10.2 What Powers the Sun
The Sun
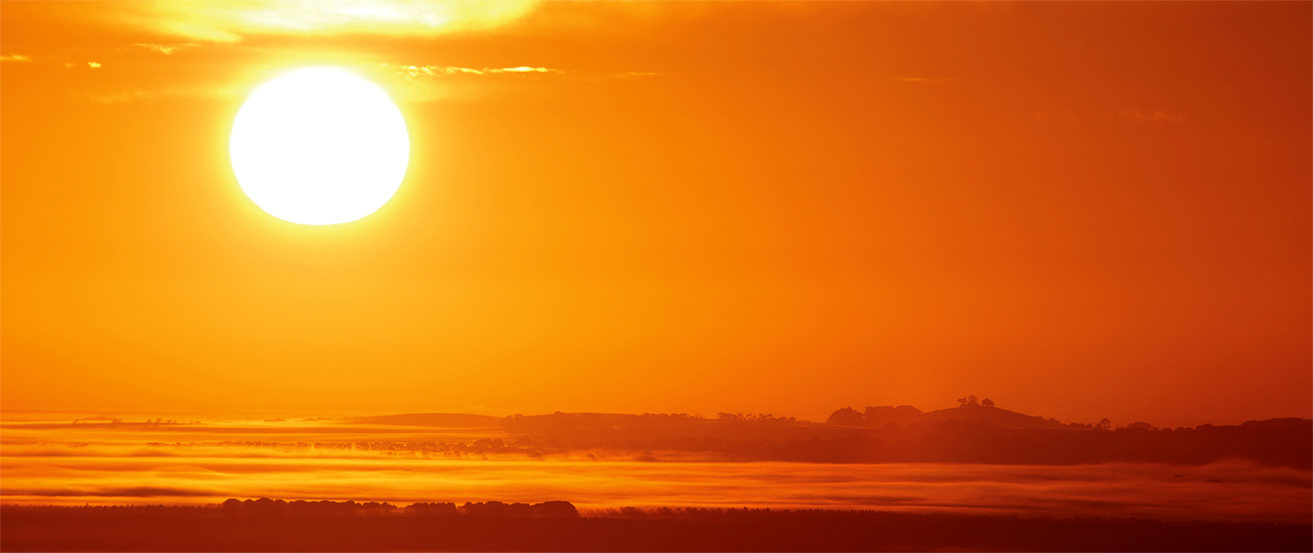
Sun and Fog by Ed Dunens, CC-BY-4.0
The Sun puts out an incomprehensible amount of energy—so much that its ultraviolet radiation can cause sunburns from 93 million miles away. It is also very old. As you learned earlier, evidence shows that the Sun formed about 4.5 billion years ago and has been shining ever since. How can the Sun produce so much energy for so long?
The Sun’s energy output is about 4 × 1026 watts. This is unimaginably bright: brighter than a trillion cities together each with a trillion 100-watt light bulbs. Most known methods of generating energy fall far short of the capacity of the Sun. The total amount of energy produced over the entire life of the Sun is staggering, since the Sun has been shining for billions of years. Scientists were unable to explain the seemingly unlimited energy of stars like the Sun prior to the twentieth century.
When striving to understand how the Sun can put out so much energy for so long, scientists considered many different types of energy. Nineteenth-century scientists knew of two possible sources for the Sun’s energy: chemical and gravitational energy. The source of chemical energy most familiar to them was the burning (the chemical term is oxidation) of wood, coal, gasoline, or other fuel. We know exactly how much energy the burning of these materials can produce. We can thus calculate that even if the immense mass of the Sun consisted of a burnable material like coal or wood, our star could not produce energy at its present rate for more than few thousand years. However, we know from geologic evidence that water was present on Earth’s surface nearly 4 billion years ago, so the Sun must have been shining brightly (and making Earth warm) at least as long as that. Today, we also know that at the temperatures found in the Sun, nothing like solid wood or coal could survive.
It was only in the twentieth century that the true source of the Sun’s energy was identified. The two key pieces of information required to solve the puzzle were the structure of the nucleus of the atom and the fact that mass can be converted into energy.
The Sun, then, taps the energy contained in the nuclei of atoms through nuclear fusion. Let’s look at what happens in more detail. Deep inside the Sun, a three-step process takes four hydrogen nuclei and fuses them together to form a single helium nucleus. The helium nucleus is slightly less massive than the four hydrogen nuclei that combine to form it, and that mass is converted into energy.
The initial step required to form one helium nucleus from four hydrogen nuclei is shown in Figure 10.3. At the high temperatures inside the Sun’s core, two protons combine to make a deuterium nucleus, which is an isotope (or version) of hydrogen that contains one proton and one neutron. In effect, one of the original protons has been converted into a neutron in the fusion reaction. Electric charge has to be conserved in nuclear reactions, and it is conserved in this one. A positron (antimatter electron) emerges from the reaction and carries away the positive charge originally associated with one of the protons.
Proton-Proton Chain, Step 1
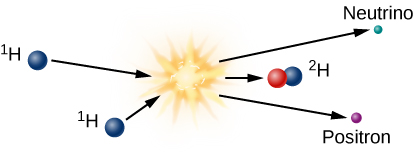
Since it is antimatter, this positron will instantly collide with a nearby electron, and both will be annihilated, producing electromagnetic energy in the form of gamma-ray photons. This gamma ray, which has been created in the centre of the Sun, finds itself in a world crammed full of fast-moving nuclei and electrons. The gamma ray collides with particles of matter and transfers its energy to one of them. The particle later emits another gamma-ray photon, but often the emitted photon has a bit less energy than the one that was absorbed.
Such interactions happen to gamma rays again and again and again as they make their way slowly toward the outer layers of the Sun, until their energy becomes so reduced that they are no longer gamma rays but X-rays. Later, as the photons lose still more energy through collisions in the crowded centre of the Sun, they become ultraviolet photons.
By the time they reach the Sun’s surface, most of the photons have given up enough energy to be ordinary light—and they are the sunlight we see coming from our star. (To be precise, each gamma-ray photon is ultimately converted into many separate lower-energy photons of sunlight.) So, the sunlight given off by the Sun today had its origin as a gamma ray produced by nuclear reactions deep in the Sun’s core. The length of time that photons require to reach the surface depends on how far a photon on average travels between collisions, and the travel time depends on what model of the complicated solar interior we accept. Estimates are somewhat uncertain but indicate that the emission of energy from the surface of the Sun can lag its production in the interior by 100,000 years to as much as 1,000,000 years.
In addition to the positron, the fusion of two hydrogen atoms to form deuterium results in the emission of a neutrino. Because neutrinos interact so little with ordinary matter, those produced by fusion reactions near the centre of the Sun travel directly to the Sun’s surface and then out into space, in all directions. Neutrinos move at nearly the speed of light, and they escape the Sun about two seconds after they are created.
Proton-Proton Chain, Step 2

The second step in forming helium from hydrogen is to add another proton to the deuterium nucleus to create a helium nucleus that contains two protons and one neutron as illustrated in Figure 10.4. In the process, some mass is again lost and more gamma radiation is emitted. Such a nucleus is helium because an element is defined by its number of protons; any nucleus with two protons is called helium. But this form of helium, which we call helium-3 (and write in shorthand as 3He) is not the isotope we see in the Sun’s atmosphere or on Earth. That helium has two neutrons and two protons and hence is called helium-4 (4He).
To get to helium-4 in the Sun, helium-3 must combine with another helium-3 in the third step of fusion as illustrated in Figure 10.5. Note that two energetic protons are left over from this step; each of them comes out of the reaction ready to collide with other protons and to start step 1 in the chain of reactions all over again.
Proton-Proton Chain, Step 3
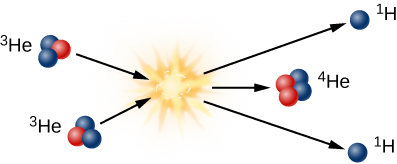
Attribution
“16.0 Thinking Ahead“, “16.1 Sources of Sunshine: Thermal and Gravitational Energy“, and “16.2 Mass, Energy, and the Theory of Relativity” from Douglas College Astronomy 1105 by Douglas College Department of Physics and Astronomy, is licensed under a Creative Commons Attribution 4.0 International License, except where otherwise noted. Adapted from Astronomy 2e.