4.8 Optical Detectors and Adaptive Optics
Throughout most of the twentieth century, photographic film or glass plates served as the prime astronomical detectors, whether for photographing spectra or direct images of celestial objects. In a photographic plate, a light-sensitive chemical coating is applied to a piece of glass that, when developed, provides a lasting record of the image. At observatories around the world, vast collections of photographs preserve what the sky has looked like during the past 100 years. Photography represents a huge improvement over the human eye, but it still has limitations. Photographic films are inefficient: only about 1% of the light that actually falls on the film contributes to the chemical change that makes the image; the rest is wasted.
Astronomers today have much more efficient electronic detectors to record astronomical images. Most often, these are charge-coupled devices (CCDs), which are similar to the detectors used in video camcorders or in digital cameras (like the one more and more students have on their cell phones). In a CCD, photons of radiation hitting any part of the detector generate a stream of charged particles (electrons) that are stored and counted at the end of the exposure. Each place where the radiation is counted is called a pixel (picture element), and modern detectors can count the photons in millions of pixels (megapixels, or MPs).
Charge-Coupled Devices (CCDs)
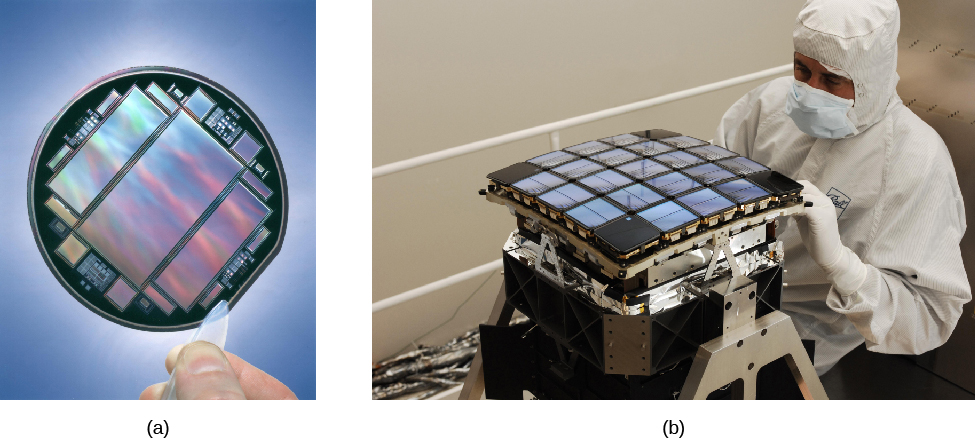
(a): Fabrication run 3 (100 mm wafers) by Berkeley Lab/US Department of Energy, Berkeley Lab Media Licence.
(b): Kepler Focal Plane Array by NASA/Ball Aerospace, NASA Media Licence.
Because CCDs typically record as much as 60–70% of all the photons that strike them, and the best silicon and infrared CCDs exceed 90% sensitivity, we can detect much fainter objects. Among these are many small moons around the outer planets, icy dwarf planets beyond Pluto, and dwarf galaxies of stars. CCDs also provide more accurate measurements of the brightness of astronomical objects than photography, and their output is digital—in the form of numbers that can go directly into a computer for analysis.
Charge-coupled devices are not only used for detailed astronomical observations but are also the basis for most digital photography. Canadian physicist Willard S. Boyle (and American George E. Smith) created the first CCD in 1969 at Bell Labs. They shared the 2009 Nobel Prize in Physics for this new “electronic eye” along with Charles K. Kao for his work on fibre optic technology.
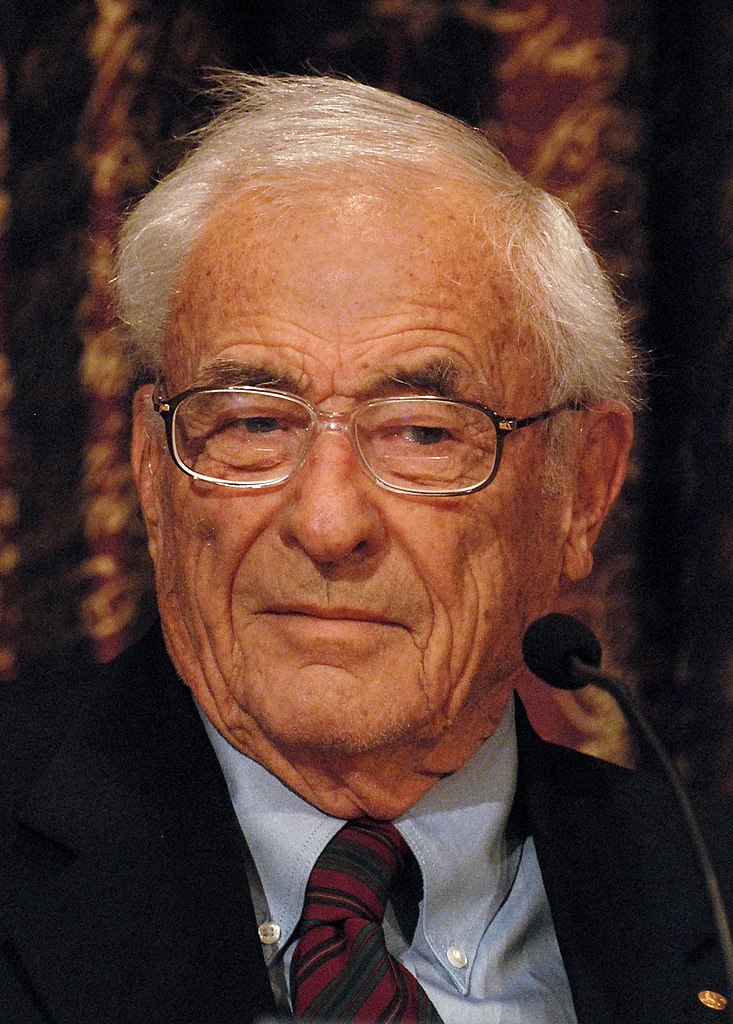
Willard Boyle by Prolineserver, CC BY-SA 3.0.
Willard Boyle was born in 1924 in Amherst, Nova Scotia. He completed his education at McGill University with a Doctorate in Physics in 1950 after flying Spitfire fighter planes for the Royal Canadian Navy. Besides inventing the CCD, Willard was a co-creator of the first continuously operating ruby laser. He also worked as the Director of Space Science and Exploratory Studies at Bellcom (a division of Bell Labs) and supported the Apollo space program. Specifically, Willard aided in the selection of certain lunar landing sites during the 1960s. Willard worked on 18 patents during his career at Bell Labs and won several other awards for his work. In 2010, he was appointed a Companion to the Order of Canada before passing in 2011.
When fast-acting cameras, and faster-acting computers, were developed in the 1970s and 1980s, some astronomers began to think about ways to get around the blurring effects of the atmosphere. They have developed several approaches to the problem, but all are given the name adaptive optics.
The basic idea sounds pretty simple:
- take a quick picture of a bright object with a “fast camera”
- measure quantitatively how imperfect the image is
- figure out how the atmosphere must be distorting the light rays right now
- using an active optical element, distort the light rays in exactly the OPPOSITE manner
- focus the un-distorted light rays on a “slow camera”
The first thing one needs is a “fast camera”, because the motion of the air changes the distortion of light rays many times each second. Typical “fast cameras” run at video rate—30 times per second—or even faster; some take over 1000 frames per second!
What sort of thing is an active optical element? There are several different types, but most are basically flexible mirrors with lots of little actuators to bend them. Some are small:
and others are large:
Mirror on the MMT
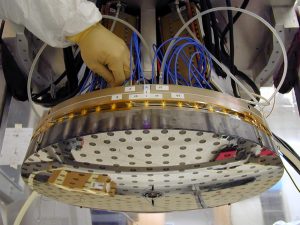
How well can these systems correct the air’s distortions? Pretty darn well! It turns out to be easier for light of long wavelengths, in the near-infrared portion of the spectrum. Let’s look at one example, taken with the MMT, a telescope of diameter roughly [latex]\text{D}=6.5[/latex] m, at a wavelength of [latex]\lambda=1650[/latex] nm.
Movie of Theta Ori 1
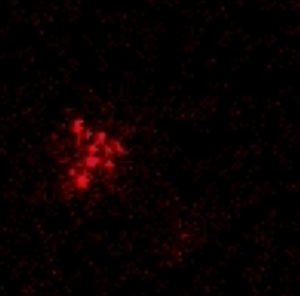
Okay, let’s compare that to the actual performance:
Recently, astronomers have managed to use adaptive optics to improve images in the optical regime, at wavelengths we can see with our own eyes.
Attribution
“6.3 Visible-Light Detectors and Instruments” from Douglas College Astronomy 1105 by Douglas College Department of Physics and Astronomy, is licensed under a Creative Commons Attribution 4.0 International License, except where otherwise noted. Adapted from Astronomy 2e.
“Removing the effect of the atmosphere” from Adpative Optics by Michael Richmond is licensed under a Creative Commons Attribution Non-Commercial ShareAlike 4.0 International License, except where otherwise noted.