12.4 The Formation of the Milky Way
Information about stellar populations holds vital clues to how our Galaxy was built up over time. The flattened disk shape of the Galaxy suggests that it formed through a process similar to the one that leads to the formation of a protostar. Building on this idea, astronomers first developed models that assumed the Galaxy formed from a single rotating cloud. But, as we shall see, this turns out to be only part of the story.
The Protogalactic Cloud and the Monolithic Collapse Model
Because the oldest stars—those in the halo and in globular clusters—are distributed in a sphere centered on the nucleus of the Galaxy, it makes sense to assume that the protogalactic cloud that gave birth to our Galaxy was roughly spherical. The oldest stars in the halo have ages of 12 to 13 billion years, so we estimate that the formation of the Galaxy began about that long ago. Then, just as in the case of star formation, the protogalactic cloud collapsed and formed a thin rotating disk. Stars born before the cloud collapsed did not participate in the collapse, but have continued to orbit in the halo to the present day as shown in Figure 12.16.
Monolithic Collapse Model for the Formation of the Galaxy

Gravitational forces caused the gas in the thin disk to fragment into clouds or clumps with masses like those of star clusters. These individual clouds then fragmented further to form stars. Since the oldest stars in the disk are nearly as old as the youngest stars in the halo, the collapse must have been rapid (astronomically speaking), requiring perhaps no more than a few hundred million years.
Collision Victims and the Multiple Merger Model
In past decades, astronomers have learned that the evolution of the Galaxy has not been quite as peaceful as this monolithic collapse model suggests. In 1994, astronomers discovered a small new galaxy in the direction of the constellation of Sagittarius. The Sagittarius dwarf galaxy is currently about 70,000 light-years away from Earth and 50,000 light-years from the centre of the Galaxy. It is the closest galaxy known. Pictured in Figure 12.17. It is very elongated, and its shape indicates that it is being torn apart by our Galaxy’s gravitational tides—just as Comet Shoemaker-Levy 9 was torn apart when it passed too close to Jupiter in 1992.
The Sagittarius galaxy is much smaller than the Milky Way, with only about 150,000 stars, all of which seem destined to end up in the bulge and halo of our own Galaxy. But don’t sound the funeral bells for the little galaxy quite yet; the ingestion of the Sagittarius dwarf will take another 100 million years or so, and the stars themselves will survive.
Sagittarius Dwarf
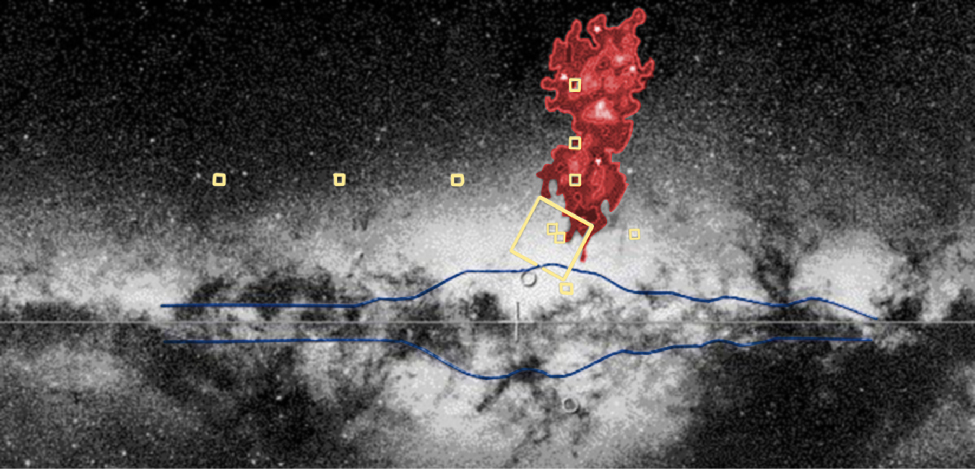
Modification of Sagittarius Dwarf to Collide with Milky Way by R. Ibata (UBC), R. Wyse (JHU), R. Sword (IoA), NASA Media License.
Since that discovery, evidence has been found for many more close encounters between our Galaxy and other neighbour galaxies. When a small galaxy ventures too close, the force of gravity exerted by our Galaxy tugs harder on the near side than on the far side. The net effect is that the stars that originally belonged to the small galaxy are spread out into a long stream that orbits through the halo of the Milky Way as seen in Figure 12.18.
Streams in the Galactic Halo
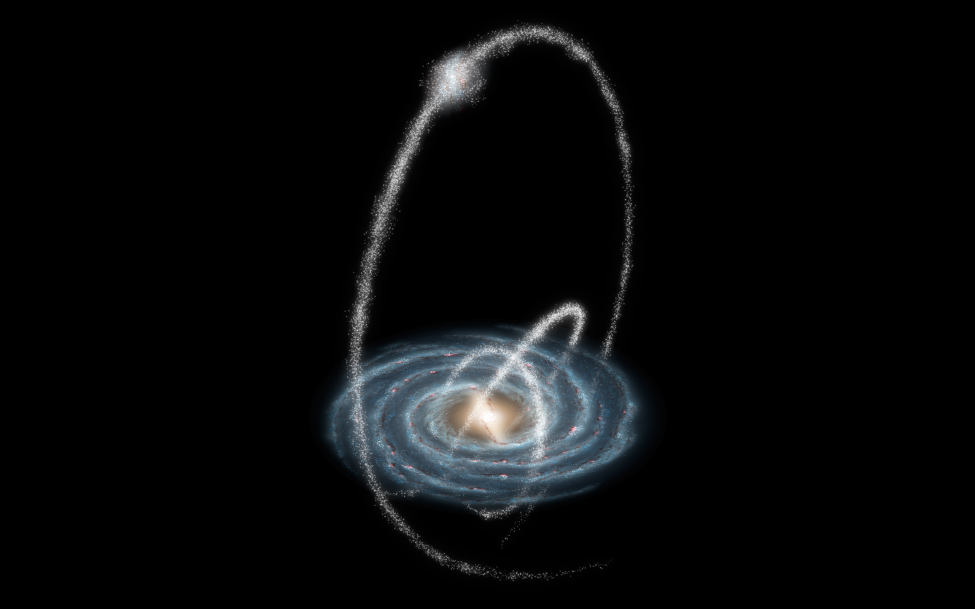
Artist´s impression of the star and dust tail from the torn-to-pieces Sagittarius dwarf galaxy, currently being engulfed by the Milky Way by NASA/JPL-Caltech/R. Hurt (SSC/Caltech), Public Domain
Such a tidal stream can maintain its identity for billions of years. To date, astronomers have now identified streams originating from 12 small galaxies that ventured too close to the much larger Milky Way. Six more streams are associated with globular clusters. It has been suggested that large globular clusters, like Omega Centauri, are actually dense nuclei of cannibalized dwarf galaxies. The globular cluster M54 is now thought to be the nucleus of the Sagittarius dwarf we discussed earlier, which is currently merging with the Milky Way as shown in Figure 12.19. The stars in the outer regions of such galaxies are stripped off by the gravitational pull of the Milky Way, but the central dense regions may survive.
Globular Cluster M54

Messier 54 by ESA/Hubble & NASA, NASA Media License.
Calculations indicate that the Galaxy’s thick disk may be a product of one or more such collisions with other galaxies. Accretion of a satellite galaxy would stir up the orbits of the stars and gas clouds originally in the thin disk and cause them to move higher above and below the mid-plane of the Galaxy. Meanwhile, the Galaxy’s stars would add to the fluffed-up mix. If such a collision happened about 10 billion years ago, then any gas in the two galaxies that had not yet formed into stars would have had plenty of time to settle back down into the thin disk. The gas could then have begun forming subsequent generations of population I stars. This timing is also consistent with the typical ages of stars in the thick disk.
The Milky Way has more collisions in store. An example is the Canis Major dwarf galaxy, which has a mass of about 1% of the mass of the Milky Way. Already long tidal tails have been stripped from this galaxy, which have wrapped themselves around the Milky Way three times. Several of the globular clusters found in the Milky Way may also have come from the Canis Major dwarf, which is expected to merge gradually with the Milky Way over about the next billion years.
In about 3 billion years, the Milky Way itself will be swallowed up, since it and the Andromeda galaxy are on a collision course. Our computer models show that after a complex interaction, the two will merge to form a larger, more rounded galaxy, shown in Figure 12.20.
Collision of the Milky Way with Andromeda
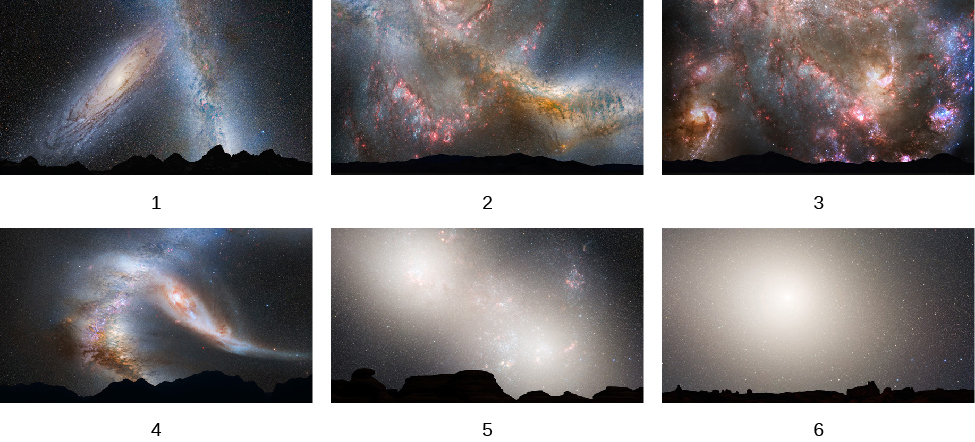
Illustration Sequence of the Milky Way and Andromeda Galaxy Colliding by NASA,ESA, Z. Levay, R. van der Marel, STScl, T. Hallas, and A. Mellinger, NASA Media License.
We are thus coming to realize that “environmental influences” (and not just a galaxy’s original characteristics) play an important role in determining the properties and development of our Galaxy. In future chapters we will see that collisions and mergers are a major factor in the evolution of many other galaxies as well.
At the Sun’s distance from its centre, the Galaxy does not rotate like a solid wheel or a CD inside your player. Instead, the way individual objects turn around the centre of the Galaxy is more like the solar system. Stars, as well as the clouds of gas and dust, obey Kepler’s third law. Objects farther from the centre take longer to complete an orbit around the Galaxy than do those closer to the centre. In other words, stars (and interstellar matter) in larger orbits in the Galaxy trail behind those in smaller ones. This effect is called differential galactic rotation.
Differential rotation would appear to explain why so much of the material in the disk of the Milky Way is concentrated into elongated features that resemble spiral arms. No matter what the original distribution of the material might be, the differential rotation of the Galaxy can stretch it out into spiral features. Figure 12.21 shows the development of spiral arms from two irregular blobs of interstellar matter. Notice that as the portions of the blobs closest to the galactic centre move faster, those farther out trail behind.
Simplified Model for the Formation of Spiral Arms
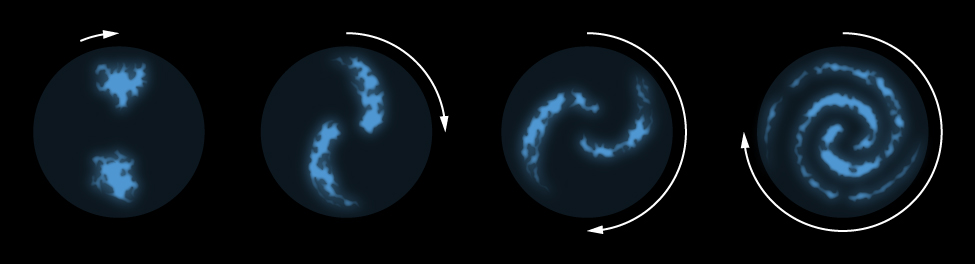
But this picture of spiral arms presents astronomers with an immediate problem. If that’s all there were to the story, differential rotation—over the roughly 13-billion-year history of the Galaxy—would have wound the Galaxy’s arms tighter and tighter until all semblance of spiral structure had disappeared. But did the Milky Way actually have spiral arms when it formed 13 billion years ago? And do spiral arms, once formed, last for that long a time?
With the advent of the Hubble Space Telescope, it has become possible to observe the structure of very distant galaxies and to see what they were like shortly after they began to form more than 13 billion years ago. What the observations show is that galaxies in their infancy had bright, clumpy star-forming regions, but no regular spiral structure.
Over the next few billion years, the galaxies began to “settle down.” The galaxies that were to become spirals lost their massive clumps and developed a central bulge. The turbulence in these galaxies decreased, rotation began to dominate the motions of the stars and gas, and stars began to form in a much quieter disk. Smaller star-forming clumps began to form fuzzy, not-very-distinct spiral arms. Bright, well-defined spiral arms began to appear only when the galaxies were about 3.6 billion years old. Initially, there were two well-defined arms. Multi-armed structures in galaxies like we see in the Milky Way appeared only when the universe was about 8 billion years old.
Scientists have used supercomputer calculations to model the formation and evolution of the arms. These calculations follow the motions of up to 100 million “star particles” to see whether gravitational forces can cause them to form spiral structure. What these calculations show is that giant molecular clouds have enough gravitational influence over their surroundings to initiate the formation of structures that look like spiral arms. These arms then become self-perpetuating and can survive for at least several billion years. The arms may change their brightness over time as star formation comes and goes, but they are not temporary features. The concentration of matter in the arms exerts sufficient gravitational force to keep the arms together over long periods of time.
Attribution
“25.6 The Formation of the Galaxy” and “25.2 Spiral Structure” from Douglas College Astronomy 1105 by Douglas College Department of Physics and Astronomy, are licensed under a Creative Commons Attribution 4.0 International License, except where otherwise noted. Adapted from Astronomy 2e.