2.5.2 – Alkene Stereochemistry and Nomenclature
Stereochemistry and Nomenclature: Cis/Trans vs. E/Z
The previous section of this chapter introduced the cis/trans designation of alkenes. This refers to the stereochemistry of the molecule, or the relative spatial arrangement of groups relative to a carbon-carbon double bond. In the cis/trans designation, cis refers to similar substituents located on the same side of the double bond, and trans refers to similar substituents on opposite sides of the double bond (Figure 2.5.2.a.). However, cis/trans designation can only be used when there are similar groups on each carbon of the alkene. If the groups on the alkene are different, then cis/trans designation is not useful to denote the stereochemistry of the molecule.
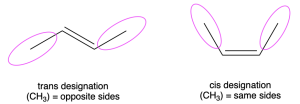
The E/Z designation provides an alternative and more general nomenclature system. This nomenclature system relies on assigning priority to each group bonded to the carbon atoms of the double bond. A designation of E denotes higher priority groups on opposite sides of the double bond, while Z denotes higher priority groups on the same side of the double bond. The letters E and Z come from the German words entgegen (opposite) and zusammen (together).
Because E/Z designation relies on priority of groups, rather than similarity, it is a more applicable system to any scenario. The E/Z system can be used for any alkene, whether the substituents on either side of the carbon-carbon double bond are similar or different from one another. Therefore, E/Z notation has replaced the more traditional cis/trans notation.
For example, Figure 2.5.2.b shows a molecule with different groups attached to the C=C double bond. Cis/trans designation would not be useful for this molecule because none of the substituents are similar, but E/Z designation can be used to denote the stereochemistry of this molecule.
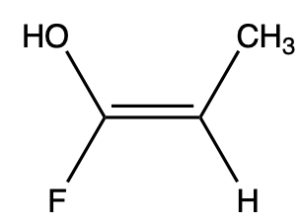
To determine whether a molecule has E or Z configuration, priority must first be assigned to each group using the Cahn-Ingold-Prelog rules.
(The full solution to this problem can be found in Chapter 5.1)
Cahn-Ingold-Prelog Rules
The first step is to cut the molecule through the double bond to create two halves, shown in green and blue in Figure 2.5.2.c.
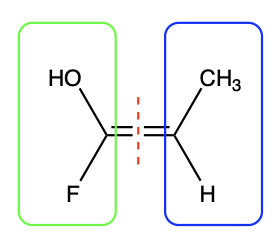
Next, consider the substituents on each half of the molecule (green and blue). Compare the substituents in the green (left) box with each other and compare substituents in the blue (right) box with each other.
Use atomic number to define priority for each pair of atoms that is singly bonded to each carbon in the C=C bond. The atom with the higher atomic number is considered the higher priority group. For example, in Figure 2.5.2.c, in the green (left) box, fluorine has an atomic number 9, compared to oxygen with atomic number of 8. This makes fluorine the higher priority group in the green (left) box. In the blue (right) box, carbon has a higher atomic number than hydrogen, meaning that carbon takes priority in the blue (right) box. The results are summarized in Figure 2.5.2.d, with the higher priority groups shown in red font.
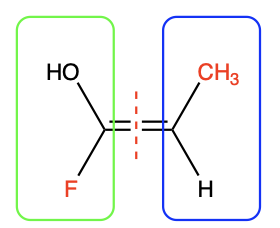
Since the two higher priority groups are on opposite faces, the alkene has the E configuration.
If the groups to be compared are different, but have identical atoms bound to the C=C bond, move on to the next connected atom until a point of difference is found. An example is seen in Figure 2.5.2.e. below.
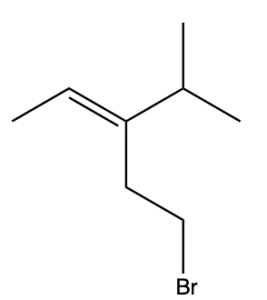
We will follow the same procedure, by first splitting the molecule through the double bond (Figure 2.5.2.f). In the green (left) box, the carbon atom takes priority because it has a higher atomic number than hydrogen. Thus, the methyl group is shown in red font to denote that it is the higher priority group.
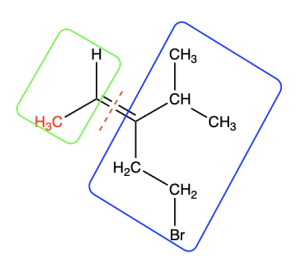
In the blue (right) box, both atoms bonded to the alkene carbon are carbon atoms. They are identical. We need to move on to the next connected atom until we find a point of difference. We compare the three atoms to which each carbon is bonded, in order of decreasing atomic number. Figure 2.5.2.g. illustrates this comparison.
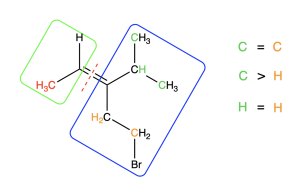
On the top carbon, the atoms shown in green include two carbons and one hydrogen. On the bottom carbon, the atoms highlighted in orange include one carbon and two hydrogens. The top carbon contains more atoms with a higher atomic number than the bottom carbon. Thus, the top carbon takes priority. Since the highest priority groups (highlighted in red on the left and orange on the right) are on opposite sides of the double bond, this alkene has an E configuration (Figure 2.5.2.h). Note that, despite bromine having the highest atomic number in this molecule, the bottom carbon does not take priority, because an earlier point of difference is found before the bromine is reached.
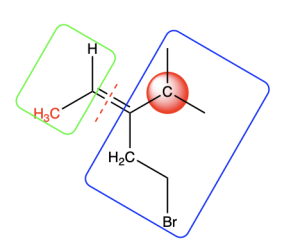
If the second layer does not reveal a point of difference, move on to the next connected atoms and compare until a point of difference is discovered. An example is shown below in Figure 2.5.2.i.
In the green (left) box, bromine takes priority over hydrogen due to its greater atomic number, as shown in red font. In the blue (right) box, the first three layers do not reveal a point of difference, with a series of carbon atoms bonded to another carbon atom and two hydrogen atoms. On the third carbon atom, a difference is observed: one carbon atom is bonded to two hydrogen atoms and a fluorine atom, while the other carbon atom is bonded to two hydrogen atoms and a nitrogen atom. Since fluorine has a higher atomic number than nitrogen, the carbon atom bonded to fluorine takes priority, as shown in red font. The two highest priority groups being on the same side of the double bond means this molecule has Z– designation.
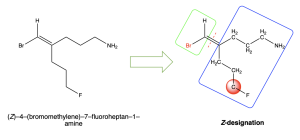
If there is no point of difference, then there is no E/Z designation, because the two stereoisomers (E vs Z) are identical to one another. An example of this is shown below in Figure 2.5.2.j.
In the green (left) box, oxygen has a higher atomic number than carbon so the oxygen atom will take priority, as shown in red font. In the blue (left) box, there is no point of difference: the two groups bonded to the alkene carbon are identical. Since there is no point of difference, this molecule does not have E/Z designation.
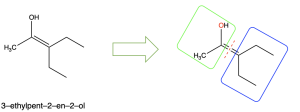
(The full solution to this problem can be found in Chapter 5.1)
Stereochemistry and Cahn-Ingold-Prelog Rules: A Quick Summary
- When presented with an alkene with 3 or more unique substituents, E/Z conformation must be assigned. To do so, the Cahn-Ingold-Prelog rules need to be followed.
- Step to remember when abiding by these rules are as follows:
- Draw an imaginary line through the double bond, splitting the alkene in half. Focus on one half at a time.
- If the two substituents are completely identical on one half, you cannot assign E/Z. Otherwise:
- On the first half of the alkene, there are two substituents. Assign priority to the first substituent atom with the GREATER atomic number. If the substituents bound directly to the double bond are the same atomic number, continue to the next atom of highest priority on both sides of the double bond. Continue until you reach the first point of difference.
- Assign priority to the side with the higher priority atom, which appears closest to the double bond.
- Do this process again on the other side of the split alkene.
- If the two assigned highest priority atoms on each half are on the SAME side, then this is a Z configuration. If they are opposite to each other, then this is an E configuration.
- Draw an imaginary line through the double bond, splitting the alkene in half. Focus on one half at a time.
Are You Wondering? Other Uses of Cahn-Ingold-Prelog Rules
The Cahn-Ingold-Prelog rules are used extensively in organic chemistry, and they will be present if continuing with future organic chemistry courses. They are not only used with alkenes, but also with alkanes when assigning names to groups of isomeric compounds with differing conformations. It is important to differentiate them due to their varying reactivity and properties.
The following video includes a worked example from a previous CHEM 1AA3 test or exam that students struggled with. Try solving it on your own before looking at the solution.
Key Takeaways
- Previously, cis/trans was introduced as a way to designate the stereochemistry of the molecule.
- If there are two of the same functional groups across a double bond and they are positioned opposite to each other, it is denoted as trans
- If they are positioned on the same side, then it is cis.
- However, cis/trans designation is not useful if there are different functional groups across a double bond, so we use the E/Z designation.
- E refers to when higher priority groups are positioned opposite of each other.
- Z refers to when higher priority groups are positioned on the same side.
- To determine priority of the functional groups, use the Cahn-Ingold-Prelog rules summarized above.
Key terms in this chapter:
Key term | Definition |
Stereochemistry | The relative spatial arrangement of groups relative to a carbon-carbon double bond. |
Diversity in Chemistry: John Cornforth
The stereochemistry of alkenes is described in this chapter; however, stereochemistry is applicable to other molecules in organic chemistry as well (a concept which is taught in second year!). John Cornforth was an Australian-British chemist who won the Nobel Prize in Chemistry in 1975 due to his work in stereochemistry. He was able to deduce exactly which clusters of hydrogens would be replaced in a synthetic reaction that used an enzyme as a catalyst. This ultimately paved the way for the development of cholesterol-lowering drugs that are still used today that target the enzymes he studied. Cornforth was diagnosed with otosclerosis at the young age of 10, a condition which deformed the bones within his ears and resulted in full deafness by age 20. Despite so, he excelled in his undergraduate classes, studying through primary literature, and succeeded greatly in his career as he contributed significantly to pharmaceutical research, and also elucidated the full biosynthetic pathway of cholesterol. More information on Cornforth and his journey can be found on his spotlight profile at The Royal Society website.
The relative spatial arrangement of groups relative to a carbon-carbon double bond.