FRC and Intrinsic PEEP
Going back to the balloon analogy, consider the process of blowing up a balloon. At what point is the balloon the hardest to blow up?
- When it is completely empty? or
- When there is a little air in it already?
An empty balloon requires very powerful and sustained breath to start inflating; but if there is a little air in the balloon already, you can blow it up with a gentler breath and you wouldn’t have to push so hard.
The lungs are no different. The initial little bit of air in the lungs requires a higher amount of pressure in order to open up the lungs a little, while a lung with some air can inflate much more easily. This is where the human body is so smart. The body stops the lungs from fully emptying—it keeps a small amount of air in the lungs at all times. This is referred to as the functional residual capacity (FRC) of the lungs. A term for the air left in the body at the end of exhalation is the intrinsic (physiologic) Positive End-Expiratory Pressure (PEEP). PEEP is very important, as it protects the lungs from the high blowing pressures that would normally be needed to inflate them from empty—decreasing the risk of trauma.
Key Takeaways
The lungs never fully empty. Some air remains in the alveoli at all times. This is known as FRC or intrinsic PEEP. Positive End-Expiratory Pressure (PEEP) refers to the pressure left in the lungs at the end of exhalation—also known as the residual air left in the balloon to stop it from fully collapsing.
Object Lesson
Think for a moment about a balloon that you have reused for three different parties. The first time you blew it up, the rubber seemed sturdy, but over time, it became more fragile and compromised. The fourth time you tried to blow it up, the balloon popped even though it was not yet full. What happened here? The high pressure damaged the balloon over time. In the same way, your lungs can be damaged by consistent exposure to high pressure.
The main physiologic mechanism to achieve FRC is surfactant, a naturally produced lubrication that coats all the alveoli of the lungs. Surfactant stabilizes the surface of the alveoli so they do not collapse and helps facilitate the reserve of the FRC we explained earlier.
Object Lesson
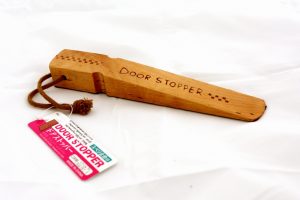
You can think about surfactant as the body’s own “door stop.” It keeps the door slightly ajar so the latch doesn’t close. In technical terms, surfactant decreases the surface tension on the alveoli. Surface tension is the force—just like the elastic of the balloon that wants to deflate the alveoli fully during expiration.
Pressures
When dealing with breathing, it is important to have a basic understanding of the resting pressure changes in the lungs during inspiration, expiration and at rest. All pressures are compared to the “baseline” of the open atmosphere. Imagine the pressure at the mouth is equal to atmosphere and is zero.
Now, let’s revisit inspiration and expiration from a pressure differential viewpoint. Remember, the lungs are glued to the diaphragm and the surrounding thorax by the pleural space. Think of this space as a large suction that is all around the lung. Just like the word suction hints at, the pleural space is a negative pressure at rest. Before inspiration occurs, the pleural space negative pressure (-5 cm H2O) keeps a small amount of air in the lungs (equal to +5 cm H2O) that cancels out the negative pressure in the lungs. This equalization stops the flow of air in or out and puts the lungs in a “rest state” until the diaphragm contracts and causes a negative pressure.
Key Takeaways
Since the pleural space has a negative pressure, to equal zero, air must be left in the lungs!
As the diaphragm contracts, the negative pressure gradient from the dropping of the diaphragm creates a pressure gradient for air to flow in. As soon as the diaphragm relaxes and stops the suction, the volume of air and therefore pressure in the chest is now higher than the pressure at the mouth. This state reverses that pressure gradient and air flows out of the lungs–in other words, exhalation occurs–until the pressures equilibrate.
Media Attributions
- Door Stopper © Chengings is licensed under a CC BY-NC (Attribution NonCommercial) license
- End Expiration and During Expiration lung pressures © Encarta Encyclopedia, used under Fair Use is licensed under a All Rights Reserved license