3 Drugs for Treatment of Hyperlipidemia
3.1 Pathology
The previous section examined the importance of clotting factors in maintaining the necessary integrity of the circulatory system and fluidity of blood. However, there can be instances where there are disequilibria of other components such has macronutrients. There are three main macromolecules which include proteins, carbohydrates, and lipids. This section is largely focussed on altered blood lipid concentrations.
Lipids have multiple essential functions in the body:
- Compartmentalization: Every cell in the human body is separated from the external environment by a phospholipid bilayer structure. Additionally, lipids are also used in sub-cellular compartmentalization.
- Energy storage: Lipids contain the highest energy per gram (compared to other macronutrients) and are used as efficient energy storage molecules. Generally, the more reduced a carbon atom is in a biological molecule, the more energy is stored.
- Cellular signalling: Different biochemical pathways rely on the presence or absence of specific lipids, such as the inflammatory response or hormones signalling.
- Dietary absorption: The body uses different lipids (often stored as bile) to aid the digestion and absorption of hydrophobic or fatty substances.
Although lipids are critical for normal physiological functioning, excess cholesterol or fatty acids in the blood can lead to a condition referred to as hyperlipidemia.
3.1.1 Types of Biological Lipids
It is recommended to consume ~20-35% of the total daily caloric intake from dietary lipids. There are three main types of lipids that are also shown in Figure 3.1:
- Triglycerides: These lipids have an invariant glycerol backbone (3-carbon chain with 3-hydroxyl groups) where each hydroxyl group is esterified to a fatty acid. The type of fatty acid that is bound to the glycerol can vary. These molecules are highly non-polar and comprise ~95% of dietary lipid uptake (fats and oils).
- Phospholipids: These are similar to triglycerides in that they have a glycerol backbone. However, phospholipids only have two fatty acid groups and the third group is a phosphate head group. The phosphate headgroup drastically alters the properties of the lipid, in that there is now a highly hydrophilic moiety on one end of the molecule and two lipophilic tails on the other. Phospholipids comprise ~2% of the dietary lipids.
- Sterols: These are compounds based on a cyclopentaphenathrene ring (3 six membered rings and 1 five membered ring) and comprise approximately 3% of dietary lipids. In mammals, the most commonly known sterol lipid is cholesterol and all steroid-based hormones and bile acids are synthesized from cholesterol.
FIGURE 3.1 Representative structures of a generic fatty acid and the three main types of lipid macromolecules.
Lipids are very different from the other macromolecules in that they are largely hydrophobic and do not associate with water. Considering that the human body is ~70% water and that lipids are essential for every single cell in the body, transporting them across the body in the aqueous environment of blood is challenging because of the hydrophobicity. Therefore, lipids are transported in a complex particle comprised of both proteins and lipids, which are referred to as lipoproteins. The structure of a lipoprotein is shown in Figure 3.2. Lipoproteins have an outer shell of phospholipids intercalated with different proteins (referred to as apolipoprotein), and the inside is occupied with triglycerides and cholesterol. These lipoproteins travel through the blood stream and facilitate the transport of lipids.
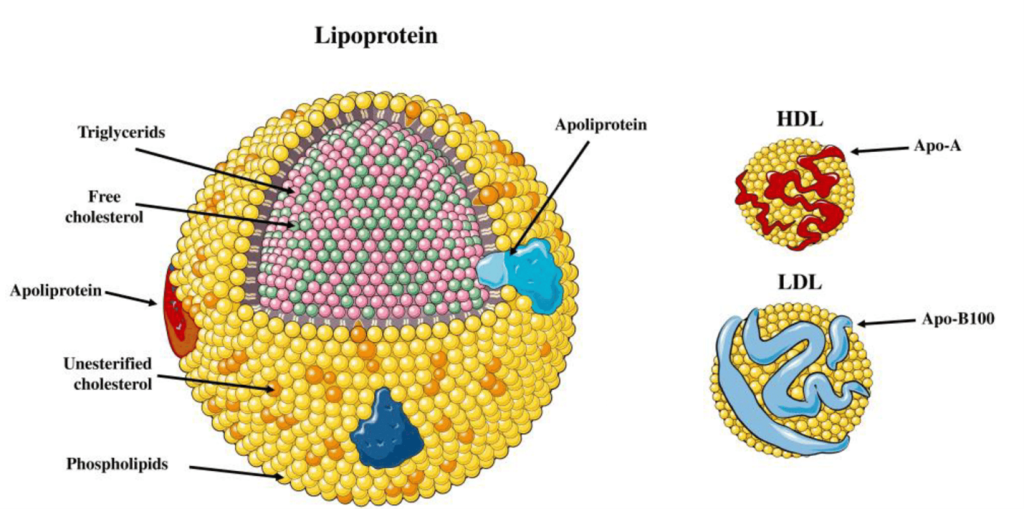
Lipoproteins have different names based on their composition and density. (Figure 3.3) Lipids that are high in protein content are referred to as high density lipoproteins (HDLs) and if they have intermediate, low, or very low densities of lipoproteins they care called IDL, LDL, and VLDL, respectively. HDLs are also relatively small in diameter (5-10 nm) compared to LDL and VLDL which are 20 – 50 nm. There are also larger classes of lipoproteins called chylomicrons which are important for digestion and absorption of fats. Colloquially, the HDLs are often thought of as “heathy cholesterol” and the LDLs and VLDLs are referred to as the “unhealthy fats”. From a general sense, larger particles have higher lipid content and lower stability.
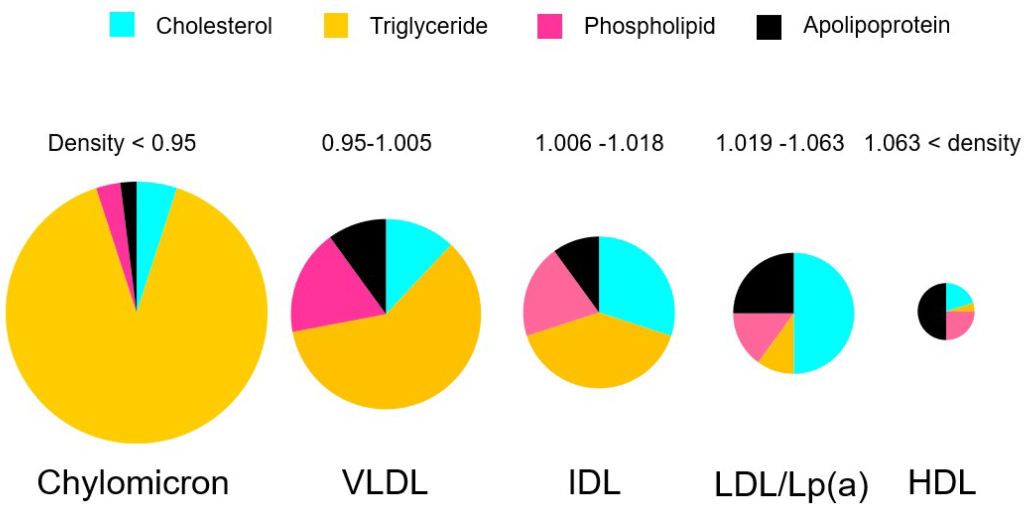
Hyperlipidemia occurs when there is an excess of lipids leading to a high concentration of LDL and VLDL. These fats can precipitate out of the blood stream and start to nucleate on the arterial walls and form a blockage of blood flow. (Figure 3.4) These blockages are referred to as plaques and reduce the arterial lumen, leading to a reduction in blood vessel elasticity, and create a nucleation site for additional thrombi. The primary goal of treating hyperlipidemia is to reduce concentrations of LDL and VLDL.
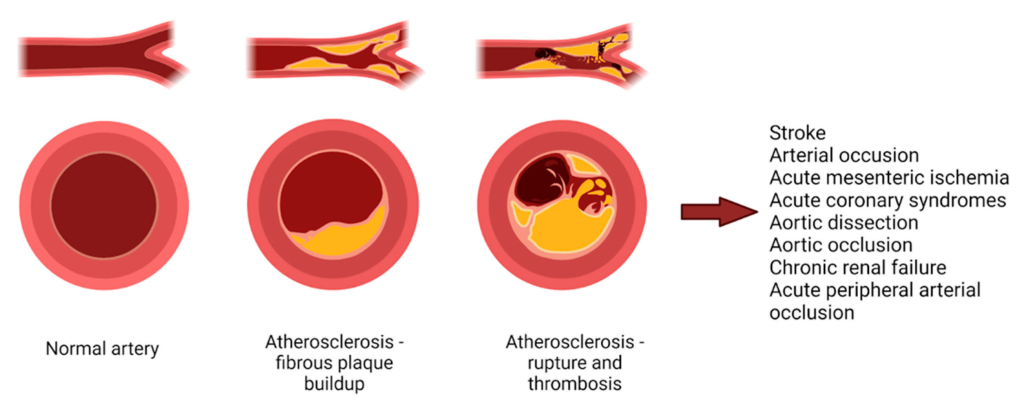
3.2 Treatments
One approach to treating hyperlipidemia is to initially reduce dietary intake of fats and cholesterol and increase cardiac exercise. Although this is important, ~80% of cholesterol is synthesized by the liver and only ~20% is obtained from dietary sources. Therefore, altering diet and exercise may be insufficient and reduction of cholesterol may require pharmacological intervention. There are three main strategies to reducing blood lipid concentrations: inhibit lipid synthesis, inhibit lipid absorption/uptake, or accelerate lipid degradation/clearance. Each of the therapeutic approaches below fit into one of these three themes.
3.2.1 Inhibiting Lipid Synthesis: Statins
The statins are a class of cholesterol-reducing agents that are the most prescribed drugs, and therefore it is important to have a thorough understanding of their mechanism of action. The statins are designed to block cholesterol synthesis. Cholesterol synthesis occurs in the liver through the mevalonate pathway, which is ~25 step biochemical pathway that begins with acetyl-CoA. One of the key features of the pathway is the rate limiting step which is the reaction that converts HMG-CoA to mevalonate. This reaction is carried out by the enzyme HMG-CoA reductase. Therefore, if this enzyme is pharmaceutically intercepted, the mevalonate pathway and cholesterol synthesis can be effectively shut down.
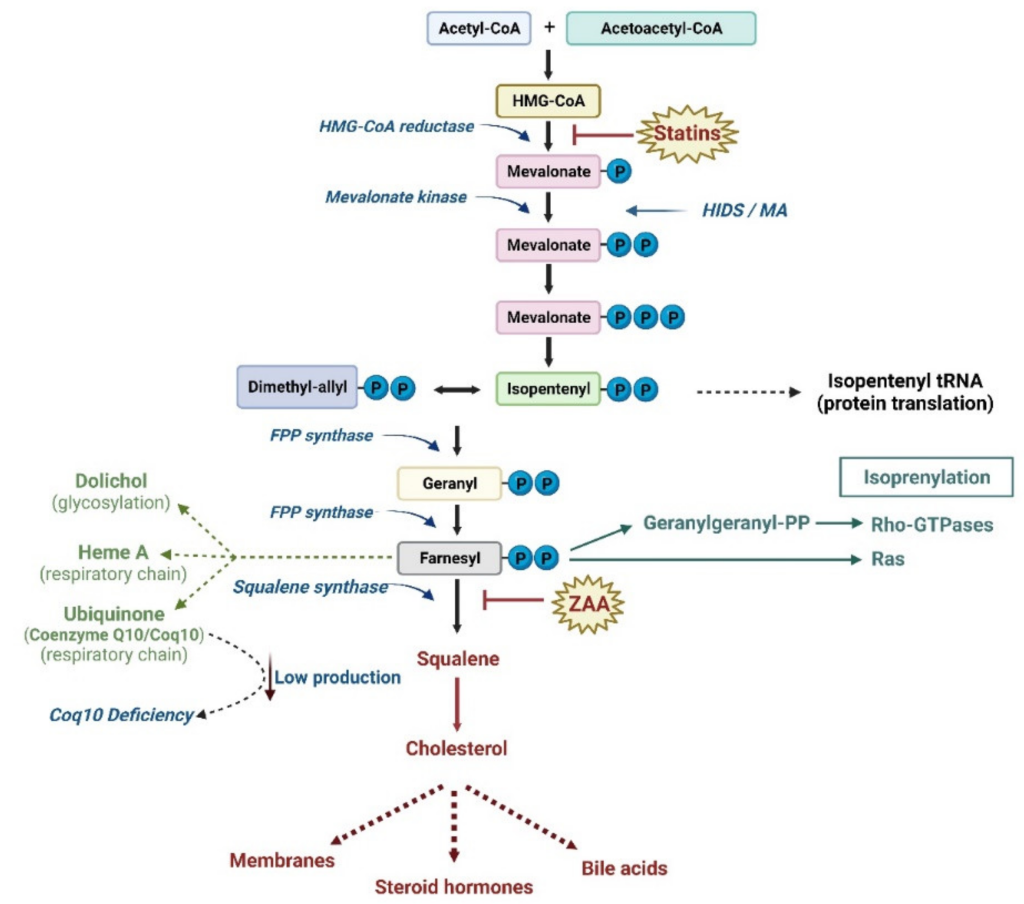
HMG-CoA reductase catalyzes the reduction of HMG-CoA with 2 equivalents of NADPH to generate mevalonic acid. Initial approaches in identifying molecules that engage this enzyme involved large natural product screens in the 1970s. The first molecule that was identified to inhibit the enzyme was compactin, produced by the fungi Penicillium. (Figure 3.6) Compactin has several important chemical features:
- Lactone ring: This ring functions as a pro-drug that opens up to form a carboxylate, whose anionic charge is necessary to engage a critical Lys735 of HMG-CoA Reductase. The hydrolyzed lactone mimics the 3,5-dihydroxylheptaonic acid of the natural enzyme substrate. Importantly, stereochemical configurations of the 3- and 5- position are important to engage with the enzyme.
- Didehydrodecalin ring: This hydrophobic ring engages with a pocket that forms upon the acid binding to the enzyme.
Compactin was ultimately not approved as a drug, due to safety concerns, but similar compounds were advanced, such as lovastatin and simvastatin. These drugs maintain the same lactone-spacer-didehydrodecalin chemotemplate. An interesting compound is pravastatin which does not contain the lactone, but closely resembles the 3R,5R-dihydroxyheptaonic acid moiety of the substrate, mevalonic acid. Note that all of these molecules have relatively short half-lives. Since cholesterol synthesis mainly occurs at night, it is recommended to take these medications in the evening so that they can be most effective in blocking cholesterol synthesis.
Although blocking HMG-CoA reductase inhibits cholesterol synthesis in the liver, the liver still requires cholesterol for proper functioning. The lower concentrations of cholesterol in the liver stimulate the uptake of cholesterol from LDL and VLDL in the blood. Therefore, extracting the LDL/VLDL from the blood will reduce the total cholesterol levels.
Second-generation statins retain some of the key structural properties that were identified above, including the dihydroxyhepatonic acid, which is critical for enzyme binding to Lys735, as well as the stereochemical configuration of the alcohol groups. However, the moiety that engages with the hydrophobic pocket was altered to contain heteroatoms, leading to atorvastatin (Lipitor) and rosuvastatin (Crestor). Both molecules contain p-fluorophenyl and isopropyl substituents which contribute to receptor affinity. The key distinguishing feature between these molecules is that atorvastatin contains an amide where as rosuvastatin contains a sulfonamide, which leads to differential properties. Atorvastatin is the most lipophilic statin and can cross through several cellular membranes including different muscles and tissues. Contrastingly, rosuvastatin is the most hydrophilic statin and the most potent. Both drugs have different CYP metabolic profiles, where atorvastatin is largely metabolized by CYP3A4 and rosuvastatin is metabolized by CYP2C9.
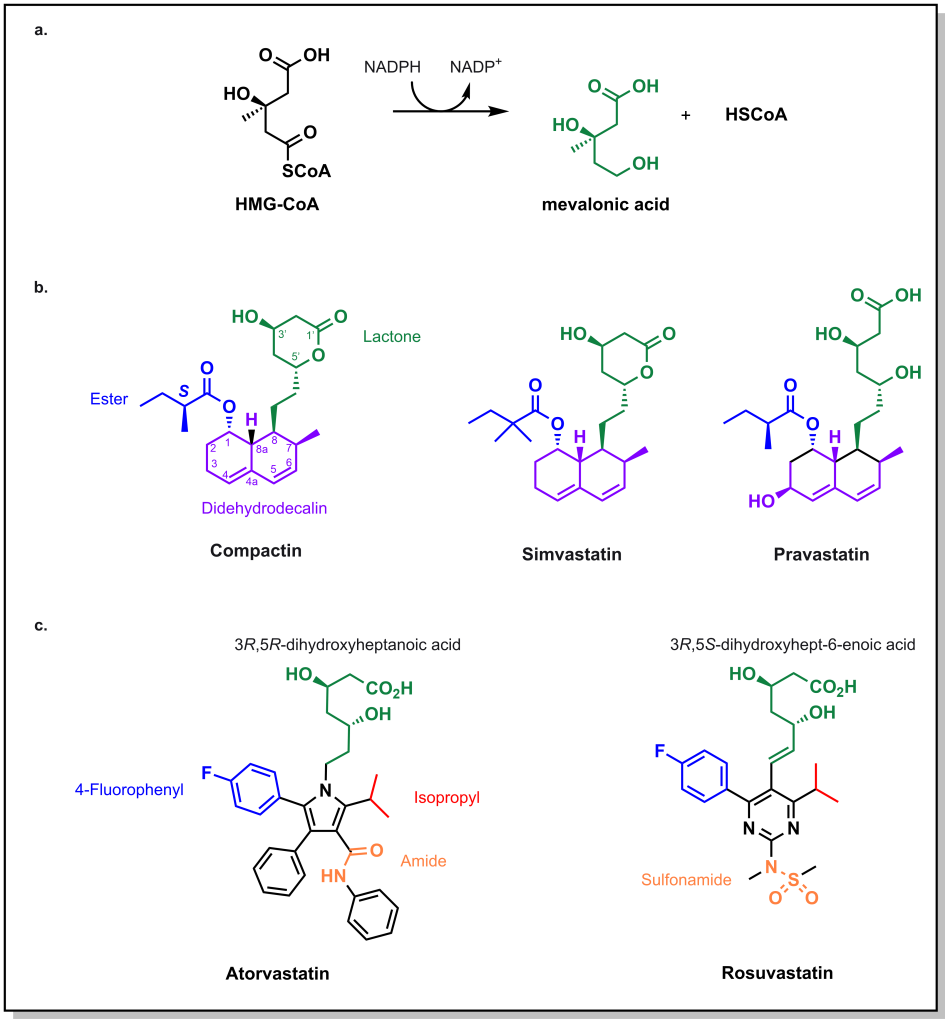
There is also controversy over the types of side effects observed with these statins, with the most common (1-25%) observed as muscle weakness and rhabdomyosis (where the muscle breaks down and releases substances in the blood which can lead to renal or cardiac damage). Although the mechanism is not fully understood, blocking HMG-CoA reductase and reducing mevalonic acid formation reduces the substrates for other biochemical pathways, such as synthesis of Coenzyme Q10 (which is a molecule that is important for muscles and energy usage, Figure 3.5). Although some patients can take supplements, the available data are inconclusive. Additionally, the more lipophilic statins can pass through the CNS which can lead to effects in the brain, including memory loss.
3.2.2 Inhibiting Lipid Synthesis: Niacin
Niacin (or vitamin B3) is a small molecule that has been used to block triglyceride synthesis from adipose tissue. (Fig 3.7) Although it has been in use in the clinics for >50 years, the mechanism of action is still not fully understood.
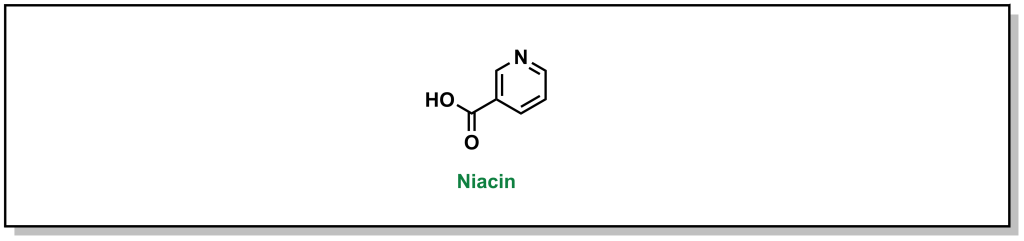
Niacin binding occurs at GPR109A (a GPCR receptor) that ultimately inhibits adenyl cyclase activity (reducing the conversion of ATP to the secondary messenger cAMP). This blocks PKA activation, and subsequent Hormone Sensitive Lipase (HSL) activity. HSL is responsible for degrading triglycerides into free fatty acids that would be released into the blood stream. The reduced concentrations of free fatty acids in the blood, leads to lower VLDL formation and triglyceride synthesis in the liver. (Figure 3.8)
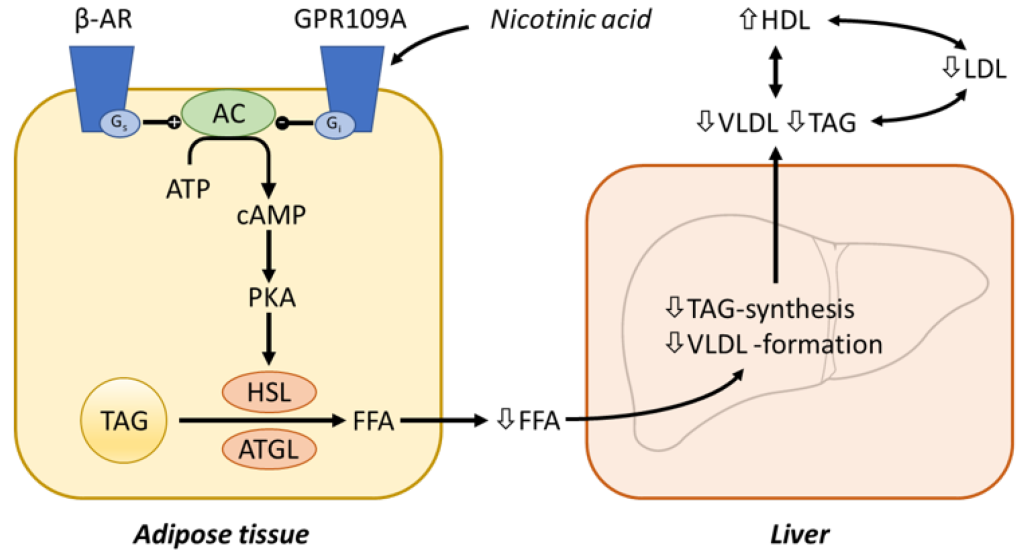
3.2.3 Inhibiting Lipid Uptake: Ezetimibe
Another mechanism for reducing cholesterol levels is to prospectively prevent cholesterol from being absorbed during food intake. Dietary cholesterol is absorbed in the small intestine via the action of a receptors called NPC1L1. (Figure 3.9) Cholesterol will bind these receptors, and the cholesterol-receptor complex will be internalized via the AP2-clathrin mediated processes.
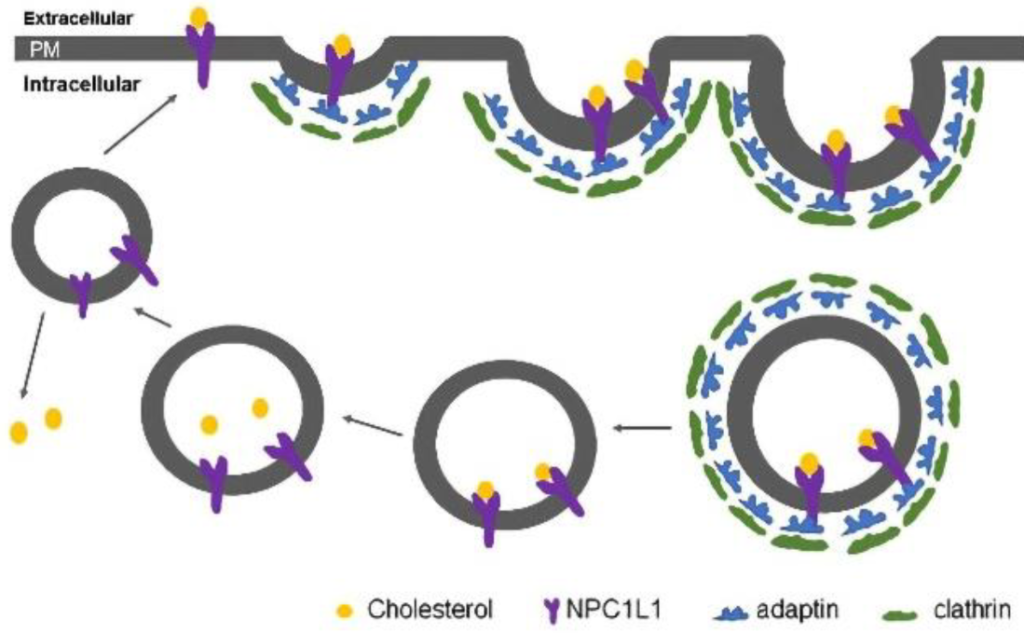
Ezetimibe is a drug that blocks the interaction between the NPC1L1 receptor and the clathrin molecules, which prevents the cell from absorbing cholesterol. The 1,4-diaryl-β-lactam structure is critical for activity. These drugs can be combined with statins to effectively reduce the amount of cholesterol that is absorbed as well as synthesized. (Figure 3.10)
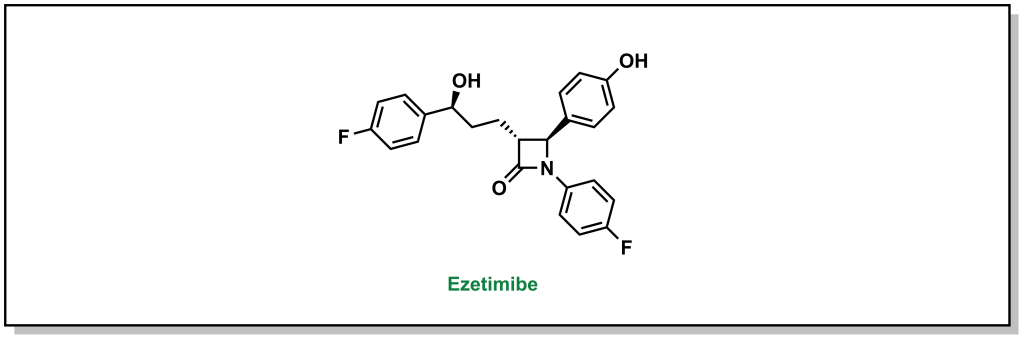
3.2.4 Accelerating Lipid Degradation/Clearance: Fibrates
Fibrates are a class of compounds that can reduce effects of hypertriglyceridemia. Fibrates activate peroxisome proliferator-activated receptor alpha (PPARα) which heterodimerizes with another protein called retinoid X receptor (RXR) and subsequently binds the PPARα response elements to regulate the expression of a cluster of genes involved in lipid metabolism. This increases HDL and reduces TG synthesis. (Figure 3.11)
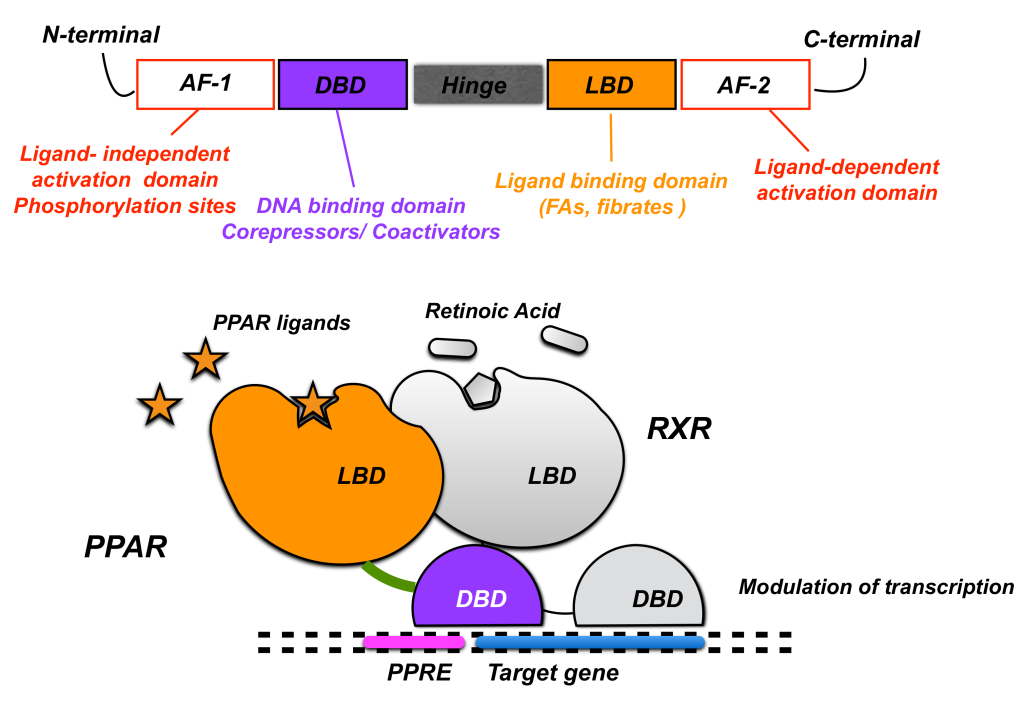
The main pharmacophore for fibrates binding and activating PPARα includes a phenoxyisbutyric acid, which forms an ionic-dipole interaction with a specific tyrosine residue (Tyr464) on PPARα. There are two main fibrates referred to as fenofibrate and gemfibrozil. (Figure 3.12)
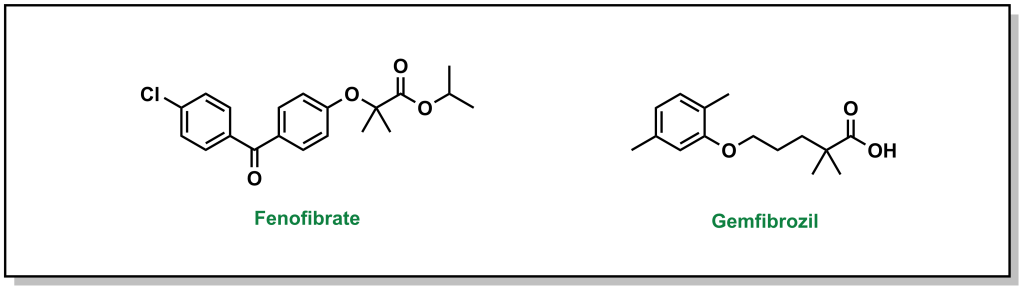
3.2.5 Accelerating Lipid Degradation/Clearance: Bile Acid Sequestrants
As mentioned above, the hydrophobic nature of lipids can create challenges during digestion and absorption, considering the aqueous environment of the stomach and small intestine. Dietary lipids are digested with the help of bile (which is a complex fluid largely consisting of bile acids and cholesterol derivatives). Bile is produced by the liver and stored in the gall bladder. Following digestion, ~97% of the bile is re-absorbed in the ileum and stored in the gall bladder. However, the introduction of bile acid sequestrants will bind to the bile, which prevents it from being re-absorbed and causes it to be excreted. In response to this loss of bile, the liver synthesizes additional bile acids, which requires uptake of cholesterol and LDL from the blood, ultimately lowering the lipid concentration.
Bile acid sequestrants (BAS) are positively charged cations that will bind to negatively charged bile acids, and this interaction creates a complex that is incapable of being absorbed. BAS are normally powders that are taken orally. They are not absorbed by the small intestine, which limits any systemic adverse effects, but they can be challenging to consume and can cause different forms of GI distress.
3.3 Summary
A summary of the different pharmacological properties of lipid lowering agents is shown in Table 3.1.
Table 3.1: Summary of lipid lowering agents.