13.2 The Cosmic Time Machine
Let’s begin by exploring some techniques astronomers use to study how galaxies are born and change over cosmic time. Suppose you wanted to understand how adult humans got to be the way they are. If you were very dedicated and patient, you could actually observe a sample of babies from birth, following them through childhood, adolescence, and into adulthood, and making basic measurements such as their heights, weights, and the proportional sizes of different parts of their bodies to understand how they change over time.
Unfortunately, we have no such possibility for understanding how galaxies grow and change over time: in a human lifetime—or even over the entire history of human civilization—individual galaxies change hardly at all. We need other tools than just patiently observing single galaxies in order to study and understand those long, slow changes.
We do, however, have one remarkable asset in studying galactic evolution. As we have seen, the universe itself is a kind of time machine that permits us to observe remote galaxies as they were long ago. For the closest galaxies, like the Andromeda galaxy, the time the light takes to reach us is on the order of a few hundred thousand to a few million years. Typically not much changes over times that short—individual stars in the galaxy may be born or die, but the overall structure and appearance of the galaxy will remain the same. But we have observed galaxies so far away that we are seeing them as they were when the light left them more than 10 billion years ago.
By observing more distant objects, we look further back toward a time when both galaxies and the universe were young, shown in Figure 13.2. This is a bit like getting letters in the mail from several distant friends: the farther the friend was when she mailed the letter to you, the longer the letter must have been in transit, and so the older the news is when it arrives in your mailbox; you are learning something about her life at an earlier time than when you read the letter.
Astronomical Time Travel
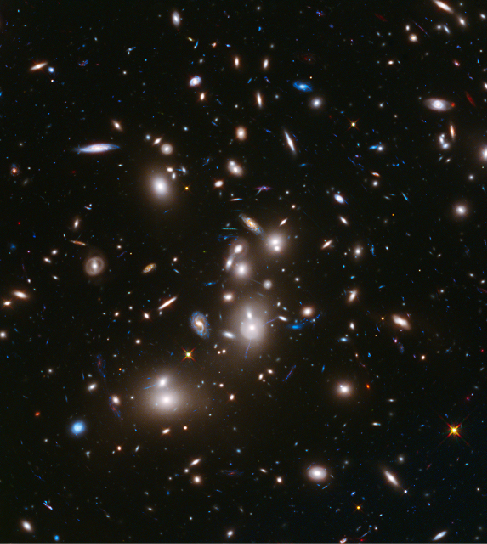
Abell 2744 by NASA, ESA, STScI, NASA Media License.
If we can’t directly detect the changes over time in individual galaxies because they happen too slowly, how then can we ever understand those changes and the origins of galaxies? The solution is to observe many galaxies at many different cosmic distances and, therefore, look-back times (how far back in time we are seeing the galaxy). If we can study a thousand very distant “baby” galaxies when the universe was 1 billion years old, and another thousand slightly closer “toddler” galaxies when it was 2 billion years old, and so on until the present 13.8-billion-year-old universe of mature “adult” galaxies near us today, then maybe we can piece together a coherent picture of how the whole ensemble of galaxies evolves over time. This allows us to reconstruct the “life story” of galaxies since the universe began, even though we can’t follow a single galaxy from infancy to old age.
Fortunately, there is no shortage of galaxies to study. Hold up your pinky at arm’s length: the part of the sky blocked by your fingernail contains about one million galaxies, layered farther and farther back in space and time. In fact, the sky is filled with galaxies, all of them, except for Andromeda and the Magellanic Clouds, too faint to see with the naked eye—more than 100 billion galaxies in the observable universe, each one with about 100 billion stars.
This cosmic time machine, then, lets us peer into the past to answer fundamental questions about where galaxies come from and how they got to be the way they are today. Astronomers call those galactic changes over cosmic time evolution, a word that recalls the work of Darwin and others on the development of life on Earth. But note that galaxy evolution refers to the changes in individual galaxies over time, while the kind of evolution biologists study is changes in successive generations of living organisms over time.
Astronomy is one of the few sciences in which all measurements must be made at a distance. Geologists can take samples of the objects they are studying; chemists can conduct experiments in their laboratories to determine what a substance is made of; archeologists can use carbon dating to determine how old something is. But astronomers can’t pick up and play with a star or galaxy. As we have seen throughout this book, if they want to know what galaxies are made of and how they have changed over the lifetime of the universe, they must decode the messages carried by the small number of photons that reach Earth.
Fortunately (as you have learned) electromagnetic radiation is a rich source of information. The distance to a galaxy is derived from its redshift (how much the lines in its spectrum are shifted to the red because of the expansion of the universe). The conversion of redshift to a distance depends on certain properties of the universe, including the value of the Hubble constant and how much mass it contains. We will describe the currently accepted model of the universe in a later chapter. For the purposes of this chapter, it is enough to know that the current best estimate for the age of the universe is 13.8 billion years. In that case, if we see an object that emitted its light 6 billion light-years ago, we are seeing it as it was when the universe was almost 8 billion years old. If we see something that emitted its light 13 billion years ago, we are seeing it as it was when the universe was less than a billion years old. So astronomers measure a galaxy’s redshift from its spectrum, use the Hubble constant plus a model of the universe to turn the redshift into a distance, and use the distance and the constant speed of light to infer how far back in time they are seeing the galaxy—the look-back time.
Another important clue to the nature of a galaxy is its shape. Spiral galaxies can be distinguished from elliptical galaxies by shape. Observations show that spiral galaxies contain young stars and large amounts of interstellar matter, while elliptical galaxies have mostly old stars and very little or no star formation. Elliptical galaxies turned most of their interstellar matter into stars many billions of years ago, while star formation has continued until the present day in spiral galaxies.
If we can count the number of galaxies of each type during each epoch of the universe, it will help us understand how the pace of star formation changes with time. As we will see later in this chapter, galaxies in the distant universe—that is, young galaxies—look very different from the older galaxies that we see nearby in the present-day universe.
In addition to looking at the most distant galaxies we can find, astronomers look at the oldest stars (what we might call the fossil record) of our own Galaxy to probe what happened in the early universe. Since stars are the source of nearly all the light emitted by galaxies, we can learn a lot about the evolution of galaxies by studying the stars within them. What we find is that nearly all galaxies contain at least some very old stars. For example, our own Galaxy contains globular clusters with stars that are at least 13 billion years old, and some may be even older than that. Therefore, if we count the age of the Milky Way as the age of its oldest constituents, the Milky Way must have been born at least 13 billion years ago.
When we make computer models of how such galaxies evolve with time, they tell us that star formation in elliptical galaxies began less than a billion years or so after the universe started its expansion, and new stars continued to form for a few billion years. But then star formation apparently stopped. When we compare distant elliptical galaxies with ones nearby, we find that ellipticals have not changed very much since the universe reached about half its current age. We’ll return to this idea later in the chapter.
Observations of the most luminous galaxies take us even further back in time. Recently, as we have already noted, astronomers have discovered a few galaxies that are so far away that the light we see now left them less than a billion years or so after the beginning as seen in Figure 13.3. Yet the spectra of some of these galaxies already contain lines of heavy elements, including carbon, silicon, aluminum, and sulphur. These elements were not present when the universe began but had to be manufactured in the interiors of stars. This means that when the light from these galaxies was emitted, an entire generation of stars had already been born, lived out their lives, and died—spewing out the new elements made in their interiors through supernova explosions—even before the universe was a billion years old. And it wasn’t just a few stars in each galaxy that got started this way. Enough had to live and die to affect the overall composition of the galaxy, in a way that we can still measure in the spectrum from far away.
In August of 2018, United Kingdon astronomers published results showing that there were galaxies that were formed only a few hundred million years after the Big Bang. https://www.bbc.com/news/science-environment-45198764
Very Distant Galaxy

EGSY8p7 by I. Labbé (Leiden University), NASA/ESA/JPL-Caltech, Public Domain
Observations of quasars (galaxies whose centres contain a supermassive black hole) support this conclusion. We can measure the abundances of heavy elements in the gas near quasar black holes. The composition of this gas in quasars that emitted their light 12.5 billion light-years ago is very similar to that of the Sun. This means that a large portion of the gas surrounding the black holes must have already been cycled through stars during the first 1.3 billion years after the expansion of the universe began. If we allow time for this cycling, then their first stars must have formed when the universe was only a few hundred million years old.
Attribution
“28.1 Observations of Distant Galaxies” from Douglas College Astronomy 1105 by Douglas College Department of Physics and Astronomy, is licensed under a Creative Commons Attribution 4.0 International License, except where otherwise noted. Adapted from Astronomy 2e.