1 Respiratory system: Anatomy and function
anatomy of the nasal cavity
Air flows through the ventral, middle, and dorsal meatuses (which are separated by the nasal tissue of the ventral and dorsal conchae/turbinates). Air continues past the ethmoid conchae in the caudal nasal cavity, then through the choanae into the nasopharynx.
Particles impact on the mucosa of the nasal turbinates, and mucociliary clearance propels trapped particles to the nasopharynx where they are swallowed.
Olfactory epithelium is present in the caudal nasal cavity, and olfactory nerves penetrate the cribriform plate before entering the olfactory lobe of the brain. Note the close association of ethmoid turbinates and brain, so that nasal neoplasia or fungal infections may induce neurologic disease by local invasion, and viruses may gain access to the brain via the olfactory nerves.
Question:


Airways
The epithelium of the trachea and bronchi consists mainly of pseudostratified ciliated epithelium. Cilia move at about 20 beats per second, propelling the mucus blanket toward the nasopharynx.
Non-ciliated cells (named “club cells”) become more numerous in the smaller airways, and outnumber ciliated cells in the small bronchioles. These are the progenitor cells to replace damaged ciliated epithelium, they metabolize and detoxify chemicals and drugs in some species, and secrete proteins that help to regulate the inflammatory response.
Mucus lining the large airways is produced by goblet cells in the surface epithelium, and mucosal glands in trachea & bronchi. Mucus-secreting cells are absent from the smaller bronchioles. In addition to secreting mucus, these cells produce proteins that defend the airways against infection: lysozyme, defensins, cathelicidins, and others.
The airway smooth muscle induces bronchoconstriction that regulates the airway diameter. Smooth muscle constriction is regulated by the autonomic nervous system and locally secreted mediators. For example, inflammatory mediators can induce bronchoconstriction.
Bronchus-associated lymphoid tissue (BALT) acts in the surveillance of inhaled antigens, as well as the effector responses of antibody production and cell-mediated mucosal immune responses.

Alveoli
Type I pneumocytes (membranous pneumocytes) are the thin cells that cover 95% of the alveolar surface. They are so thin that they are barely seen histologically. Type I pneumocytes are thin to allow gas exchange and pump fluid out of the alveolus. Because the cytoplasm contains so few organelles, these cells have few defences against injury.
Type II pneumocytes are cuboidal cells at the corners of the alveolus. They are the progenitor cells: following loss of type I pneumocytes, the type II pneumocytes proliferate to form a cuboidal epithelium lining the alveolus, and eventually differentiate to type I pneumocytes. Type II pneumocytes secrete surfactant lipids and proteins. Surfactant is composed of lipids—mainly phosphatidylcholine—which are stabilized by surfactant proteins B & C. Surfactant reduces surface tension to allow dilation of the alveolus with each breath. Finally, type II pneumocytes biotransform xenobiotics (including toxins) in some species. Similar to the situation with periacinar hepatocytes, this biotransformation function can lead to toxic lung damage.


Alveolar macrophages
In the healthy lung, most alveolar macrophages are a self-perpetuating population of cells residing in the lung. These resident alveolar macrophages are “housekeepers” that ingest and remove inhaled particulates, recycle or degrade surfactant, and produce inflammatory mediators that generate a rapid response to infectious agents. But, infection in the lung causes neutrophils and monocytes to be recruited from the blood, and these monocyte-derived macrophages are more pro-inflammatory and capable of causing lung damage.
Pulmonary function
The major function of the lung is gas exchange: O2 absorption and CO2 excretion. This is dependent on all of the following:
- Ventilation. Adequate ventilation requires adequate functioning of the respiratory centre in brain, respiratory muscles, a normal balance between bronchodilation and bronchoconstriction, and patency of airways.
- Gas exchange. Gases are exchanged across a barrier formed by type I pneumocytes, alveolar interstitium consisting of a fusion of the 2 basement membranes, and endothelium. This barrier can be thickened by exudate in the alveolus, by “hyaline membranes” (cell and protein debris, discussed later), by thick cuboidal type II pneumocytes that have replaced damaged membranous type I pneumocytes, or by thickening of the alveolar septum with fibrous tissue.
- Perfusion. Low oxygen tension in the alveoli triggers local pulmonary vasoconstriction, which serves to match blood flow to ventilation within individual acini. By reducing blood flow to non-ventilated areas of lung, it prevents non-oxygenated blood from reaching the systemic circulation. However, widespread hypoxia—for example, due to high altitude or bronchiolar obstruction—may induce widespread vasoconstriction leading to increased resistance to blood flow through the lung, and consequent right heart failure.
Respiratory physiological trivia to thrill and amaze you …
- Gas exchange in the alveolus takes 0.25 seconds—1/3 of the transit time of the erythrocyte. As a result, conditions that thicken the alveolar septum (fibrosis, cellular hypertrophy) can easily reduce the exchange of oxygen between the alveolar air and the hemoglobin-laden erythrocytes.
- The entire blood volume—about 5 litres in humans—passes through the lung each minute. In animals with pulmonary intravascular macrophages, the lung is effective in removing foreign material such as bacteria, and is a prime target organ of endotoxemia. However, conditions that impair blood flow through the lung will result in right heart failure, a condition known as cor pulmonale.
- The total surface area of the human alveoli is about 80 m2 — the size of a tennis court. Imagine having to keep a tennis court sterile! Without water!
- Human lung contains about 20,000 acini and 480,000,000 alveoli. Each mm3 of human lung contains 170 alveoli.
- A person inspires about 10,000 litres of air daily. An effective system of defence is essential to protect the lung from the particles that accompany this inhaled air.
Endocrine functions of the lung include angiotensin metabolism and prostaglandin production.
Biotransformation of some toxins occurs in the lung. Club cells and type II pneumocytes, like hepatocytes in the liver, convert some chemicals and drugs to reactive intermediates, which are then detoxified to compounds that can be more easily excreted. However, if these reactive intermediates cannot be adequately detoxified, they may react with membrane lipids and injure resident cells of the lung.
Pulmonary mechanics and gas exchange
Pulmonary compliance measures how easily the lung expands. It is defined by the change in lung volume resulting from a given change in transpleural pressure (ΔV/ΔP). For non-mathematical thinkers: with normal compliance, chest expansion induces a big change in volume during inspiration and expiration; with reduced compliance, the same effort gives a lower tidal volume.
Airway resistance is the difficulty with which air flows through the airways. It is increased by bronchoconstriction, exudates in the airway lumen, or edema and inflammation of airway wall. Normally, most airway resistance is in the larger airways & nasopharynx, so partial obstruction of the upper airways has a big effect on lung function.
Causes of hypoxemia
- Hypoventilation: due to respiratory depression, weak respiratory muscles, chest injury, upper airway obstruction, airway diseases. These changes reduce lung compliance and/or increase airway resistance. If the lungs are not ventilated, gas exchange cannot occur.
- Impaired diffusion: due to diseases affecting the alveolar septum. These especially induce hypoxemia, because O2 diffusion takes 0.3 sec, while capillary transit time for blood is only 0.75 sec. CO2 diffuses more readily than O2; therefore, barriers to diffusion of gas across the alveolar wall tend to cause hypoxemia but not hypercapnea, unless they also alter the matching of ventilation and perfusion.
- Ventilation-perfusion mismatching: In poorly ventilated areas of lung, blood supply is diminished because of alveolar hypoxic vasoconstriction. However, inflammation can cause dilation of these blood vessels, so that non-oxygenated blood flows past the non-ventilated alveoli to reach the systemic circulation and thus causes hypoxemia.
Shunts: congenital anomalies that may cause right-to-left shunting. As above, this can cause severe hypoxemia, because non-oxygenated blood reaches the systemic circulation.
Key points
The conducting system of the respiratory system includes the nasal cavity, trachea, bronchi, and bronchioles. It is lined by ciliated epithelium and covered by a layer of mucus, and functions to warm and humidify the inspired air, remove inhaled particles, and protect the lung against infection.
The alveoli are mainly lined by type I pneumocytes, which have very little cytoplasm. The thinness of the type I pneumocytes and the lack of interstitial connective tissue in the alveolar septa are essential for normal gas exchange and maintaining pulmonary compliance, but make the lung susceptible to injury.
Type II pneumocytes are cuboidal epithelial cells lining alveoli, which are the regenerative cells that replaces injured type I pneumocytes, and also metabolize toxins and produce surfactant.
Alveolar macrophages are present in normal lung, and function to remove inhaled particles including bacteria.
Pulmonary Defences
The necessary function of gas exchange requires that the lung remains exposed to the environment. Thus, the sterility of the lung is continuously challenged by micro-organisms and foreign material in inhaled air, and by opportunistic pathogens within droplets that are aspirated from the non-sterile upper respiratory tract. Despite this constant challenge, the lung must remain sterile, or at least minimally contaminated, because excessive numbers of bacteria induce inflammation, and inflammation impedes gas exchange in the lung. Thus, the lung requires a multi-layered system of defence against infectious agents, including mucociliary clearance, antibodies and innate defence proteins, alveolar macrophages, and neutrophils.
If the infection cannot be contained by mucociliary clearance and defence proteins, inflammation is triggered in an attempt to control the threat: alveolar macrophages produce inflammatory mediators that recruit neutrophils and more macrophages to the site of invasion, and increase the production of antibacterial proteins by airway epithelial cells. But this response has the potential to do harm: inflammatory exudates impair gas exchange, leukocyte-derived enzymes and oxygen radicals cause injury to lung tissue, and repair processes organize alveolar exudates into fibrous tissue that permanently decreases lung compliance and thickens the blood-gas barrier.
Deposition and clearance of inhaled particulates
Important particulates include infectious agents, allergens, dusts, and inert particles (e.g. asbestos, silica). The nasal cavity removes most particles >10 mm in diameter. Most 3-10 mm diameter particles lodge in the trachea and bronchi, and are removed by the mucociliary apparatus. 0.1-5 mm particles, such as bacteria, may reach terminal airways.
Airway mucus is secreted by goblet cells and by bronchial mucosal glands. The secretions of these glands cover the epithelial surface; they form a lower aqueous layer in which the cilia beat, and a superficial mucus blanket that traps particles and carries them upward. Mucus contains many protective substances:
- Defensins, cathelicidins, lysozyme, complement, and lactoperoxidase cause direct damage to microbes.
- Immunoglobulin A blocks attachment of bacteria to mucosal surfaces.
- Immunoglobulin G, complement C3b, and surfactant proteins A & D opsonize bacteria and make them tastier for macrophages.
- Lactoferrin binds iron and makes it unavailable for pathogens.
Alveolar macrophages are derived from blood monocytes, or from macrophages that replicate in the lung. Particulates that reach the alveoli are engulfed within 2 hours by alveolar macrophages, and this is enhanced if particles are opsonized by antibody, complement, or surfactant proteins. Macrophages kill bacteria using oxygen radicals, nitric oxide, and lysosomal enzymes. They also call more neutrophils and macrophages to the site of infection.
Pulmonary intravascular macrophages reside within the capillaries of the alveolar septa. Pulmonary intravascular macrophages are present in pigs, horses, ruminants, and cats, but not in healthy rodents, dogs, or humans. These cells remove infectious agents and other particles from blood, similar to stellate macrophages (Kupffer cells) in liver and macrophages in splenic sinusoids. In species that have pulmonary intravascular macrophages, sepsis tends to target the lung, causing pulmonary edema and dyspnea.
How are respiratory defences overcome?
- Aspiration pneumonia: exposure to an overwhleming number of bacteria.
- Viruses predispose to bacterial pneumonia by damaging the airway epithelium and reducing mucociliary clearance by reducing the secretion of antimicrobial peptides and by impairing the function of alveolar macrophages.
- Mycoplasma and Bordetella adhere to cilia and impair mucociliary clearance.
- Stresses and adverse climatic conditions are important causes of reduced lung defence. Stress reduces alveolar macrophage function and pulmonary immune responses, and inhibits production of antimicrobial peptides. Cold-stressed animals have reduced mucociliary function.
- Immunosuppressive drugs reduce pulmonary immune responses.
- Neutropenia deprives the lung of a major defence mechanism: neutrophils.
- Pollutants and toxic gases, for example in poorly ventilated or overcrowded barns, may reduce the ciliary function in airway epithelial cells.
- Genetic disorders are a rare cause of impaired lung defences. Ciliary dyskinesis: affected cilia lack the normal dynein arms, so ciliary beating is abnormal. Cystic fibrosis in children: altered ion exchange results in viscous airway mucus which cannot be adequately propelled to the pharynx.
Common artefacts
The following photos illustrate some normal features that can be confused with lesions, and some common artefacts.
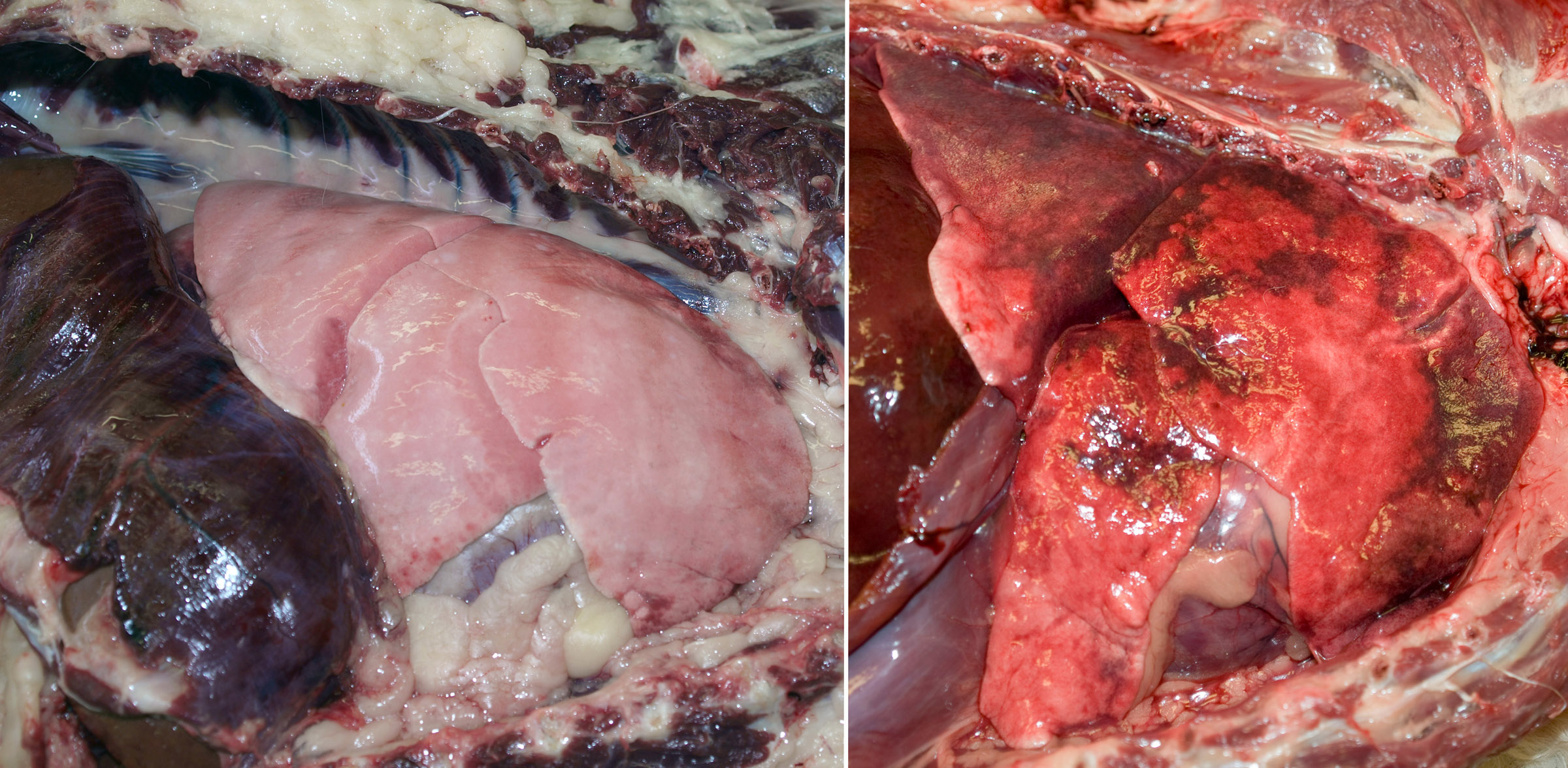



Answer:
Normal nasal anatomy in a cow (left) and Yorkshire terrier dog (right). Identify the nasal meatuses, dorsal, middle, & ventral concha (turbinates), ethmoid conchae, nasopharynx, and the thin cribriform plate separating the nasal cavity from the olfactory lobe of the brain.