23 6.6 – The Future of FMD
Learning Objectives
By the end of this section, you will be able to:
- Identify the major limitations of the fasting mimicking diet
- Think critically about factors that need to be considered for future research
- Identify plausible mechanisms by which FMD acts to improve human health
6.6.1. Limitations – Can FMD become a universal treatment?
There are some issues when it comes to implementing FMD in the real world and achieving similar benefits seen in this article. The fasting mimicking diet tested in this article was a potent and broad-spectrum diet hence this it should only be attempted with medical supervision (Brandhorst et al. 95). This brings into question the feasibility of FMD and how easy it is to adhere to for individuals who want to achieve the benefits of FMD at home. This factor combined with the other limitations of FMD (mentioned below) leave a lot of room for improvement. Furthermore, not many researchers have yet looked at the feasibility of FMD as large randomized control trials are needed to properly examine this (Cheng et al. 786).
Maintaining the benefits of FMD
The FMD diet was designed to maintain low circulating concentrations of insulin-like growth factor 1 (IGF-1), insulin, and glucose and at the same time increase plasma concentrations of IGF-binding protein 1 and ketone bodies (Francesco et al. 5) (figure 6.6.1). During the pilot study, these effects of FMD were observed in humans and low IGF-1, insulin and glucose were maintained after returning to a normal diet (Brandhorst et al. 94). However, this conflicts with the mouse trials during which maintaining the benefits after returning back to a “normal” diet proved difficult. It was observed that the effects of FMD on visceral fat, glucose and IFG-1, which were all reduced during or shortly after the FMD cycle, changed back to pre-FMD levels once the mice were allowed to feed freely (Brandhorst et al. 87). A better understanding of the mechanism by which FMD reduces risk factors may help alleviate this discrepancy in the data. Also, future studies need to design and implement randomized control trials that are well-controlled and yield significant results that can be translated to humans.
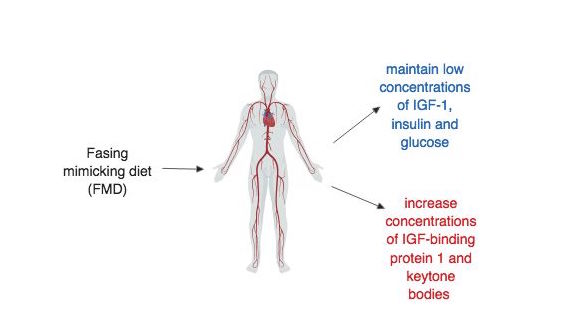
FMD and Age
FMD has been observed to increase the lifespan of mice but this effect was not consistent across all age groups. In very old mice death occurred during or shortly after completing the cycle of FMD (Brandhorst et al. 93). Thus, in older mice, FMD may be detrimental. Furthermore, another group of researchers cited that FMD did not have effects on maximum longevity in mice and similarly mentioned the detrimental effects of FMD in older mice (Francesco et al. 5). One way to move past this issue could include shortened cycles of FMD that may last 3 days instead of 4 which may reduce malnourishment and still provide benefits (Brandhorst et al. 93).
The female participants in the FMD cohort of the human pilot study by Brandhorst et al. were an average age of 41.8 +/- 4.9 years and the male participants in the FMD cohort were an average age of 41.5 +/- 3.5 years (94). Therefore research suggests that this group of participants was too young to observe any age-related decline in longevity or healthspan due to FMD. Such age effects on humans need to be further investigated but in some literature, there have already been suggestions of similar age effects in humans.
Due to the fact that the human FMD is a plant-based diet, it does not incorporate some other key aspects of human diet such as proteins and carbohydrates. The omission of protein and carbohydrates may not be an issue for most people as FMD is done in intervals with a pre-FMD diet typically resuming after the 5-day FMD cycle (Francesco et al. 5). For the older population (aged 65 +) the lack of protein especially may negatively affect the older person’s lifespan rather than providing any benefits (Levine et al. 407). Similarly to an FMD diet, low-protein diets reduce IGF-1 which has been associated with great reductions age-related diseases (Levine et al. 407). When examining participants aged 50 – 65 it has been shown that high protein diets resulted in a 75% increase in overall mortality and a 4-fold increase in the risk of cancer death (Levine et al. 407). Low protein diets in participants in the age 50 – 65 population have been shown to improve disease outcomes, lower overall mortality and lower cancer mortality (Levine et al. 407). However, in older populations (aged 65 +), reduced intake of protein is associated with an increase in overall mortality and an increase in cancer mortality (Levine et al. 407) (figure 6.6.2). This may be due to the weight decline that occurs as humans age whereby older humans with lower body mass may be more susceptible to protein malnourishment (Levine et al. 414).
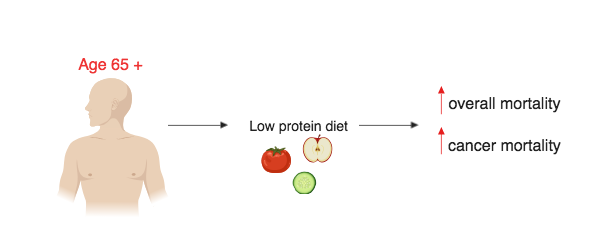
Aside: Are social factors worth considering?
Some determinants of health and longevity include socioeconomic status (SES), energy, quality of the environment, and genetics (Francesco et al. 1). The only factor a person has direct control over is energy intake, in other words, the sustenance a person receives in the form of food consumption (Francesco et al. 1). FMD relies on plant-based soups, herbal tea, energy bars, nut-based snacks that are all slowly implemented into the diet in 5-day cycles once a month for 3 months (Francesco et al. 5). The most recently published “Canada’s Price Report 2019” found that the price of plant-based products such as fruits and vegetables will significantly increase in the year 2019 (5). This increase in food prices may make pursuing FMD more difficult to adhere to for people who may have low socioeconomic status. FMD has a wide array of effects on cardiovascular disease, diabetes and cancer and it has been shown to be potentially beneficial in reducing the risk factors associated with these diseases (Brandhorst et al. 86). Thus, it is important to consider social factors when assessing the feasibility of a potential new treatment to make sure that if FMD is implemented as a treatment method in the future then barriers to access are eliminated.
Test your knowledge:
6.6.2. Need for Clinical Trials and Possible Mechanisms
Brandhorst et al. provide a good starting point which can be used to further investigate FMD so that it can be incorporated into treatment methods, but more work is needed to confirm these findings and solidify associations between FMD and disease risk factors. Larger randomized control trials will be needed to achieve this, and they will need to investigate the effects of FMD cycles on biomarkers and risk factors for ageing, diabetes, cancer and other common diseases (Brandhorst et al. 95). Future studies should also look at the feasibility of FMD, how accessible is to various populations and how likely it is that people will be able to adhere to and comply with the diet. FMD diets that do not need medical supervision and are easy to administer at home should also be considered for future studies.
Possible Mechanisms
Although there is still a long way to go before mechanisms can be fully understood there a number of studies that are examining the possible therapeutic roles of FMD and how FMD is reducing risk factors for certain diseases. Two examples will be further discussed in the sections to follow that demonstrate the potential for the therapeutic action FMD and possible mechanisms by which this is achieved. First, is the effect of FMD on diabetes whereby it had been proposed that FMD can induce antidiabetic effects by promoting the regeneration of pancreatic B cells, restoring insulin secretion and creating glucose homeostasis (Cheng et al. 775). It is thought that these antidiabetic effects occur through the regulation of the protein kinase A (PKA) and mTOR (mammalian target of rapamycin) pathways (Cheng et al. 775). Second, is the effect of FMD on autoimmune diseases such as multiple sclerosis where researchers have found that FMD can help control the disease and reduce disease severity through immune system regulation (Choi et al. 2136).
Example 1: FMD and Diabetes
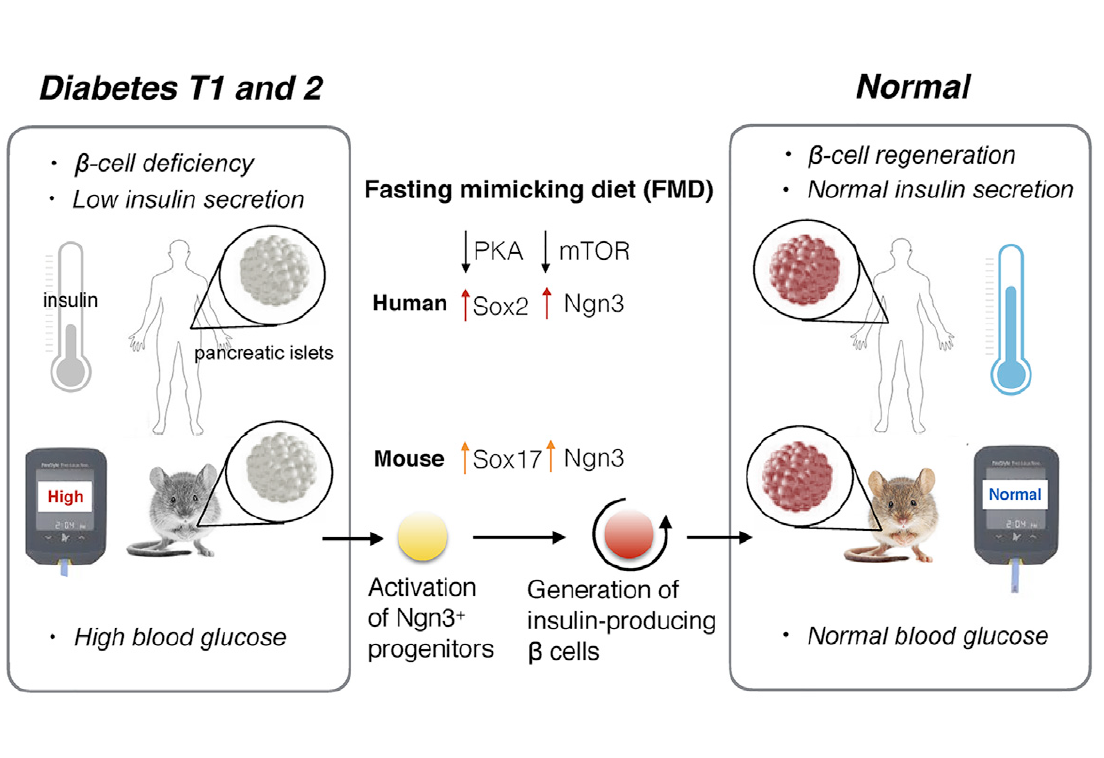
Brandhorst et al. observed a decrease in risk factors/biomarkers associated with diabetes during the randomized control pilot study (86). However, they did not propose a mechanism by which this reduction occurs. Cheng et al. have observed that FMD had therapeutic effects on diabetes and proposed a mechanism by which this may occur. In mice, a 4-day fasting mimicking diet resulted in stepwise expression of Sox17 and Pdx-1 which was followed by Neurogenin 3 (Ngn3)-driven regeneration of insulin-producing beta (B) cells (Cheng et al. 775). This mechanism was similar to the patterns observed during the development of the pancreas (Cheng et al. 786). In agreement with research already presented, in both type 1 and type 2 diabetic mice insulin secretion and glucose homeostasis was restored after the FMD cycles (Cheng et al. 775). Type 1 and type 2 diabetes are both characterized by B-cell differentiation whereas B-cell reprogramming, proliferation and/or stepwise re-differentiation has been proposed to be therapeutic (Cheng et al. 775). This suggests lineage conversion of B-cells are essential in diabetes pathogenesis and therapy (Cheng et al.). Thus, when Ngn-3 driven regeneration of B cells occurs during cycles of FMD there is a reversal in B-cell failure and rescue in mice with type 1 and type 2 diabetes (Cheng et al.). In humans with type 1 diabetes fasting conditions inhibits PKA and mTOR pathways to induce Sox2 and Ngn expression which results in insulin generation (Cheng et al. 775). When IGF-1 levels are restored or increased the effects of FMD are reversed (Cheng et al. 786). Similarly when the PKA and mTOR pathways are reinstated (i.e. not inhibited) the effects of FMD are reversed (Cheng et al. 786).
What was learned from Example 1 and what still needs to be done:
- This study has filled in some of the gaps in knowledge left from the Brandhorst et al. article
- The proposed mechanism by which FMD can act as an antidiabetic display the potential for future research
- There is potential to coordinate cell fate through dietary restriction and reverse pathogenesis (Cheng et al. 786)
- Large randomized control trials are still needed to test this FMD for the effects of insulin resistance and B-cell regeneration in the pancreas (Cheng et al. 786)
Example 2: FMD and Multiple Sclerosis
Autoimmune diseases were not something that the Brandhorst et al. article explored, however, there have been promising results pertaining to the effect of FMD on reducing the severity of autoimmune diseases such as multiple sclerosis. Multiple sclerosis is a disorder characterized by demyelination mediated by T cells which leads to neurodegeneration (Choi et al. 2136). 3-day cycles of FMD have been shown to be effective in improving demyelination and reducing the severity of multiple sclerosis (Choi et al. 2136). In multiple sclerosis mouse models suppression of autoimmunity and stimulation of remyelination though oligodendrocyte regeneration reduced the severity of the disorder (Choi et al. 2143). FMD promoted regeneration of the oligodendrocyte precursor which in turn, favoured remyelination The improvements in the mouse models were associated with higher corticosterone levels, higher regulatory T cells (T regs) and lower levels of pro-inflammatory cells such as cytokines, T-helper cell 1 (TH1), T-helper cell 17 (TH17) and antigen-presenting cells (APCs) (Choi et al. 2136).
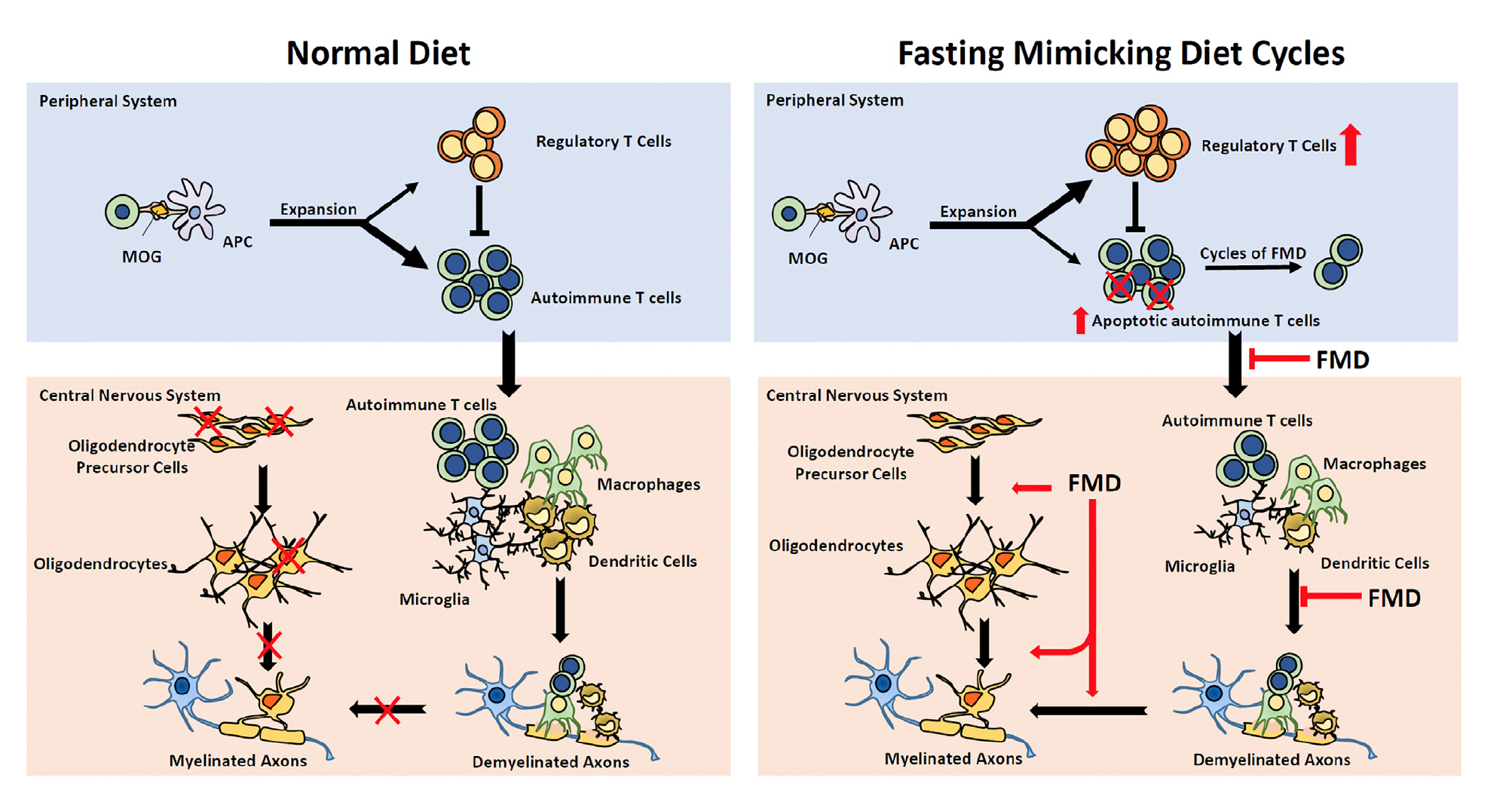
The results proposed by Choi et al. support the anti-inflammatory effects of the FMD which may involve the upregulation of AMP-activated protein kinase (AMPK) or the downregulation of mTORC1 (mammalian target of rapamycin complex 1) (2143). Both upregulation of AMPK and downregulation mTORC1 sense nutrient availability and direct cell fate (Choi et al. 2143). It has been revealed that mTORC1 can couple immune signals with metabolic programming in order to establish T cell function (Choi et al. 2143). Treatment with the mTORC1 inhibitor, rapamycin or the AMPK activator, metformin has actually been shown to alleviate symptoms by modulating effector T cells and Tregs as well as restricting the infiltration of mononuclear cells into the central nervous system (Choi et al. 2143). After looking at this information, Choi et al. propose that FMD may interfere with T cell proliferation and differentiation as well as reducing the number of other immune cells by interfering with their recruitment (2143).
What was learned from Example 2 and what still needs to be done:
- This study goes beyond the scope of the Brandhorst et al. to show further applications of FMD as a therapeutic treatment for multiple sclerosis
- Looking at both example 1 and example 2, mTOR seems to be an important component of the mechanistic pathway of FMD by which FMD reduces risk factors and disease symptoms
- Therefore, future studies can examine the link between mTOR and FMD as mTOR is a factor in many biological processes
Choi et al. and the Feasibility of FMD
Choi et al. also conducted a randomized pilot study to evaluate the feasibility of FMD on patients with multiple sclerosis (2143). The FMD they tested was different from the Brandhorst et al. study in that participant assigned to the FMD diet completed a single cycle of FMD for 7 days which was then followed by a Meditteranean diet for 6 months (Choi et al. 2143). The intervention of the FMD was well received by participants as indicated by the 100% compliance rate (Choi et al. 2143). In 72% patients with multiple sclerosis who were on the FMD, there was a 20% drop in total lymphocyte count which returned back to normal after the switch to the Meditteranean diet (Choi et al. 2143). These results indicate that the FMD may have alleviated symptoms of multiple sclerosis in humans because there was a drop in autoimmune lymphocytes (Choi et al. 2143). It is important to note that in the Choi et al. article the Mediterranean diet post-FMD was not controlled hence it’s difficult to say whether FMD alone can reduce symptoms associated with multiple sclerosis (2144).
This was a promising modification of the FMD that differentiated from Brandhorst et al. who did one 5-day cycle of FMD once a month for 3 months (93). 5% of the participants, in this case, were disqualified for non-compliance (Brandhorst et al. 93). This illustrates the potential for modifications of FMD such as a change in the number of cycles (e.g. 1 vs 3), the length of each FMD cycle (e.g. 5 vs 7) and the post-FMD diet (e.g. normal diet vs Mediterranean diet). Modifications may be easier for patients to adhere to which showcases the potential for future research to incorporate variations of the FMD.
Test your knowledge:
Thinking about the Cheng et al. article and the proposed mechanism by which FMD affects type 1 diabetes in humans, match the images to the correct number in the order by which they occur. (e.g. first = first to occur in the sequence of events). (Hint: try to remember figure 6.6.3)
6.6.3. Closing Remarks
Overall the fasting mimicking diet is a promising new area of study that has potentially beneficial effects on disease reduction and disease risk factor reduction. Further research into the fasting mimicking diet will allow for a better understanding of its effects on biomarkers, risks factors and mechanisms of how FMD influences disease. The potential for therapeutic applications of the fasting mimicking diet provides great avenues of science that are yet to be explored.