3.1.1 – Introduction to Nucleophiles, Electrophiles and Curved Arrows
In organic chemistry, chemical reactions occur through the breaking and forming of bonds. Since a bond is a pair of electrons associated between nuclei, the flow of electrons in a reaction process can be used to represent the breaking and forming of bonds. Below (Figure 3.1.1.a) is an example of a simple substitution reaction.
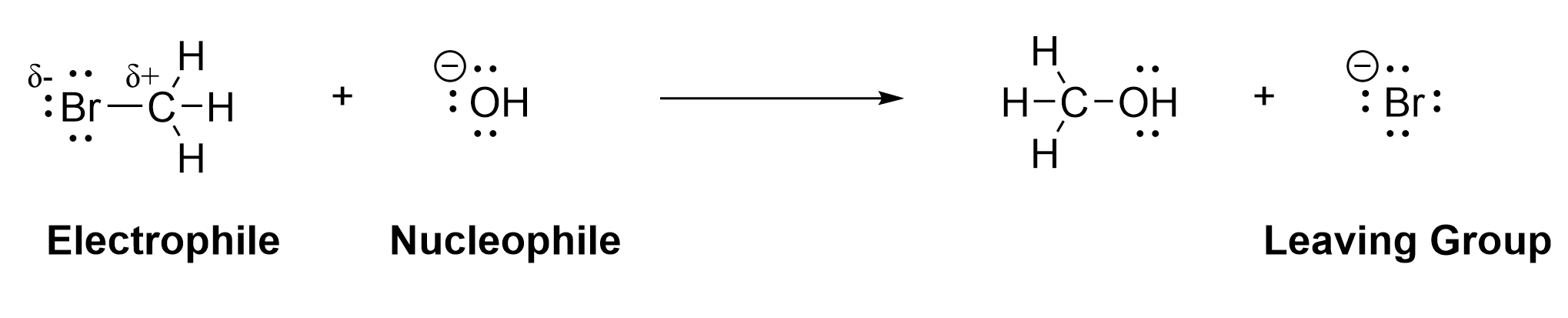
In this reaction, the bromine atom (Br) in the reactant bromomethane (CH3Br) is replaced by the hydroxyl group (OH), making the products methanol (CH3OH) and bromide (Br–). This process involves both the breaking and forming of a sigma bond, and is classified as a substitution reaction. Before we learn the details of this reaction, we must first introduce some key terms that are critical for understanding why and how the reaction proceeds. These terms are electrophile, nucleophile and leaving group.
Electrophile
The reactant CH3Br is classified as an alkyl halide. The C–X bond represents an alkyl halide, where X is a halogen and C is a carbon. Alkyl halides are polar due to the electronegativity difference of the two atoms in the bond, as halogens behave as more electronegative than carbon. Additionally, the difference in atomic radii also contributes to polarity of the bond as halogens tend to be larger and more electron rich atoms. As a result, a dipole moment exists where carbon has a partial positive charge and Br has a partial negative charge. This can be seen in the following diagram:
Because of the partial positive charge on carbon, the carbon atom in the C–X bond is considered electron-deficient. An electron-deficient species, such as an alkyl halide, is called an electrophile, also known as a Lewis Acid (introduced in CHEM 1A03), with the carbon being classified as an electrophilic center. As an electron-deficient center, the carbon atom is capable of accepting electrons from electron donors in a chemical reaction. Electrophiles often have a positive charge, a partial positive charge, and/or an incomplete octet. For example, the carbon atom in CH3Br has a partial positive charge because of the polarity of the carbon-halogen bond. A carbocation is another example of an electrophile, in which the carbon centre has a positive charge and an incomplete octet (6 electrons).
Some common electrophiles are shown below in Figure 3.1.1.b. Notice how all examples contain either a positive or partial positive charge.
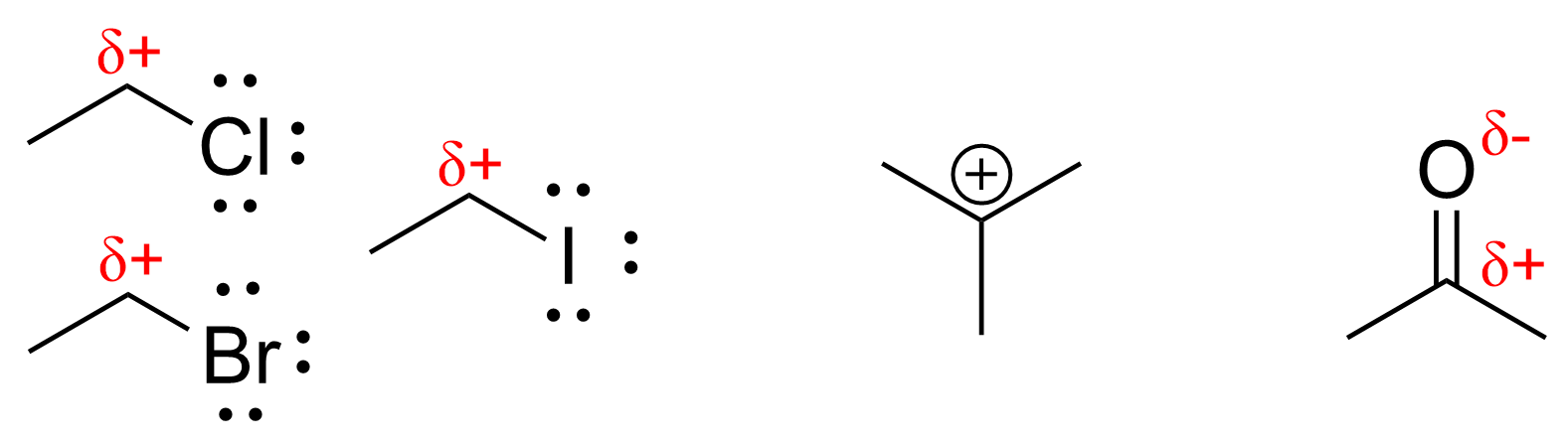
For CH3Br in this reaction, this carbon is referred to as an electrophilic site – the atom that will accept a pair of electrons in a bond forming process.
The entire compound CH3Br that undergoes the substitution is called the substrate. It may help to think of a substrate as just another word for a reagent involved in a reaction.
Nucleophile
The hydroxide anion, OH–, is another reactant in the substitution reaction shown in Figure 3.1.1.a. In the Lewis structure of OH–, the oxygen atom has three lone pairs of electrons and is negatively charged. Due to these factors, hydroxide can be considered an electron-rich species with a high electron density around the oxygen atom.
An electron-rich species, such as hydroxide, is called a nucleophile, with oxygen behaving as the nucleophilic center. Nucleophiles can also be referred to as Lewis base, as they behave as electron-pair donors in bond forming reactions. Nucleophiles seek positively charged or electron-deficient species to form bonds with. In this case, hydroxide is considered the nucleophile and the oxygen atom is what acts as an electron pair donor and is referred to as the nucleophilic site.
Generally, any neutral or anionic species with a lone pair of electrons available to donate can be a nucleophile. Nucleophiles, often written as Nu, can either be negatively charged (Nu:–) or neutral (Nu:). For example, OH–, OR–, H2O, ROH, NH3, RNH2, and RCOO– are all possible nucleophiles due to the presence of at least one pair of electrons. Various nucleophiles are shown below in Figure 3.1.1.c.
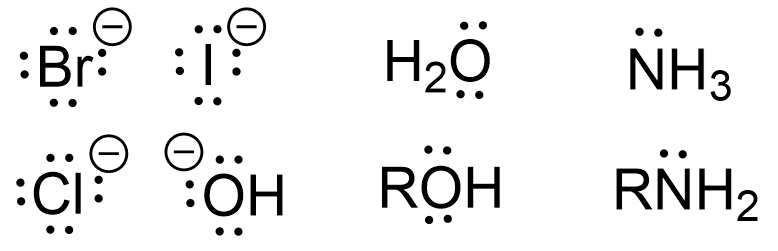
Based on this understanding of nucleophiles and electrophiles, it can be said that when electron-rich nucleophiles meet electron-deficient electrophiles in the correct orientation, bond formation can occur. For the reaction to proceed, these two molecules must collide in the correct orientation which is dependent on the geometry of both species.
Are You Wondering? The Greek Origins of “Electrophile” and “Nucleophile”
The terminology “electrophile” and “nucleophile” actually have from a Greek origin! These words can be split into two parts, which can be used to help remember the reactivity of these species.
The word electrophile is made up of the terms electro and phile, where electro refers to electrons, and phile is the Greek suffix meaning love. Together, they make the word electrophile mean “electron-lover.” This makes sense with what we know, as they are electron deficient species that want more electrons.
Like electrophile, the word nucleophile has two parts: nucleo, meaning nucleus, and phile, meaning love. This word then means nucleus loving. Since the nucleus is made of positive protons, this means nucleophiles love positively charged species.
(The full solution to this problem can be found in Chapter 5.2)
Leaving Group
To ensure the substitution reaction shown in Figure 3.1.1.a occurs, another critical factor is that the C–Br bond must break in order for the carbon to accept a bond from the incoming nucleophile (OH–) without exceeding its octet. The bromide, Br–, is referred to as the leaving group in this scenario. The leaving group (often written as LG) is an electronegative species that leaves with the pair of electrons from the C–LG bond. Without a proper leaving group, even if a nucleophile is electrostatically attracted to an electrophile, the substitution will not occur. Leaving groups can start as neutral and become negatively charged upon accepting the pair of electrons from the bond breaking process, or they can positively charged and become neutral upon accepting the pair of electrons from the bond breaking process. They are not only seen in substitution reactions, but in a number of reactions in organic chemistry.
Applying the three key terms, the above substitution reaction can be summarized as: the nucleophile displaces the leaving group in a substrate. Such a reaction is called a nucleophilic substitution reaction. A nucleophilic substitution reaction can therefore be shown in a more general way:
Note: The nucleophile and leaving group are not necessarily negatively charged, as they could be neutral as mentioned earlier.
Drawing Curved Arrows
Curved arrows are an essential part of organic chemistry, as they showcase what is happening in these chemical reactions at the subatomic level. Curved arrows show the flow of electrons as they move from one region to another. Each curved arrow represents the movement of a pair of electrons.
The tail of the curved arrow always represents the source of the electrons. This can only come from a bond or a lone pair on an atom. The head of the arrow points to where the electrons are going and will also end up either as a lone pair or a new bond. Examples of curved arrows are shown in Figures 3.1.1.d, 3.1.1.e, 3.1.1.f.

The example shown in Figure 3.1.1.d depicts a bond forming curved arrow. The arrow starts at the NH2– anion, which acts as the nucleophile as it has a lone pair that can act as an electron pair donor. It points to the electron-poor electrophile, which contains a positive charge and an incomplete octet. Thus, the arrow shows how the NH2– nucleophile donates a lone pair to form a new covalent bond with the carbon. A good way to remember how to draw curved arrows for a bond forming reaction is: the electrons always move from a species with a high electron density to one with a low electron density.
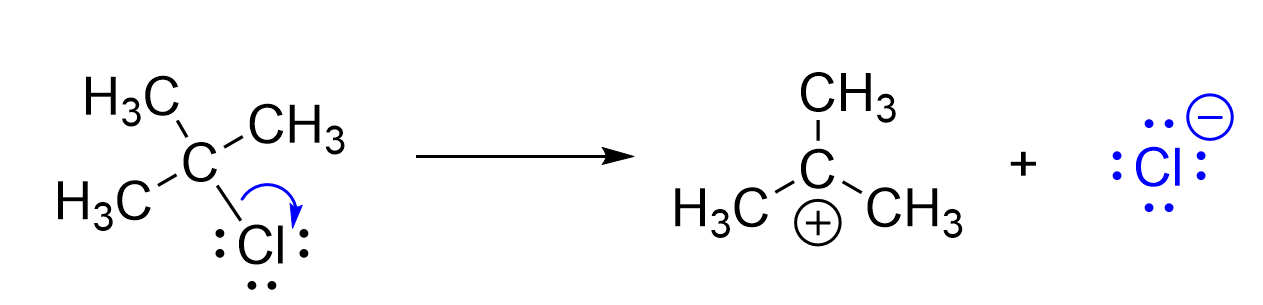
In contrast, the example shown in Figure 3.1.1.e showcases a bond breaking curved arrow. Remember: a covalent bond represents the sharing of two electrons between two atoms, and thus, the electrons can move to form new bonds or to become lone pairs. This arrow starts at a bond, which acts as a source of electrons. The arrow points toward chlorine, indicating the movement of the electron pair to the chloride ion, breaking the bond. When looking at electronegativity trends, it is understandable that the more electronegative chlorine accepts the pair of electrons. As carbon loses an electron, it gains a positive charge, whereas chlorine gains an electron to make an anion.
Let’s apply this new knowledge of curved arrows to the substitution reaction that was shown in Figure 3.1.1.a.
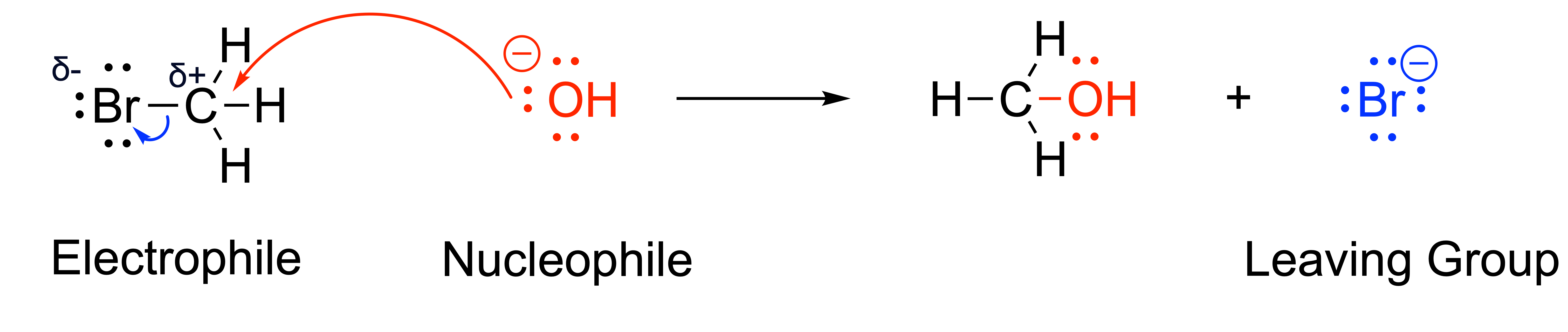
The first arrow, shown in red, is a bond forming curved arrow that depicts the formation of a new bond between the nucleophilic hydroxide and electrophilic carbon. As carbon cannot have more than 8 electrons in its valence shell, it is necessary for carbon to lose electrons elsewhere. Thus, a bond breaking curved arrow is also drawn between the polar bond of C–Br, where the electrons move from the bond to the electronegative Br. This results in the final product, methanol, and the leaving group, bromide.
When drawing the mechanism of an organic reaction, it is important to never violate the octet rule by exceeding 2 electrons around hydrogen or 8 electrons around carbon, nitrogen or oxygen. Incomplete octets around carbon atoms can occur in certain scenarios that we will explore in this text. If a bond is forming that leads to more than 8 electrons residing on C, N, or O, then this atom must lose electrons elsewhere. This is often seen in reaction mechanisms where multiple arrows are drawn in one step.
The reaction mechanism between a Lewis Acid and a Lewis Base drawn below (Figure 3.1.1.g) also strongly parallels that of a reaction between a nucleophile and an electrophile.
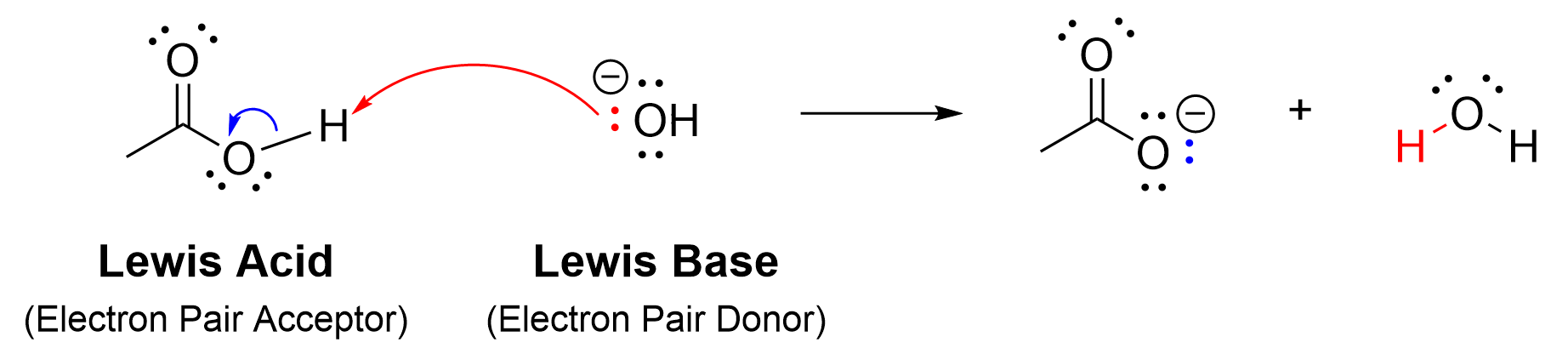
The Lewis Base, hydroxide, functions similarly to a nucleophile, using its lone pair of electrons to donate to the electron acceptor, the Lewis Acid (or electrophile). This will form a bond between the two species. However, hydrogen can only hold two electrons in its valence shell. Thus, for this bond to form, a bond to hydrogen must also break. The blue arrow demonstrates the movement of the pair of electrons from the O-H bond to the oxygen, giving oxygen a negative charge in the product.
Ensure that the curved arrows you draw are clearly double-sided arrows, as these represent the flow of a pair of electrons. Single-sided arrows represent something slightly different, which will be further discussed in second year organic chemistry.
Types of Nucleophilic Substitution Reactions
Now that we have discussed some key terminology, it is important to know that there are two types of nucleophilic substitution reactions, which will be discussed in detail in Chapter 3.1.2 and Chapter 3.1.3. Although the products of these reactions look the same, they both undergo different reaction mechanisms, which was discussed in the kinetics unit. These are:
- The SN2 reaction (see Chapter 3.1.2 for greater detail):
- A second-order reaction that goes through the bimolecular reaction mechanism
- The name SN2 means Substitution, Nucleophilic and Bimolecular.
- The SN1 reaction (see Chapter 3.1.3 for greater detail):
- A first-order reaction that goes through the unimolecular reaction mechanism
- The name SN1 means Substitution, Nucleophilic and Unimolecular.
We will have detailed discussions on SN2 and SN1 mechanisms respectively, and then compare the similarities and differences between them in the upcoming chapters.
(The full solution to this problem can be found in Chapter 5.2)
Key Takeaways
- Electrophiles, nucleophiles and leaving groups are all key components in organic reactions
- Nucleophiles are electron-rich, visible as lone pairs, which attract to electron deficient electrophiles in a reaction; this movement of electrons can be shown using two-headed arrows
- Remember: carbon cannot exceed its octet, so any bond formation to carbon must have the loss of a leaving group to take away two electrons
- Nucleophilic substitution reactions can be one of two types, SN1 or SN2, each involving a nucleophile, electrophile and a leaving group, but differing kinetics and mechanisms
Key terms in this chapter:
Key term | Definition |
Nucleophilic Substitution Reactions | A reaction type which involves the substitution of a leaving group with a nucleophile. The reactants are a nucleophile and an electrophile bonded to a leaving group. The products are the lone leaving group and an electrophile now bonded to the nucleophile. It can be also viewed as the nucleophile “taking place” of the leaving group. |
Electrophile | An electron-deficient species, such as an alkyl halide (C–X). It accepts electrons from nucleophiles (electron donors) in a chemical reaction. These often have a positive charge, partially positive charge, or an incomplete octet. The electrophilic center is the specific atom on the electrophile that is electron-deficient and will accept electrons. |
Nucleophile | An electron-rich species, such as hydroxide (OH-). Nucleophiles seek positively charged or electron-deficient species to form bonds with. Generally, any species, either neutral or anionic, which contains a lone pair of electrons can behave as a nucleophile in an organic reaction. |
Any feedback or comments on this chapter? You may either email chemoer@mcmaster.ca, access this MS Form, or provide a comment in the feedback box below.
An electron-deficient species, such as an alkyl halide (C–X). It accepts electrons from nucleophiles (electron donors) in a chemical reaction. These often have a positive charge, partially positive charge, or an incomplete octet. The electrophilic center is the specific atom on the electrophile that is electron-deficient and will accept electrons.
An electron-rich species, such as hydroxide (OH-). Nucleophiles seek positively charged or electron-deficient species to form bonds with. Generally, any species, either neutral or anionic, which contains a lone pair of electrons can behave as a nucleophile in an organic reaction.
A reaction type which involves the substitution of a leaving group with a nucleophile. The reactants are a nucleophile and an electrophile bonded to a leaving group. The products are the lone leaving group and an electrophile now bonded to the nucleophile. It can be also viewed as the nucleophile “taking place” of the leaving group.