5.1 Chemical Hazards
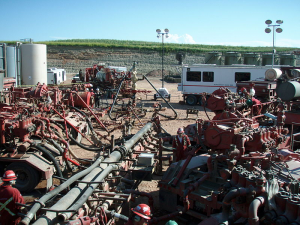
Story: Lawsuit over hydraulic fracturing (“fracking”)
In the spring of 2015, the Supreme Court of Canada agreed to review a decision made by the Alberta courts in a lawsuit brought forward by Jessica Ernst against Alberta’s energy regulator. Ernst filed a suit against the province and Calgary-based energy company Encana over the contamination of her groundwater by hydraulic fracturing.[1] Hydraulic fracturing (or ‘fracking’) is a petroleum-extraction process wherein workers drill deep holes and then inject fluid into the ground under high pressure to fracture rock layers and thereby recover otherwise inaccessible petroleum. The occupational and environmental risks associated with fracking are significant and complicated.
Each fracking effort can require up to 8 million gallons of water and 400,000 gallons of fracking chemicals. Wells can be fracked up to 20 times. Fracking fluid contains water, sand, and various chemicals. When researchers examined the 632 chemicals known to be used in fracking, they found that 75% of them negatively affect the skin and sensory organs as well as the respiratory and gastrointestinal systems. At least 40% are believed to negatively affect the brain and/or nervous system, immune system, cardiovascular system, and kidneys. And 25% are believed to cause cancer and other mutations.[2]
Workers can be exposed to these hazards while fracking. Yet the chemical hazards of fracking don’t just endanger workers. Like most chemical hazards, they also endanger the general public. For example, fracking chemicals can enter the local water table (which often serves as the source of local drinking water). Leakage can occur along the fissures caused by the fracking, from the well casings (which often pass through local water tables), and from inadequate storage of fracking wastewater. Ernst, for example, alleges that fracking northeast of Calgary has resulted in so much methane entering her well that she can now light her drinking water on fire.
Fracking also causes earthquakes and releases airborne chemical hazards. Drilling the well site alone can release “benzene, toluene, xylene and ethyl benzene (BTEX), particulate matter and dust, ground level ozone, or smog, nitrogen oxides, carbon monoxide, formaldehyde and metals contained in diesel fuel combustion—with exposure to these pollutants known to cause short-term illness, cancer, organ damage, nervous system disorders and birth defects or evendeath.”[3] Workers on site and individuals passing or living nearby are affected by these chemical hazards.
Fracking is but one example of the growing threat that chemical hazards pose to the health of workers. It also demonstrates that there is no clear division between a workplace hazard and an environmental hazard. There is no comprehensive list of chemical substances that workers may be exposed to in the workplace, but the number is suspected to be at least 80,000. As we will see below, there is toxicological data available for about 1% of these chemicals, and the data that is available is highly suspect. The essentially unregulated nature of chemical exposures in the workplace is an important argument for adopting the precautionary principle in occupational health and safety.
Chemical Hazards
Chemicals are everywhere in the modern workplace, from printer toner to engine exhaust to sink cleaners. While most chemical exposures do not cause ill effects, some certainly do. As we saw in Chapter 3, chemical hazards cause harm to human tissue or interfere with normal physiological functioning when they enter our bodies. Some chemicals irritate our tissue while others poison our systems or organs. Chemicals can asphyxiate us or negatively affect the functioning of our central nervous systems. Chemicals can also cause our immune systems to overreact, change our DNA, cause cancer, or damage a fetus.
There are four routes of entry by which chemicals can get into a worker’s body, the most common being through respiration (i.e., breathing in contaminated air) and absorption through the skin. Chemicals can also enter our bodies through ingestion (i.e., we can eat them—usually accidentally) and through cuts in our skin. Our bodies excrete some chemicals in our sweat, exhaled breath, urine, or feces, while retaining other substances. Our bodies metabolize some chemicals into other substances, which may be more or less toxic than the original substance.
Chemical hazards have varying levels of toxicity (i.e., ability to cause injury). Toxicity can be local or systemic. Local toxicity is a reaction at the point of contact. For example, you might experience a burn on the skin of your fingers after handling spicy peppers in a restaurant kitchen. Systemic toxicity occurs at a point in the body other than the point of contact. Allergic reactions after prolonged exposure to latex would be an example of systemic toxicity (see Box 5.1). Another example might be organ damage following skin absorption of a pesticide while picking fruit.
Contact dermatitis among food service workers
Many food service workers cope with a chronic rash on their hands. This dermatitis is caused by exposures to chemical substances such as cleaners and food products as well as by frequent handwashing—all of which can irritate a worker’s skin. Workers can develop severe itching, burning, flaking, cracking, blistering, and bleeding of their hands. Over time, repeated exposures to chemical substances can also make workers allergic to those chemicals. Allergic reactions mean workers can develop symptoms on other parts of the body. There are over 1000 workers’ compensation claims for dermatitis in Ontario alone each year. [4]
Other factors appear to play a role in food service workers’ propensity to develop dermatitis. Extreme temperatures (such as hot dishwater and serving dishes as well as cold freezers), mechanical trauma (such as friction, pressure, abrasions, and lacerations) and biological agents (such as bacteria on meat and vegetables) are common food service hazards. Each of these hazards can increase the likelihood of workers developing dermatitis.[5]
Some food service workers wear latex gloves as a form of PPE in order to reduce their contact with chemical substances. Latex gloves are also widely used by health care workers. Ironically, latex gloves themselves contain multiple chemicals (called rubber accelerators). These chemicals have allergenic properties and may contribute to the skin damage that gives rise to dermatitis. Workers can also become allergic to the latex gloves themselves, an allergy that can subsequently be triggered by household, recreational, medical, and clothing items. Proper skin care combined with eliminating or reducing exposures to the chemical, physical, and biological hazards of food service is likely to be more effective in reducing the incidence of dermatitis.
Acute toxicity represents the immediate harm caused by exposure to a chemical substance. Chronic toxicity represents a substance’s ability to cause harm over a longer period of time. The time between exposure to a chemical hazard and the development of symptoms from that exposure is called the latency period. Many of the consequences of exposures to chemical hazards (e.g., occupational diseases) have a latency period that is measured in years. As we saw in Chapter 2, this delay can confound the relating of diseases to occupational exposures.
Although only a fraction of all chemical exposures result in a worker’s death, toxicity is often measured in terms of a substance’s lethal dose (LD) as determined from animal experiments. For example, the toxicity of a chemical tested on rats via ingestion might be expressed as Oral LD50 (rat): 56mg/kg. What this means is that when rats were fed the substance, half (the ‘50’ after the LD) died shortly after ingestion when given 56 milligrams of the substance per kilogram of animal weight. These LD50 values are measures of substances’ acute toxicity and allow us to compare the toxicity of substances. Substances with a lower LD50 are more acutely toxic than substances with a higher LD50 because lower LD50 substances cause half of the animals to die at lower doses. The toxicity of substances may also be measured based upon their lethal concentration (LC) in the air or water.
These toxicity measures show us that the dose (or amount) of a chemical that enters the body affects whether the chemical exposure causes harm and the degree of harm. For example, some chemicals are relatively harmless in low concentrations, such as the methane gas found in Jessica Ernst’s well water. But, in high concentrations, methane can displace oxygen and cause rapid heart rate, fatigue, nausea, and, eventually, death by asphyxiation. (It is also flammable and potentially explosive.) That said, it is important to note that doses that are too low to cause acute toxicity can still cause chronic toxicity, especially if the dose is repeated over time. Prolonged exposure to silica dust, for example, can give rise to silicosis—a lung disease that impedes respiration—but silicosis may not manifest itself for 10 to 30 years after the exposure.
While toxicity data is helpful in identifying chemical hazards, it is important to be cautious when using it. Lethal dose measures focus on the acute toxicity of a substance and are less useful in assessing a substance’s chronic toxicity or the effect of repeated exposures to low doses. Toxicity experiments also tend to be based upon ingestion of the substance because ingestion-based experiments are less expensive than experiments based upon respiration or contact. This bias may reduce the accuracy of the resulting data because most chemicals enter our bodies through respiration or skin absorption. Toxicity data is also based upon animal experiments, and these results may not be perfectly applicable to humans. Perhaps most concerning is that toxicity experiments typically assess the toxicity of a single substance in isolation. This ignores the reality that most workplaces expose workers to multiple chemicals and these exposures may interact synergistically. That is to say, exposures to multiple chemicals may increase the toxicity of each chemical out of proportion to its toxicity in isolation.
As discussed in Chapter 3, controlling chemical hazards begins by identifying worker tasks and environmental factors associated with the location. Subsequently, we must identify and list each chemical a worker is exposed to and the route(s) of entry for that chemical. The potential hazard posed by each exposure and the risk of exposure should be determined along with control strategies. Control strategies used should follow the hierarchy of controls, beginning with elimination (e.g., using non-chemical processes) and substitution (e.g., using a less hazardous chemical), then progressing to engineering controls (e.g., physically isolating workers from the chemical). [6]
Less effective control approaches include administrative controls that minimize or standardize exposures and the provision of personal protective equipment (PPE). In addition, some workplaces provide special facilities (e.g., showers, lunch rooms) to minimize workers’ exposure to chemicals. Some organizations will also undertake extensive medical and environmental monitoring and record keeping. This can include monitoring the level of a hazard in a specific area area, the dose experienced by a worker (personal monitoring), or the presence of a chemical or its metabolic residue in a worker’s blood, body fluids, or tissues (medical monitoring). While not hazard controls per se, monitoring and record keeping can provide data that can help to adjust administrative controls, assess the effectiveness of PPE, and identify early signs of health effects.
In practice, controlling exposure to chemical substances can be difficult. Workplaces often use multiple chemicals, which may have poorly documented synergistic effects. Further, the ways in which products are used may change over time, thereby reducing the effectiveness of administrative controls such as exposure and handling protocols. For example, a reduction in the number of cleaning staff in a hotel may mean workers must now work faster because their workloads have increased. Prior to the staffing change, workers may have used one chemical product to clean toilets and, subsequently, another product to clean the bathroom floors. To cope with the reduced time the workers are given to clean the entire bathroom, the workers may begin applying both products at the same time, creating the possibility of hazardous chemical interactions. Such a change in practice may be unknown to the employer. This example demonstrates that health and safety can be profoundly affected by other human resource practices, such as job design, staffing, and scheduling.
- Canadian Press. (2015, April 30). Jessica Ernst’s fracking case to be heard by the Supreme Court of Canada. CBC. http://www.cbc.ca/news/canada/calgary/jessica-ernst-s-fracking-case-to-be-heard-by-supreme-court-of-canada-1.3055627 ↵
- Colborn, T., Kwiatkowski, C., Schultz, K., & Bachran, M. (2012). Natural gas operations from a public health perspective. Human and Ecological Risk Assessment, 17(5), 1039–1056. ↵
- Hoffman,J.(2015).PotentialHealthandEnvironmentalEffectsofHydrofrackingintheWilliston Basin, Montana. Geology and Human Health. http://serc.carleton.edu/NAGTWorkshops/health/case_studies/hydrofracking_w.html ↵
- Morra-Carlisle, M. (2012, August 23). Service industry hazards getting under workers’ skin. Canadian Occupational Safety. http://www.cos-mag.com/Hygiene/Hygiene-Stories/service-industry-hazards-getting-under-workers-skin.html ↵
- Centres for Disease Control and Prevention. (2012). Skin exposures and effects. http://www.cdc.gov/niosh/topics/skin/ ↵
- Government of Alberta. (2011). Best Practices Guidelines for Occupational Health and Safety in the Healthcare Industry. Edmonton: Author. ↵
Workplace hazards potentially giving rise to injuries caused by a chemical substance that harms human tissue or interferes with normal physiological functioning.
The four ways chemicals can get into a workers’ body: inhalation, ingestion, skin absorption, and skin penetration.
The ability of a substance to cause injury.
Reaction to a toxic substance reaction at the point of contact.
Reaction to a toxic substance at a point in the body other than the point of contact.
Irritation of the skin that often begins with a rash and can lead to severe itching, burning, flaking, cracking, blistering, and bleeding.
The immediate harm caused by exposure to a chemical substance.
Harm caused by exposure to a substance that manifests itself over a longer period of time.
The time between exposure and the development of symptoms from that exposure.
The amount of a substance required to cause death upon ingestion, thereby quantifying a substance’s acute toxicity and allowing us to compare the toxicity of substances.
The amount of a substance in the air or water required to cause death.
The amount of a chemical that enters the body.
An increase in an effect (e.g., toxicity) caused by two chemicals interacting.
Measuring the dose experienced by a worker.
Measuring the presence of a chemical or its metabolic residue in a worker’s blood, body fluids, or tissues.