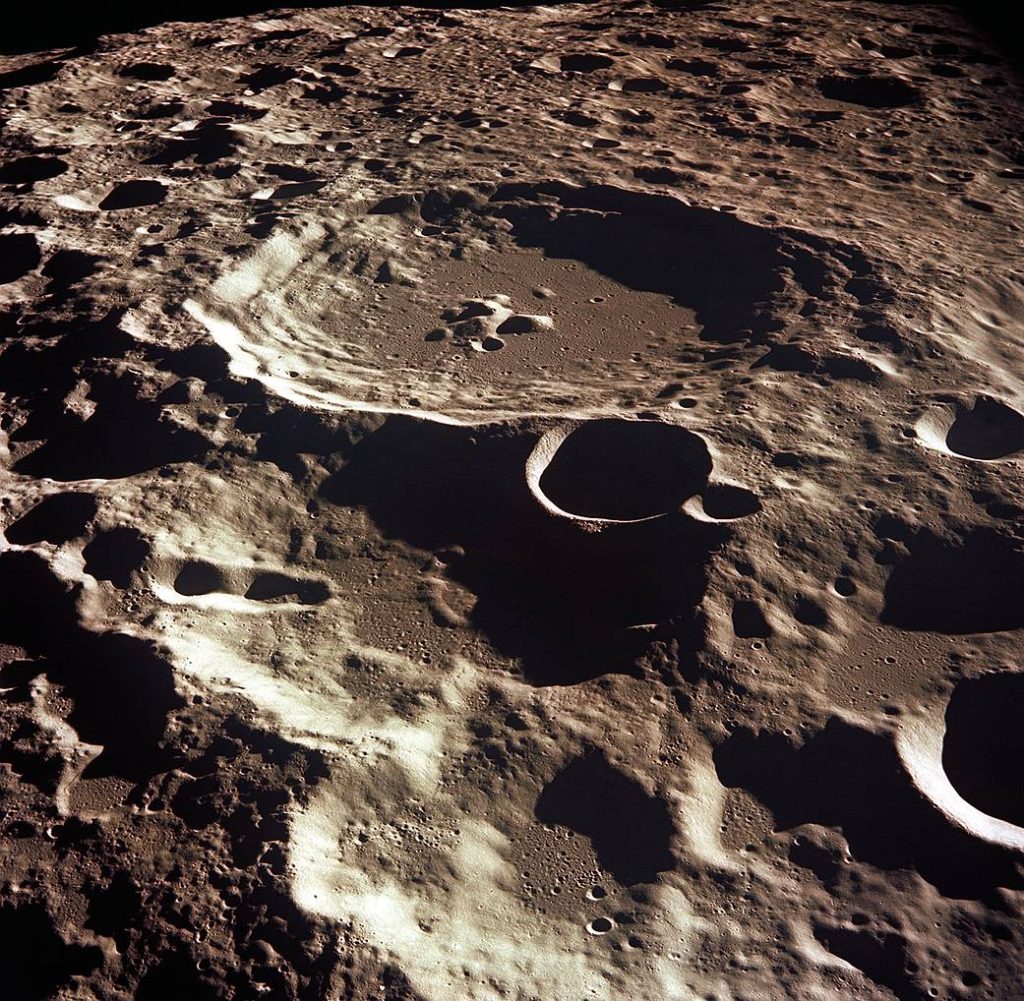
Crater Daedalus by NASA Goddard/Arizona State University, NASA Media Licence.
Unlike the mountains on Earth, the Moon’s highlands do not have any sharp folds in their ranges. The highlands have low, rounded profiles that resemble the oldest, most eroded mountains on Earth as shown in Figure 6.6. Because there is no atmosphere or water on the Moon, there has been no wind, water, or ice to carve them into cliffs and sharp peaks, the way we have seen them shaped on Earth. Their smooth features are attributed to gradual erosion, mostly due to impact cratering from meteorites.
Lunar Mountain

Apollo 15 Mt. Hadley Delta by NASA/Apollo 15, NASA Media Licence.
The maria are much less cratered than the highlands, and cover just 17% of the lunar surface, mostly on the side of the Moon that faces Earth. The maria are pictured in Figure 6.7.
Lunar Maria
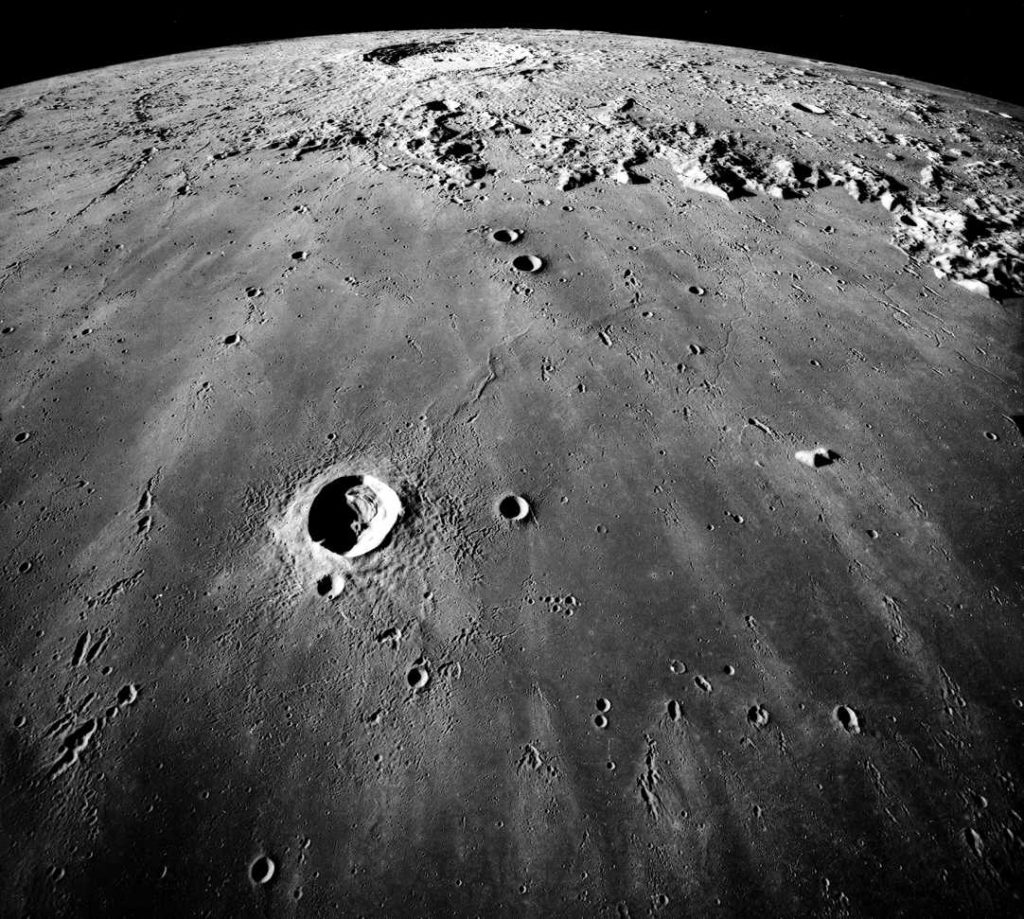
Mare Imbrium of Copernicus crater on the Moon by NASA/Apollo 17, NASA Media Licence.
Today, we know that the maria consist mostly of dark-coloured basalt (volcanic lava) laid down in volcanic eruptions billions of years ago. Eventually, these lava flows partly filled the huge depressions called impact basins, which had been produced by collisions of large chunks of material with the Moon relatively early in its history. The basalt on the Moon, as shown in Figure 6.8, is very similar in composition to the crust under the oceans of Earth or to the lavas erupted by many terrestrial volcanoes. The youngest of the lunar impact basins is Mare Orientale, shown in Figure 6.9.
Rock from a Lunar Mare
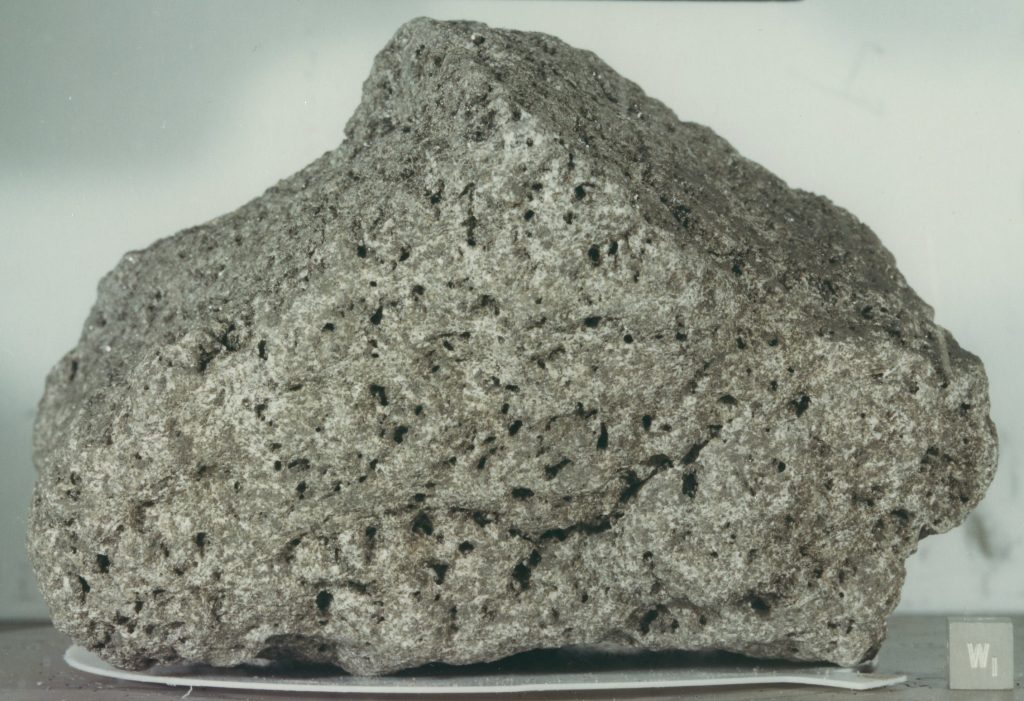
Lunar basalt 70017 by NASA/Apollo 17, NASA Media Licence.
Mare Orientale
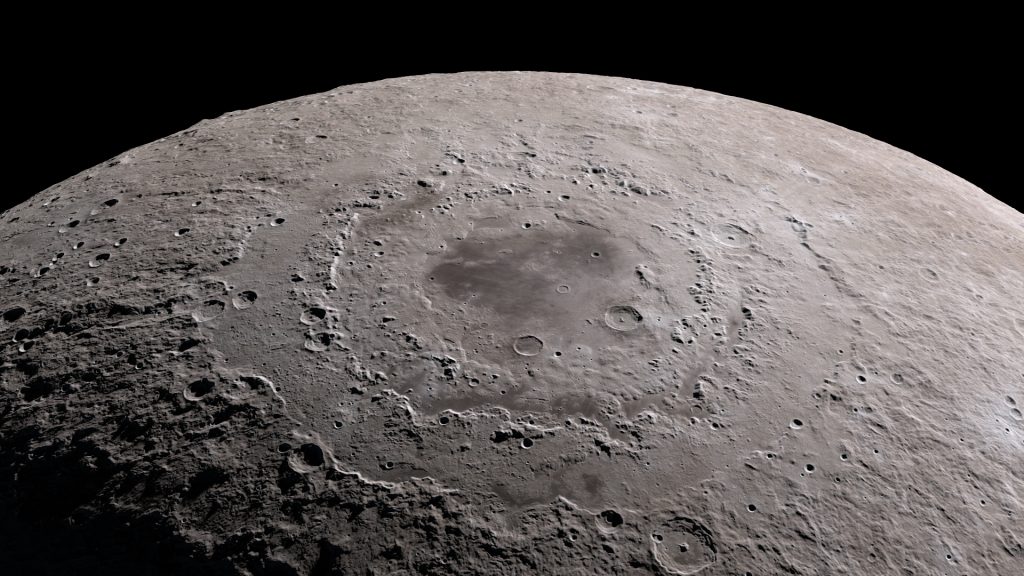
Orientale Basin by NASA Goddard Space Flight Center, CC BY 2.0.
Volcanic activity may have begun very early in the Moon’s history, although most evidence of the first half billion years is lost. What we do know is that the major mare volcanism, which involved the release of lava from hundreds of kilometres below the surface, ended about 3.3 billion years ago. After that, the Moon’s interior cooled, and volcanic activity was limited to a very few small areas. The primary forces altering the surface come from the outside, not the interior.
Composition and Structure of the Moon
The composition of the Moon is not the same as that of Earth. With an average density of only 3.3 g/cm3, the Moon must be made almost entirely of silicate rock. Compared to Earth, it is depleted in iron and other metals. It is as if the Moon were composed of the same silicates as Earth’s mantle and crust, with the metals and the volatiles selectively removed. These differences in composition between Earth and Moon provide important clues about the origin of the Moon, a topic we will cover in detail later in this chapter.
Studies of the Moon’s interior carried out with seismometres taken to the Moon as part of the Apollo program confirm the absence of a large metal core. The twin GRAIL spacecraft launched into lunar orbit in 2011 provided even more precise tracking of the interior structure. We also know from the study of lunar samples that water and other volatiles have been depleted from the lunar crust. The tiny amounts of water detected in these samples were originally attributed to small leaks in the container seal that admitted water vapor from Earth’s atmosphere. However, scientists have now concluded that some chemically bound water is present in the lunar rocks.
Most dramatically, water ice has been detected in permanently shadowed craters near the lunar poles. In 2009, NASA crashed a small spacecraft called the Lunar Crater Observation and Sensing Satellite (LCROSS) into the crater Cabeus near the Moon’s south pole. The impact at 9,000 kilometres per hour released energy equivalent to 2 tons of dynamite, blasting a plume of water vapor and other chemicals high above the surface. This plume was visible to telescopes in orbit around the Moon, and the LCROSS spacecraft itself made measurements as it flew through the plume. A NASA spacecraft called the Lunar Reconnaissance Orbiter (LRO) also measured the very low temperatures inside several lunar craters, and its sensitive cameras were even able to image crater interiors by starlight.
The total quantity of water ice in the Moon’s polar craters is estimated to be hundreds of billions of tons. As liquid, this would only be enough water to fill a lake 100 miles across, but compared with the rest of the dry lunar crust, so much water is remarkable. Presumably, this polar water was carried to the Moon by comets and asteroids that hit its surface. Some small fraction of the water froze in a few extremely cold regions (cold traps) where the Sun never shines, such as the bottom of deep craters at the Moon’s poles. One reason this discovery could be important is that it raises the possibility of future human habitation near the lunar poles, or even of a lunar base as a way-station on routes to Mars and the rest of the solar system. If the ice could be mined, it would yield both water and oxygen for human support, and it could be broken down into hydrogen and oxygen, a potent rocket fuel.
It is characteristic of modern science to ask how things originated. Understanding the origin of the Moon has proven to be challenging for planetary scientists, however. Part of the difficulty is simply that we know so much about the Moon (quite the opposite of our usual problem in astronomy). As we will see, one key problem is that the Moon is both tantalizingly similar to Earth and frustratingly different.
Early Ideas for the Origin of the Moon
Most of the earlier hypotheses for the Moon’s origin followed one of three general ideas:
- The fission hypothesis—the Moon was once part of Earth, but somehow separated from it early in their history.
- The sister hypothesis—the Moon formed together with (but independent of) Earth, as we believe many moons of the outer planets formed.
- The capture hypothesis—the Moon formed elsewhere in the solar system and was captured by Earth.
Unfortunately, there seem to be fundamental problems with each of these ideas. Perhaps the easiest hypothesis to reject is the capture hypothesis. Its primary drawback is that no one knows of any way that early Earth could have captured such a large moon from elsewhere. One body approaching another cannot go into orbit around it without a substantial loss of energy; this is the reason that spacecraft destined to orbit other planets are equipped with retro-rockets. Furthermore, if such a capture did take place, the captured object would go into a very eccentric orbit rather than the nearly circular orbit our Moon occupies today. Finally, there are too many compositional similarities between Earth and the Moon, particularly an identical fraction of the major isotopes1 of oxygen, to justify seeking a completely independent origin.
The fission hypothesis, which states that the Moon separated from Earth, was suggested in the late nineteenth century. Modern calculations have shown that this sort of spontaneous fission or splitting is impossible. Furthermore, it is difficult to understand how a Moon made out of terrestrial material in this way could have developed the many distinctive chemical differences now known to characterize our neighbor.
Scientists were therefore left with the sister hypothesis—that the Moon formed alongside Earth—or with some modification of the fission hypothesis that can find a more acceptable way for the lunar material to have separated from Earth. But the more we learned about our Moon, the less these old ideas seem to fit the bill.
The Giant Impact Hypothesis – Currently accepted
In an effort to resolve these apparent contradictions, scientists developed a fourth hypothesis for the origin of the Moon, one that involves a giant impact early in Earth’s history. There is increasing evidence that large chunks of material—objects of essentially planetary mass—were orbiting in the inner solar system at the time that the terrestrial planets formed. The giant impact hypothesis envisions Earth being struck obliquely by an object approximately one-tenth Earth’s mass—a “bullet” about the size of Mars. This is very nearly the largest impact Earth could experience without being shattered.
Such an impact would disrupt much of Earth and eject a vast amount of material into space, releasing almost enough energy to break the planet apart. Computer simulations indicate that material totaling several percent of Earth’s mass could be ejected in such an impact. Most of this material would be from the stony mantles of Earth and the impacting body, not from their metal cores. This ejected rock vapor then cooled and formed a ring of material orbiting Earth. It was this ring that ultimately condensed into the Moon.
While we do not have any current way of showing that the giant impact hypothesis is the correct model of the Moon’s origin, it does offer potential solutions to most of the major problems raised by the chemistry of the Moon. First, since the Moon’s raw material is derived from the mantles of Earth and the projectile, the absence of metals is easily understood. Second, most of the volatile elements would have been lost during the high-temperature phase following the impact, explaining the lack of these materials on the Moon. Yet, by making the Moon primarily of terrestrial mantle material, it is also possible to understand similarities such as identical abundances of various oxygen isotopes.
This is a link to the current research on this topic. https://www.psi.edu/epo/moon/moon.html.
Attribution
“9.1 General Properties of the Moon”, “9.2 The Lunar Surface”, and “9.4 The Origin of the Moon” are from Astronomy by Department of Physics and Astronomy at Douglas College is licensed under a Creative Commons Attribution 4.0 International License, except where otherwise noted.