14 5.6: Novel Treatments – The Role of Cannabigerol in Neuroprotection
Learning Objectives
After reading this section, you will be able to:
- Describe how CBG can alleviate neuroinflammation
- Understand how certain key apoptosis regulators operate in a cell
- Explain how the researchers analyzed their immunocytochemistry results
There continues to be great scientific interest amongst researchers regarding the Cannabis sativa plant and its role in neuroprotection. In this chapter, we will consider current research that explores this topic.
PAPER REVIEW: In Vitro Model of Neuroinflammation: Efficacy of Cannabigerol, a Non-Psychoactive Cannabinoid
Motivations and Methods
A study published recently by Gugliandolo et. al. focuses on CBG, a non-psychoactive cannabinoid that is found in the C. sativa plant. While CBG is relatively new to this field of research compared to the more widely studied CBD, there has been some evidence from recent literature describing neuroprotective anti-inflammatory and anti-oxidative effects. The objective of this study was to examine the anti-inflammatory and anti-oxidative effects of CBG on a motor neuron cell model that has been implicated in several neurodegenerative diseases, such as Multiple Sclerosis, Amyotrophic Lateral Sclerosis, and Alzheimer’s Disease. The findings from the study have the potential to not only progress the inquiry in this field, but also improve upon current treatments that are only marginally effective in these debilitating diseases.
The researchers obtained CBG from the C. sativa plant to be used in the treatment cohort. Two cell lines were utilized, RAW 264.7 macrophages and NSC-34 motor neurons. The macrophages were activated via LPS exposure. The media from these macrophages were taken and incubated with untreated motor neurons and CBG pre-treated motor neurons. As control treatments, untreated and CBG pre-treated NSC-34 motor neurons were incubated with media from the inactivated macrophages. Western blot and immunocytochemistry assays were used to determine expression levels of apoptosis regulators, inflammatory cytokines, and ROS associated proteins.
Key Results
For this paper, we will focus on four major results that are relevant to this topic:
Result #1: Cell Viability
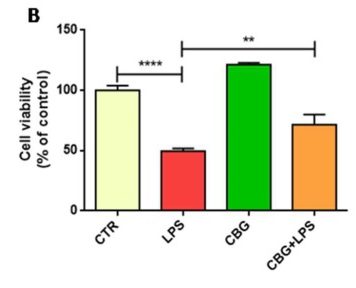
The researchers used the untreated control neurons as a baseline measurement. They found that the untreated neurons had experienced a 50% loss in cells after incubation with the media of LPS-stimulated macrophages. In comparison, the CBG pre-treated neurons experienced significantly reduced cell death, closer to approximately 25%, when exposed to the same medium (Figure 6.1).
Result #2: Expression of Apoptosis Regulators
Several proteins were evaluated in this study for their expression levels in each of the cell cohorts. Many of these proteins are involved in apoptosis regulation. Caspase 3, the first protein of interest, is an endoprotease involved in progressing the cellular death cascade in mammalian cells (see: Death By Executioner Protein). Caspase 3 is a zymogen that becomes active when cleaved, so the researchers used a Western Blot experiment to determine if active caspase 3 was present in the multiple cohorts. They were able to determine that the control cohorts (in which there was no exposure to activated macrophages) did not present cleaved caspase 3, which was expected (Figure 6.4). The untreated cells that had been exposed to the media from activated macrophages, however, displayed a dramatic increase in cleaved caspase 3, indicating that these cells would undergo apoptosis (Figure 6.4). The CBG pre-treated neurons also displayed cleaved caspase 3 when exposed to this media, but to a significantly decreased amount. GAPDH was used in the experiment as a control and was displayed in a graphical format in a ratio with levels of cleaved caspase 3 to quantify the data (figure 6.4).
YOU CAN(NABIS) LEARN MORE…
Death by Executioner Protein: Caspase 3
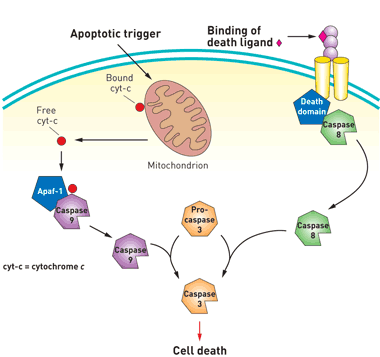
The caspase gene family are a group of proteins that are involved in the induction of apoptosis in mammalian cells. Caspase 3, frequently named the executioner caspase, is an endoprotease that cleaves a significant number of downstream proteins to cause cell death. In a normal cell, caspase 3 remains inactive in the form of its zymogen, pro-caspase 3. Pro-caspase 3 can be activated by the extrinsic and intrinsic pathways. The extrinsic pathway involves signalling from specific receptors on the cell surface. For example, TNF-alpha, an inflammatory cytokine, can bind to its receptor on the plasma membrane and activate adaptor proteins that lead to the dimerization of the initiator caspase 8. Caspase 8 cleaves pro-caspase 3 in order to make it active. As its name suggests, the intrinsic pathway is induced in response to an internal insult, which results in the leakage of cytochrome c from the mitochondria. This activates the initiator caspase 9, which like caspase 8, is involved in the cleavage event of pro-caspase 3. Once active, caspase 3 cleaves several cellular proteins that are vital for cell survival, ultimately leading to apoptosis.
Next, the researchers looked at expression levels of two key apoptosis regulatory proteins. Bax and Bcl-2 both belong to the Bcl-2 gene family. Bax is a pro-apoptotic protein that induces mitochondrial instability. In a normal cell, Bax remains in the cytosol and is tightly regulated by pro-survival proteins from the Bcl-2 family. When initiator caspases are activated, Bax relocates to the outer mitochondrial membrane and begins to form pores. This leads to leakage of mitochondrial contents, such as cytochrome c, which activates the intrinsic pathway. Bax expression in the untreated neurons exposed to the macrophage media is significantly increased, with just over 80% positive staining (Figure 6.3, 6.4). In contrast, CBG pre-treated neurons have near zero levels of Bax expression, similar to the control cohorts (Figure 6.3, 6.4).
On the other hand, Bcl-2 is a pro-survival protein that has many anti-apoptotic functions, one of which includes the regulation of Bax. Bcl-2 is highly expressed in both control cohorts and only in the CBG pre-treated neurons (Figure 6.3, 6.4). However, it is completely absent in untreated neurons that had been incubated with this medium, indicating here that these cells are strongly up-regulating the pro-apoptotic pathway (Figure 6.3, 6.4).
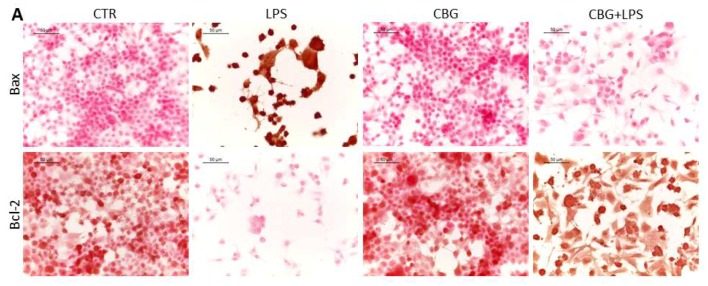
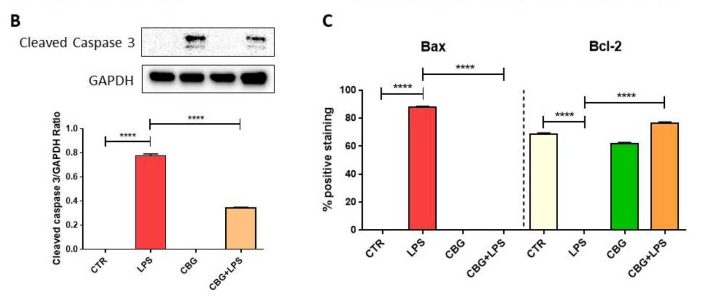
Result #3: Inflammatory Mediators
Activated macrophages produce a plethora of pro-inflammatory mediators that can contribute to tissue damage. The researchers next decided to examine expression levels of these mediators in the different cohorts. Interleukin-1β, tumour necrosis factor-α, and interferon-γ are proteins that are involved in boosting the immune system in response to an insult and can activate many pathways, including the most well–known, NFκB pathway, in surrounding cells.
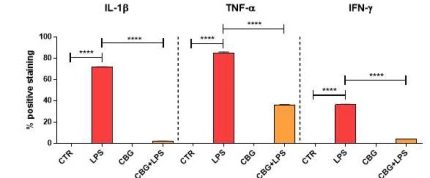
By using immunocytochemistry, they were able to determine that the untreated neurons had significant increases in these pro-inflammatory mediators when incubated with media from LPS-stimulated macrophages, as compared to negligible levels in the control cohorts (Figure 6.5). In comparison, while there was evidence of these mediators in the CBG pre-treated cells, they were found to be at drastically lower levels as compared to the untreated macrophage exposed cells (Figure 6.5). The presence of these mediators in the pre-treated neurons was not considered significant when compared to the control cells.
Result#4: Oxidative Stress
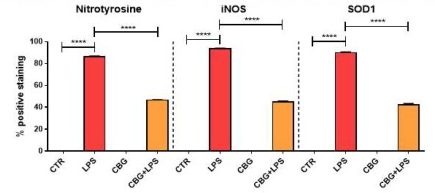
The final key result from this paper describes potential anti-oxidative effects of CBG in the brain. Mammalian cells naturally produce reactive oxygen species (ROS) during the process of ATP production. Reactive oxygen is a free radical, which means that it has an unpaired electron that can react with and oxidize almost anything inside the cell. This reaction can be very harmful and can destroy vital molecular processes, structures, and genetic material inside of the cell. While ROS are absolutely necessary for the elimination of invading microbes, they are often also responsible for peripheral tissue damage. In inflammatory models, macrophages produce molecules such as iNOS as part of the innate response and are key markers of oxidative stress. The researchers evaluated 3 of these markers found in the media of activated macrophages; iNOS, as mentioned earlier; nitrotyrosine, another free radical; and SOD1, a protein that converts harmful superoxide to molecular oxygen and hydrogen peroxide. They were able to determine that the untreated NSC-34 neurons experienced a significant increase in each of these markers when incubated with the medium of activated macrophages. In contrast, the expression of these markers in the CBG pre-treated neurons was found to be reduced as compared to the untreated (Figure 6.6).
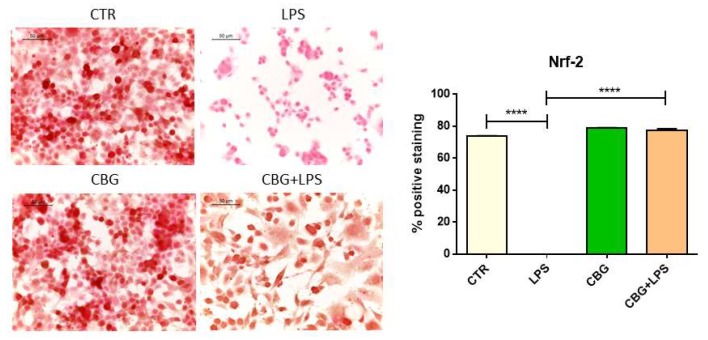
They also examined the expression levels of the transcription factor, Nrf-2. Nrf-2 is a key protein that has many functions within the cell, one of which includes the suppression of oxidants. Nrf-2 activates genes involved in superoxide conversion (i.e. SOD1), reducing factors, and other anti-oxidant proteins. The untreated neurons that had been incubated with the media from LPS-stimulated macrophages displayed a significant loss of this protein, whereas the CBG pre-treated neurons in the same conditions displayed a recovery of this protein to similar levels as the controls (Figure 6.7).
Critiques
The authors of this paper have provided substantial supporting evidence in order to strengthen their conclusions. In all of the experiments, controls had been well established and logical in order to prevent any misinterpretation of the results. Also, the researchers evaluated several hallmarks of most neurodegenerative diseases, including proteins that are involved in inflammation and oxidative stress. However, there were also some key areas that this study could have been improved upon.
An important limitation of this study was that it was conducted on in-vitro samples. While in-vitro studies allow for the administration of unique treatments to cells in a controlled environment, it can be difficult to assign significance to the results when they are collected outside of the original system.
Another possible drawback of the study is in regards to the overall experimental design. The researchers chose to treat the NSC-34 motor neurons with CBG before exposure to the media from LPS-stimulated RAW 264.7 macrophages. The main results were able to support that this pre-treatment confers protection against inflammation, and while this conclusion is valid, it may not fully address what is required to treat the disease. As with most disorders, treatment would normally begin at symptom onset, which makes it difficult for a pre-treatment to be implemented. For example, in the case of Multiple Sclerosis (MS), clinically relevant symptoms may not be present until long after lesions have accumulated in the brain and spinal cord. While this treatment may prevent or delay further neurodegeneration, it may not have an effect on the neurons that have already deteriorated enough to produce symptoms.
The final important limitation of this study was the lack of adequate discussion on the authors’ part, regarding one of the results. Along with the inflammatory cytokines, the authors had utilized immunocytochemistry to determine expression levels of the peroxisome proliferator-activated receptor (PPARγ). The PPARs are a family of nuclear receptors that can affect the transcription of a wide variety of genes. PPARγ, in particular, is more prominent in adipose and vascular tissue. However, its importance has more recently been explored in neurons. Current research on this receptor has indicated that when activated by agonists in the brain, PPARγ can slow down the progression of neurodegenerative diseases by decreasing inflammation and cell death.
In this study, one would expect (based on the inflammatory mediator and cell viability results) for PPARγ to be highly expressed in the CBG pre-treated neurons when exposed to the media. However, the opposite result was seen here. The untreated cells expressed significantly high levels of PPARγ, whereas the CBG pre-treated neurons displayed drastically lower
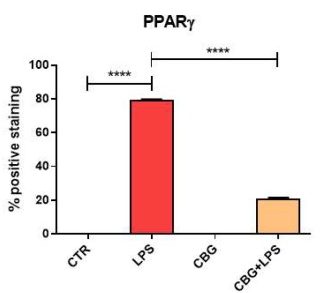
levels of PPARγ (Figure 6.8). This is a baffling result, as the prediction could be made that the reduction in inflammation and increase in cell viability would be, in part, due to a neuronal receptor that has been shown to play a role in cell survival in models of neuroinflammation. Moreover, TNF-α is a known negative regulator of PPARγ, therefore decreased levels of this cytokine may lead one to expect an increased expression of PPARγ.
Nonetheless, many predictions can be made, but it is the responsibility of the researchers to interpret whatever the results may be, to the best of their ability. What is most concerning about this is that the authors do not provide any explanation or discussion as to why this may have occurred. They simply state that, in this case, CBG treatment decreased levels of PPARγ and that derivatives of this cannabinoid may increase its expression. This ambiguous account of the data can lead to confusion regarding the conclusiveness of the results and its overall impact on the study.
Future Directions
There are several ways in which the authors and other researchers can use this study as a base to further expand upon. Firstly, in-vivo studies can be conducted in mice that model neurodegenerative diseases. For example, transgenic mice with the SOD1G93A mutation are popular models used to replicate ALS. Another good example is the use of experimental autoimmune encephalomyelitis or EAE mice models to study MS. Replicating the results from this study using in-vivo models will strengthen the conclusions made by the authors.
Moreover, in-vivo studies are vital to understanding how treatments can be delivered more effectively. For example, the delivery and correct dosage depend on how effectively the medicine can pass through the blood-brain barrier. This and many other obstacles need to be taken into consideration and tested before any conclusive treatment can be achieved.
Lastly, it may be useful to study any effects that CBG can have on motor neurons that have already experienced deterioration. While it is absolutely important to prevent further degeneration, there is still a hope for a treatment that can “turn back the clock” and restore motor neuron function. Ultimately, if there is some regenerative effect that can be determined from such studies, it is possible that the use of CBG as a treatment could better the lives of the many who live with these diagnoses.
Challenge Yourself!