Locomotion Lab Outline
Introduction
C. elegans
Caenorhabditis (see-no rab-dye-tis) elegans (C. elegans) is a species of non-parasitic, non-pathogenic roundworms (Phylum: Nematoda). The life cycle of the nematode can be seen in figure 1. Nematodes are a model organism used in research labs around the world, including here at McMaster University. This includes the Nematode Biodiversity Project (Dr. Dej; School of Interdisciplinary Science), studying developmental biology and regulation of gene networks (Dr. Bhagwati Gupta; Department of Biology) and how age-related disorders develop (Dr. Leslie MacNeil; Department of Biochemistry and Biomedical Sciences).
When placed on nematode growth media (NGM) plates, C. elegans move in response to environmental cues using rhythmic, undulating and oscillating body motions that resemble a sinusoidal wave-like pattern (figure 2). The particular pattern of movement is a result of the coordination by the neuromuscular system. The nervous system of C. elegans is made up of 302 neurons. These include sensory neurons, which detect changes in environmental conditions and relay signals to central processing centers. These processing centers contain interneurons that are able to integrate sensory information with motor neuron activity. It is then the direct control of muscle activity by 75 motor neurons that coordinates the undulating movements of the worm. Being able to coordinate movement seems to be a complex task for such a small, 1-1.5 mm long organism, but imagine scaling the control of locomotion to a human, who can have as much as 100 billion neurons in the body!
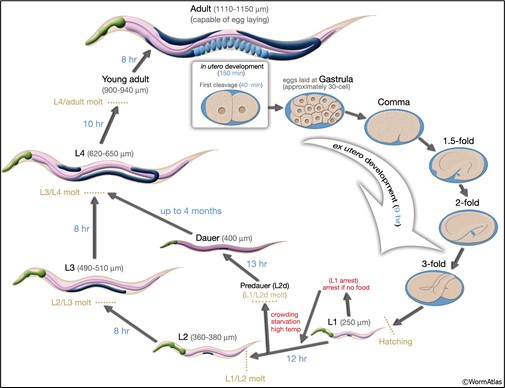
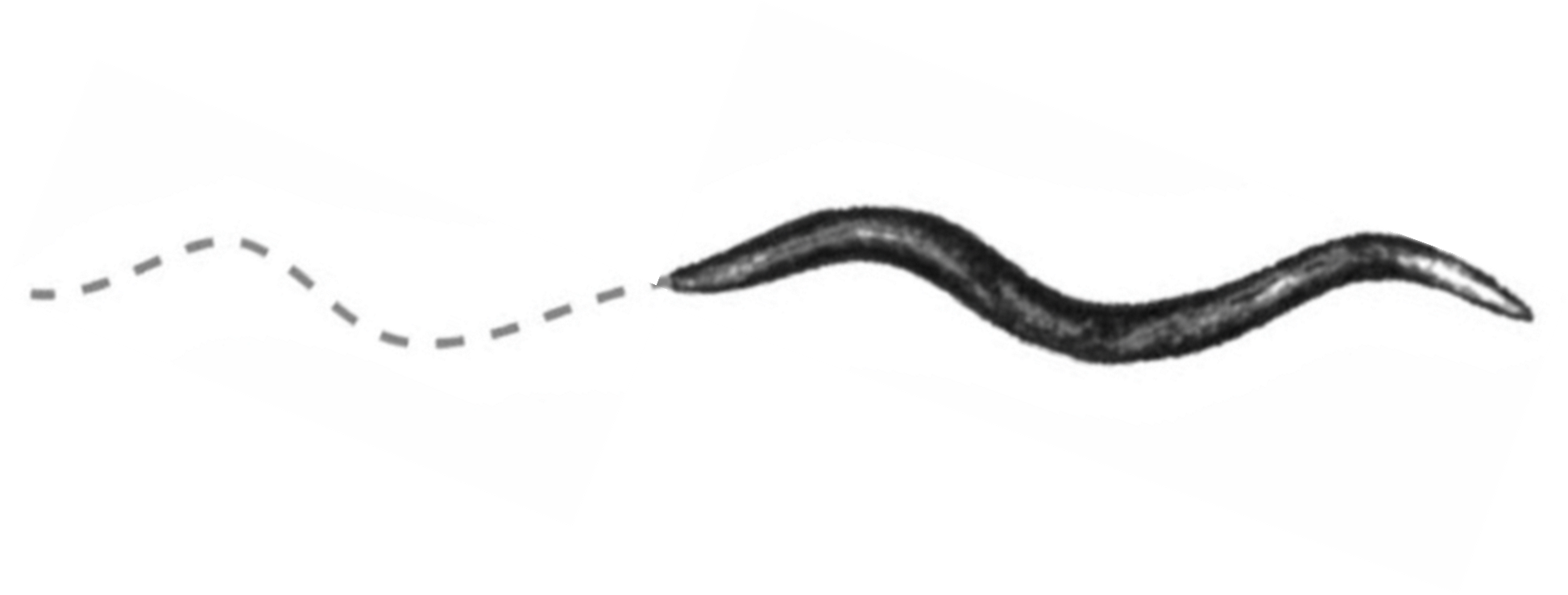
One of the unique aspects that makes C. elegans a great model organism is that we can investigate the coordination of physiological processes (such as movement) and correlate this knowledge with how humans regulate the same processes. Being such small organisms that can be easily manipulated also makes C. elegans a great model to investigate the effects of aging and aging-related disorders on neuromuscular activity. This is easy to do since there are mutant or knockout strains for almost every C. elegans gene available to order from work repositories (e.g. Caenorhabditis Genetics Center) or from research labs which have engineered their own strains. This enables us to identify how gene products correlate to their function, and also enables us to investigate how physiological processes (such as movement) can be affected with disease and aging.
Protein Aggregation
Aging is a complex biological process that involves changes in molecular and cellular behaviours that in turn impact tissue and system health and function. Cellular aging is accompanied by an increasing number of toxic protein aggregates in the cells. These aggregates are correlated with various neurodegenerative disorders, such as Alzheimer’s, Huntington’s, and Parkinson’s disease. Researchers are trying to identify how protein aggregation might cause the observed cellular and tissue-level phenotypes seen with these diseases.
Modelling Protein Aggregation in Living Systems
Protein aggregation can be studied using a number of biological model systems, including the C. elegans. The strains we are using today have been developed by the Morimoto lab at Northwestern University. These strains produce fluorescently labelled protein aggregates in the muscle cells of its body wall (figure 3). The effect of knocking down various proteins (e.g. through RNA interference; RNAi) on the size and amount of these aggregations can be used to identify proteins involved in the unfolded protein response, which is crucial for understanding these neurological diseases. However, there are limitations to using this C. elegans model for studying neurological disease pathology for a number of reasons. For instance, the protein aggregations in these disease states actually occur in neurons, not in the muscle cells like we see in this model. However, this is still a good model for studying the unfolded protein response (UPR) which is activated in response to an accumulation of unfolded or misfolded proteins in the lumen of the endoplasmic reticulum, and how dysfunction of this response can affect protein aggregations that contribute to these neurological diseases.
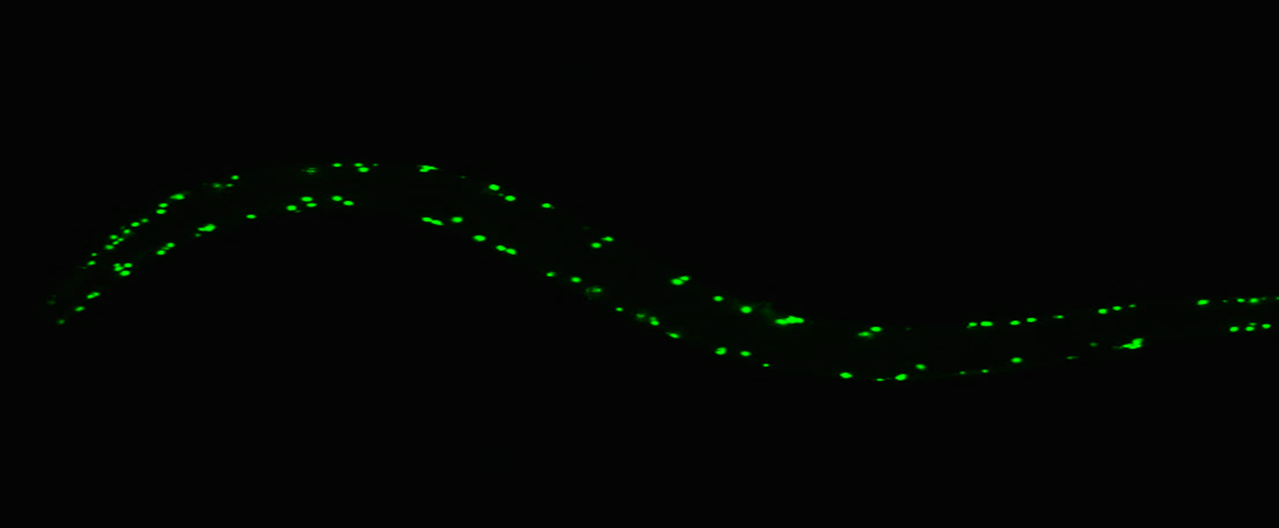
The video below is from a different strain of C. elegans that contains a green fluorescent protein (GFP) in the muscle cells. You can observe these organisms under a stereomicroscope. In this video, C. elegans movement is being influenced by chemotaxis – movement of the organism triggered by following chemical gradients in the surrounding environment.
LSL Video Locomotion – C. elegans
Investigating UPR and protein aggregation disorders with C. elegans
The AM138 (Q24), AM140 (Q35) and AM141 (Q40) strains are being used in the lab today. These strains carry a yellow fluorescent protein (YFP) fusion under the regulation of a muscle-specific (myosin heavy chain) promotor (unc54p). This means that the YFP localizes to just the muscle cells of the organism, as that is where the protein is expressed in the nematode. In the Q0 strain of nematode, the proteins tagged with YFP show a diffuse (soluble) distribution throughout the body wall muscle cells their whole lives. However, the construct in the AM141 strain (“Q40”; unc54p::Q40:YFP) also contains a poly-glutamine repeat (polyQ), Q40 (N=40 glutamines) fused in frame with YFP. Immediately after hatching, there is a soluble distribution of the polyQ:YFP protein in the muscle cells of the body wall. As the nematode ages, these polyQ:YFP proteins form aggregates within the muscle cells of the body wall, which affects its locomotion. The number of aggregates is proportional to the poly-glutamine expansion length. There are actually dozens of these varying strains, each engineered with a different length of aggregation – fluorescent images of 9 of these strains are shown in figure 4, including the Q35 and the Q40.

These worm strains are often utilized to study how aging can contribute to physiological accumulation of protein aggregates and cellular toxicity. This models the various neurodegenerative and neuromuscular diseases including Alzheimer’s, Parkinson’s, amyotrophic lateral sclerosis (ALS), and Huntington’s diseases. This is a model only, because the expression of these protein aggregates is in the muscles, where we would find these aggregates in the neurons in the human diseases.
Interestingly, the potential for aggregate formation with increased poly-glutamine repeats is not only regulated by a muscle-specific promotor (e.g. as seen in the AM141 (‘Q40’) strain). There are strains available that contain the poly-glutamine repeats that are induced for expression by neuron-specific promoters. Aggregate formations in these neuron cells are similar to the aggregate formation phenotype observed in muscle cells. Then this provides further evidence that wildtype and mutant strains of C. elegans can be used to model both neurodegenerative and neuromuscular disorders.
Table I provides a summary of the genotype and phenotype of these 3 mutant strains we are specifically using today.
Table I: Description of Nematode Strains Used in the 2L03 Lab. Strain ID corresponds to the ID# for ordering this strain from the Caenorhabditis Genetics Center (CGC).
Strain ID | Genotype | Phenotype |
---|---|---|
AM 138 "Q24" | rmls130 [unc-54p::Q24::YFP] | Diffuse distribution of Q24::YFP throughout body-wall muscle cells. Worms maintain a soluble phenotype during their lifespan. |
AM140 "Q35" | rmls132 [unc-54p::Q35::YFP] | Animals show a Q35::YFP progressive transition from soluble to aggregated as they age. |
AM141 "Q40" | rmls133 [unc-54p::Q40::YFP] | Animals show a soluble Q40::YFP distribution in their body wall muscle cells immediately after hatching. However, as the nematodes age, foci (aggregated protein) are observed. When they reach adulthood, animals show an entirely Q40::YFP aggregated phenotype. |
You’ll want to watch the video about how to use the Zen Stemi305 stereomicroscope before coming to lab this week – this microscope is different from a compound light microscope in that the you’ll retain 3-D view of the materials you are working with (with a compound light microscope, you are reduced to a 2-D plane that you are viewing, and the image has no depth of field). You may also hear stereomicroscopes called “dissecting” microscopes, as they facilitate microdissection activities on small organisms like nematodes or fruit flies, which would not be possible with normal human vision! Please refer to Appendix E: Zeiss Stemi305 Stereomicroscope to learn about the operation of the stereomicroscope.
Lab Overview and Timeline
Time (min) | What will you be doing? | Total Time (min) |
---|---|---|
0 - 10 | Overview of lab activities by the TA. | 10 |
10 - 50 | Lab Part 1: Mechanosensory Response Assay Lab Protocol 1 - Mechanosensory Response Assay |
40 |
50-90 | Lab Part 2: Thrashing assay using C. elegans Lab Protocol 2: Collecting your worms Lab Protocol 3: Capturing Videos of Nematodes during Thrashing Assay Ensure all data is in the class dataset by the 90 min mark. |
40 |
90-170 | Clean up your workstation Complete and submit your ELN in PAIRS |
80 |
Lab Part 1: Mechanosensory Response Assay (‘touch-test’)
Purpose
To practice manipulation of objects under a stereomicroscope, and collect data regarding behavioural differences in various nematode mutations to observe the differences between organisms related to protein aggregation in the muscle cells of the body wall.
Summary of Activities
You will be conducting a mechanosensory response assay with the same mutant strain of nematode you used from Part 1. This time, you’ll be using nematodes still on the surface of their NGM agar plate, and you will touch the nematode on the head with a fine paintbrush and observe whether they retreat away from the stimulus (what is called a “backing” response) or if they do not react. This will provide an indicator of the functionality of the mechanoreceptors in the organisms in response to stimuli.
Form two pairs at your stations. Each pair will work together with one petri dish of nematodes. Record your data in table II.
Materials
A. Plastic transfer pipettes
B. plate of nematodes – will be ONE of three types of nematodes used by you and your partner
- Q24 nematodes (AM138)
- Q35 nematodes (AM140)
- Q40 nematodes (AM141)
C. empty plates for conducting experiments
D. M9 buffer jar
E. P20 pipette tips
F. P1000 pipette tips
G. 15 ml conical tubes for collecting nematodes in Part 2
H. micro-brush for Part 2 (THESE ARE REUSABLE! DON’T THROW AWAY!)
I. permanent marker for labelling plasticware
J. P1000 micropipette
K. P20 micropipette
Not shown in image:
- Stemi305 Stereomicroscope
- Computer set up with Zeiss Zen software
- tips waste container
- liquid waste container
- Benchtop centrifuge (the TA will operate this today)
- cell counters
- stopwatches
Safety Reminders
There are potential risks associated with these lab activities that you should be aware of, including but not limited to:
-
- The food source for nematodes is E. coli (OP50) which is found on the agar layer of the petri dish you get the nematodes from. Use caution working with the nematode plates and M9 buffer of nematodes, wearing gloves and ensuring not to touch your personal belongings or face with your gloves!
- Centrifuges are extremely dangerous if not properly loaded. Your TA will be supervising centrifuge usage in this lab, and the centrifuge should never be turned on if not properly balanced.
Lab Protocol 1: Mechanosensory Response Assay
LSL Video Locomotion – Mechanosensory Response to micro-brush contact
- You will select a petri dish with NGM media and nematodes in it. Make sure you record which strain of nematodes you will be working with today (e.g., Q35).
- You’ll be removing the lid of the petri dish for this activity, so you can interact with the nematodes on the plate. Do this before you start adjusting the focus, or you’ll need to adjust again when the lid comes off.
- Place the NGM plate under the stereomicroscope and adjust the coarse focus and magnification until you can see several nematodes.
- Increase the magnification until you are viewing one specific adult nematode. You can move the plate around under the field of view to adjust as required.
- You will not be using the computer/microscope camera for this part, because the field of view through the eyepieces is much easier to work with for this activity.
- You will use the micro-brush and gently brush the bristles across the head of the nematode you are viewing. Observe the response, and give the nematode about 20s to recover from the poke.
- How can you tell which end of the nematode is the head? Watch it move a bit – the nematode will naturally be travelling head-first, with it’s tail dragging along behind it.
- Repeat this touch test four more times, recording the response each time.
- Generate a score for this nematode /5, where the nematode receives 1pt for each backing away in response to the paint brush poke, and 0pts for each time it does not respond. Record this score in your downloaded copy of Table II.
- Move the NGM plate a bit and locate a different nematode. Repeat the touch test again, poking the nematode on the head 5 times, with rests in between, and recording a score /5.
- Complete the touch test for 5 nematodes on your NGM plate, recording the scores as you go.
Lab Part 2: Thrashing assay using C. elegans
Purpose
To practice working with the stereomicroscope and Zen software, and to experience working with a model organism and scoring behavioural data related to muscle-localized protein aggregation.
Summary of Activities
You will use the stereomicroscope and Zen software to capture short (~30s) videos of the Q24, Q35 and Q40 nematodes moving in a droplet of M9 buffer. You will then count the number of body motions for each worm during an elapsed time of 30 seconds. This will allow you to investigate the effects of age-related disorders (specifically, polyQ-repeats) on the locomotion of C. elegans. Record your data in table I.
Materials
- Same as part 1
Lab Protocol 2: Collecting your worms
- Using a p1000 micropipette, wash the surface of the petri dish with 0.5 ml of M9 buffer 4 times, transferring the liquid into a 15 ml conical tube each time. Be careful not to overfill the plate!
- NOTE: you should swap tips on the pipette after each wash. This is so you don’t put worms in the M9 buffer jar when you get your next 0.5 ml out!
- This 15 ml conical tube now contains the nematodes that were on the surface of the NGM plate, in addition to 2 ml of M9 buffer. You will label your tube with your pod name, and take your tube over to the TA to centrifuge.
Why are we centrifuging our nematodes? Won’t that hurt them?
-
- Nope! The forces being applied during this centrifugation are not high enough to damage the organisms. Instead this will create a “pellet” of worms in the bottom of the conical tube, leaving the liquid buffer on top.
- When the TA gives you back your tube, take it back to your pod. You will use the plastic transfer pipette to remove the extra M9 buffer on top of the pellet, leaving ~1 ml of buffer in the tube with the worm pellet.
- Now, the worms need to be resuspended! Use the plastic transfer pipette to gently pipette the remaining M9 buffer up and down over and over again, creating turbulence to mix up the worms in the pellet with the buffer. You’re creating a HOMOGENOUS solution of worms and buffer.
Lab Protocol 3: Capturing Videos of Nematodes during Thrashing Assay
- Using the P20 micropipette, you will draw up 10 μl of resuspended worms and place this droplet onto a fresh petri dish (not containing any agar).
- Place the petri dish under the stereomicroscope. Bring the droplet into focus, increase the magnification as required, and identify how many adult worms you have within the droplet. You can refer back to Figure 1 to refresh your memory about the life stages of the nematode.
- If there are too many worms – add another 10 μl of M9 buffer to dilute your droplet.
- If there are not enough worms – add another 10 μl of the resuspended worm solution to increase the number of worms in the droplet.
- Connect the stereomicroscope camera to the computer, and open the Zen software.
- Place 1 (or more) adult nematodes in the field of view of the microscope camera on the software. Ensure the live view of the camera is in focus.
- Following the instructions for recording videos using Zen (see Appendix F: Zen Software). The relevant part of this appendix for this protocol is part 5. You will capture 30s of video for each organism, using the stopwatch to measure the time you are recording.
- Save the file with a descriptive title so you remember the organism strain information, as well as your pair info so you can easily identify the video afterwards. Be sure to stick to B/W videos, or the files will get too large!
- You can skip saving the videos as files if you just watch back the video in the Zen software. Ensure you’ve recorded a 30s video exactly using the stopwatch, and then you can count the thrashes within Zen instead.
- Open the video file on the computer, and watch the video through, recording how many FULL body thrashes take place in the elapsed 30 seconds. If the video is longer than 30s, you won’t count thrashes after this time.
- A full body thrash is when the nematode swings it’s body from the midline all the way to the left, back through the midline, all the way to the right, and back to the midline.
- You can use the counters to help record thrashes while you watch back the video.
- Record the number of body thrashes for your organism in Table I.
- Repeat this process for 5 worms. Note: You may have more than one worm in a single video, so you can double up on data for that recording, counting the thrashes for one worm first, then counting the second worm, giving each of them their own value in your list of 5 recorded worms.
- When you are done collecting your data, you will add your 10 values to the class dataset. Clean up your station, turning off the microscope and putting the nematode plate you were using for this assay in the discard pile on the central lab bench – these plates are now empty, so they won’t be reused, and we’ll be bleaching them to disinfect them before disposal. The micro-brush should be sprayed with 70% EtOH (recall, there is OP50 E. coli on the plate, so we want to disinfect the paint brush), and the bench top can be sprayed and wiped down as well. Do NOT throw away the micro-brush!
- Once all data is collected, the TA will post the class data set on Avenue for you to begin your analysis. We will be aiming to complete the class data spreadsheet by the 90 minute mark – if students have not completed their data collection by this time, we’ll move on with the partial dataset so student pairs have time to complete the graph for the ELN.
LSL Video Locomotion – C. elegans movement in water
(Video by Clint Reynolds, YouTube, 2012.)
Let’s consider the experimental design of today’s lab a bit!
What are the differences between the replicate measurements taken in the two activities today?
- Thrashing Assay:
- We counted the number of thrashes in 30s for 5 unique nematodes.
- Each of these nematodes was the same mutant strain in your personal data tables (although different pairs worked with different strains, to allow for data to be collected for all 3 strains for the class dataset)
- Mechanosensory Response Assay (touch-test):
- 5 unique nematodes were assessed by each pair of students
- For each unique nematode, the touch-test was repeated 5 times, to generate a score out of 5
Technical Replicates: these are replicates taken within the same biological sample (this could be a cell, a human, a cat, a nerve, etc.) They show if there is any variability of the protocol. An example of this was when you did the aging and frailty chair stand test multiple times, or when you counted the cells from your cell suspension ten times, using each of the grid squares across the two sides of the haemocytometer.
Biological Replicates: these are the same type of measurement but in different biological samples. These biological samples can be considered to be part of the same treatment group for this definition here, but this isn’t a perfect rule for the full field of science! An example of this was in the epidemiology lab, where you ran each NetLogo simulation multiple times.
Treatment Groups: Treatment Groups should receive a different experimental intervention between them, with the rest of the variables controlled. When you design an experiment, you want to consider to what extent both technical and biological replicates will play a role within each of your treatment groups. (E.g., how many mice will be in each treatment group? How many times will you test each mouse? How many treatment groups are there?)
Can you identify which type(s) of replicates were used for each of the two experiments today?
With your partner, consider the following experimental design features of today’s class data set:
- What is the hypothesis/prediction for each experiment? (i.e. what are we expecting we will see when we analyze the class data set during the ELN?)
- What is the independent variable? (Was it something intrinsic to the organisms, or was it something related to the procedure we executed?)
- What is the dependent variable? (Note: there are two, since there were two experiments with different endpoints!)
Lab Exit Checklist
- Thrashing assay scores collected for 5 nematodes from your pair, using the Zen software, and recorded in your personal copy of Table I
- Touch-test scores collected for 5 nematodes from your pair, using just the stereomicroscope (no videos for these!), and recorded in your personal copy of Table II
- Scores from Table I and Table II transferred to the class dataset for each assay. NOTE: the mutant you collected this data from is very important, please don’t forget to indicate this in the class dataset
- Clean up your station for the next group of students:
- All tips waste into the tips waste container
- Any waste buffer in the liquid waste container
- Plastic transfer pipettes and petri dishes used for assays in the solid waste container on the TA bench (we need to bleach these before disposal)
- Gloves in biowaste box (yellow bag cardboard box)
References
Altun, Z.F., & Hall, D.H. (2009). Introduction. In WormAtlas. doi:10.3908/wormatlas.1.1
Brignull, H.R., Morley, J.F., Garcia, S.M., & Morimoto, R.I. (2006). Modeling polyglutamine pathogenesis in C. elegans. Methods in Enzymology. 412: 256-282.
Gjorgjieva, J., Biron, D., & Haspel, G. (2014). Neurobiology of Caenorhabditis elegans locomotion: Where do we stand? BioScience, 64(6): 476-486.
Morley, J.F., Brignull, H.R., Weyers, J., & Morimoto, R.I. (2002). The threshold for polygluatmine-expansion protein aggregation and cellular toxicity is dynamic and influenced by aging in Caenorhabditis elegans. PNAS, 99(16): 10417-10422.
Nussbaum-Krammer, C.I., Neto, M.F., Brielmann, R.M., Pedersen, J.S., &Morimoto, R.I. (2015). Investigating the Spreading and Toxicity of Prion-like Proteins Using the Metazoan Model Organism C. elegans. J. Vis. Exp. 95(e52321). doi:10.3791/52321
Volovik, Y., Marques, F.C., & Cohen, E. (2014). The nematode Caenorhabditis elegans: A versatile model for the study of proteotoxicity and aging. Methods. 68(2014): 458-464.