3.5 – Reactions of Carboxylic Acids and Derivatives
Carboxylic Acids and its Derivatives
A carboxylic acid is a functional group containing a central carbon atom bound to both a carbonyl group and a hydroxyl group. Various examples of carboxylic acids are displayed below in Figure 3.5.a. The carbonyl carbon is electron-deficient due to the surrounding electronegative oxygen atoms, rendering this carbon electrophilic.
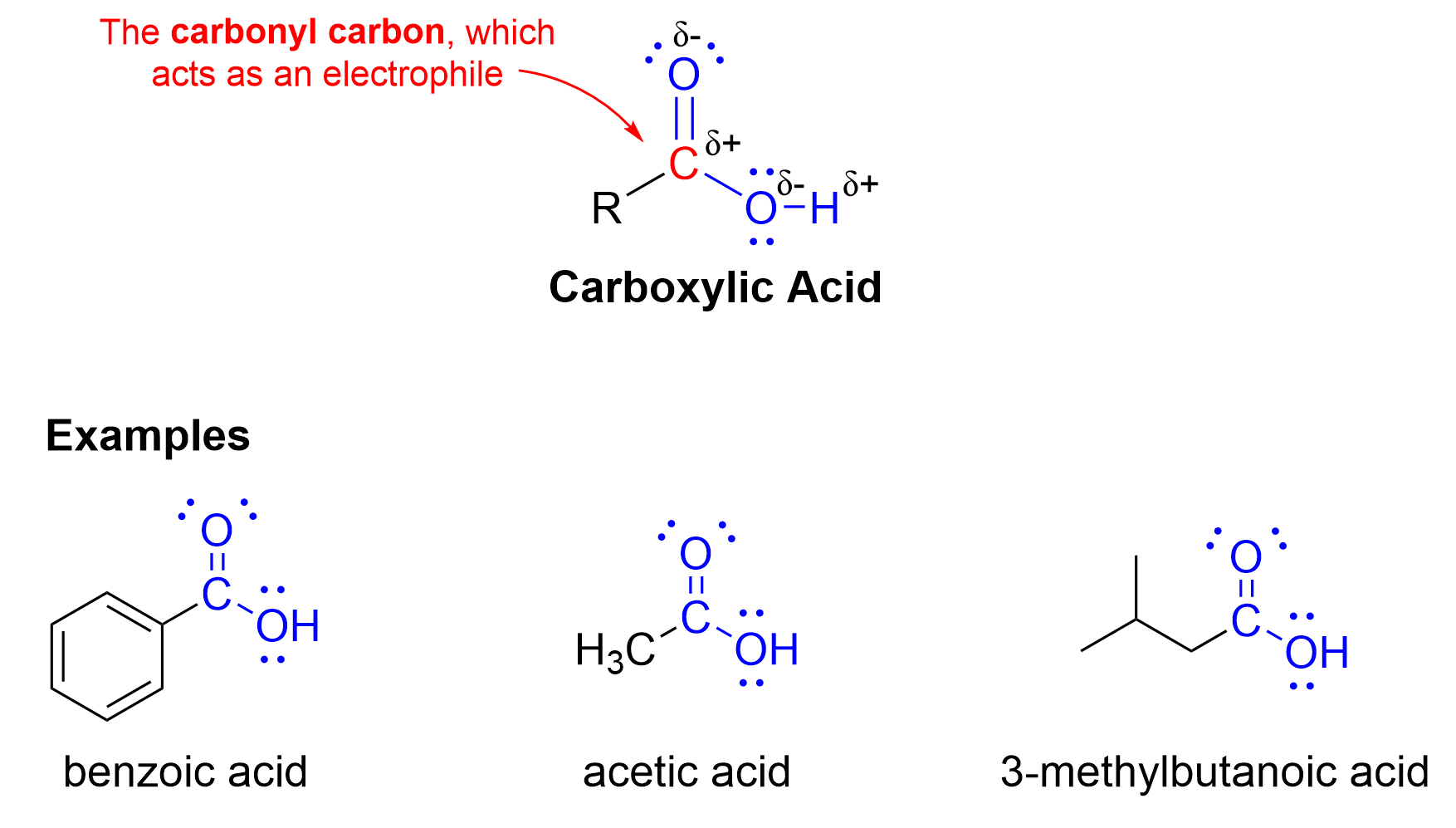
There are also several carboxylic acid derivatives (Figure 3.5.b), as described in Chapter 2.3. These derivatives all contain a central carbon that contains a carbonyl group along with a single bond to an electronegative atom or group. In these derivatives, the carbon center contains three total bonds to heteroatoms that are more electronegative than carbon. As a result, carboxylic acid derivatives share a similar oxidation state around the central carbon. Many carboxylic acid derivatives have similar reactivity patterns, with the carbonyl carbon acting as an electrophile, similar to the reactivity to aldehydes and ketones.
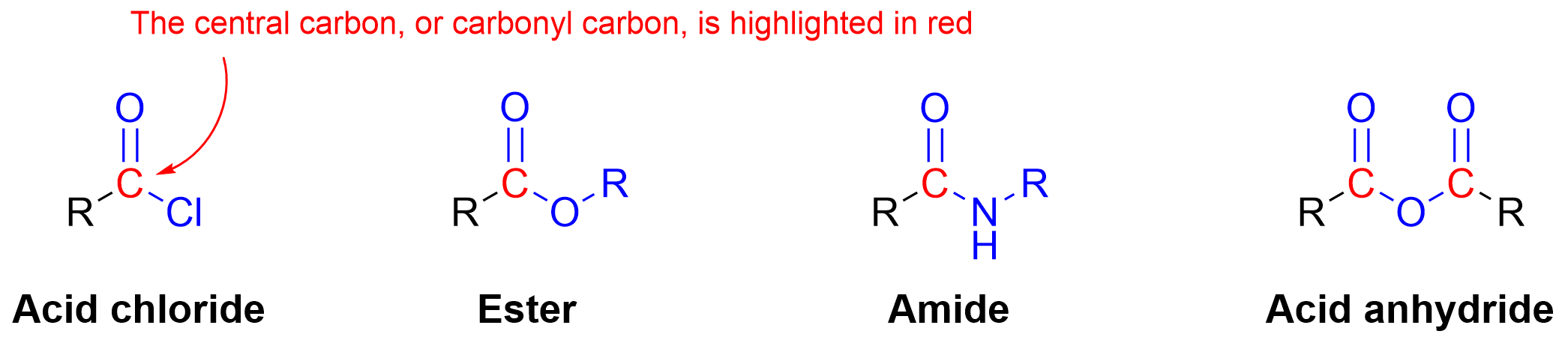
Carboxylic acids are weakly acidic functional groups, with Ka values in the 10-5 range. Thus, carboxylic acids react with water in an equilibrium reaction to generate a small proportion of the conjugate base, known as a carboxylate, along with hydronium ions, H3O+ (Figure 3.5.c, top). Carboxylic acids also react with strong bases, such as sodium hydroxide, and the reaction goes to completion to generate the conjugate base carboxylate and water (Figure 3.5.c, bottom).
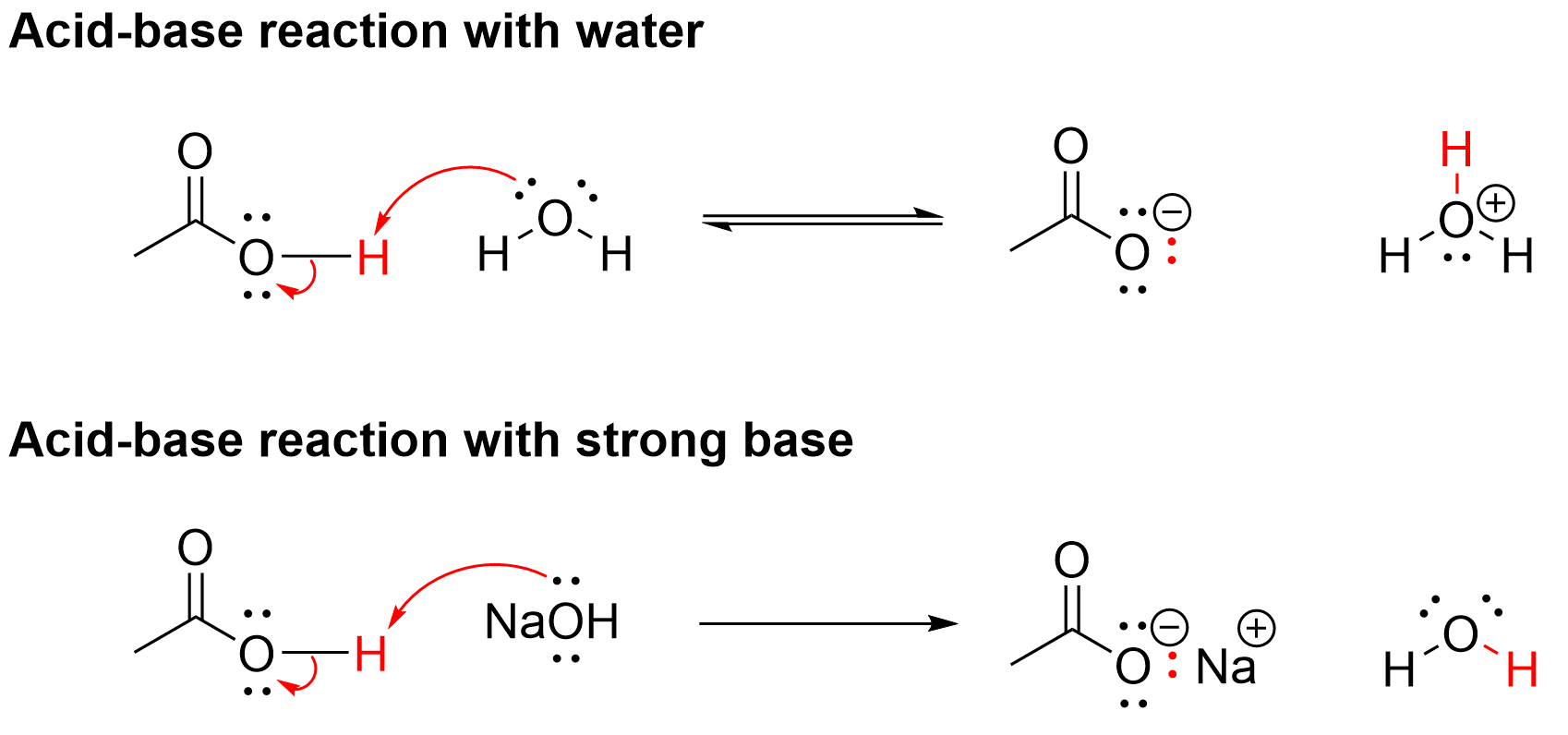
The Synthesis of Carboxylic Acids
Based on what has been covered in previous chapters, there are two major methods to synthesize carboxylic acids.
The first method involves the oxidation of a primary alcohol or an aldehyde to a carboxylic acid (Figure 3.5.d), which was discussed in Chapter 3.3. When reacting a primary alcohol with an oxidizing agent such as KMnO4 or K2Cr2O7, two successive oxidations will occur. The primary alcohol will first be oxidized to an aldehyde, and then immediately oxidized further to a carboxylic acid. An aldehyde can also be reacted with these oxidizing agents to form a carboxylic acid in a single step.
![A primary alcohol undergoes oxidation, represented as [O] above the reaction arrow, to form an aldehyde. The aldehyde goes through a second oxidation and forms a carboxylic acid. In this example, butanol turns into butanal and then butanoic acid.](https://ecampusontario.pressbooks.pub/app/uploads/sites/3169/2023/06/3.5.d.-Oxidation-to-c-acid.png)
Another method to synthesize carboxylic acids involves reacting a Grignard reagent with carbon dioxide, CO2 (Figure 3.5.e). The Grignard reagent acts as a nucleophilic carbon center as discussed in Chapter 3.4.2, while the CO2 acts as an electrophile due to its electron-deficient carbon center. In the first step of the reaction, the nucleophilic Grignard reagent attacks the electrophilic carbon of the carbon dioxide, resulting in the formation of a new C–C bond. At the same time, one of the C=O π bonds breaks, with both electrons moving to oxygen. The result is a carboxylate anion. In the second step, the addition of acid protonates the carboxylate, producing a neutral carboxylic acid.
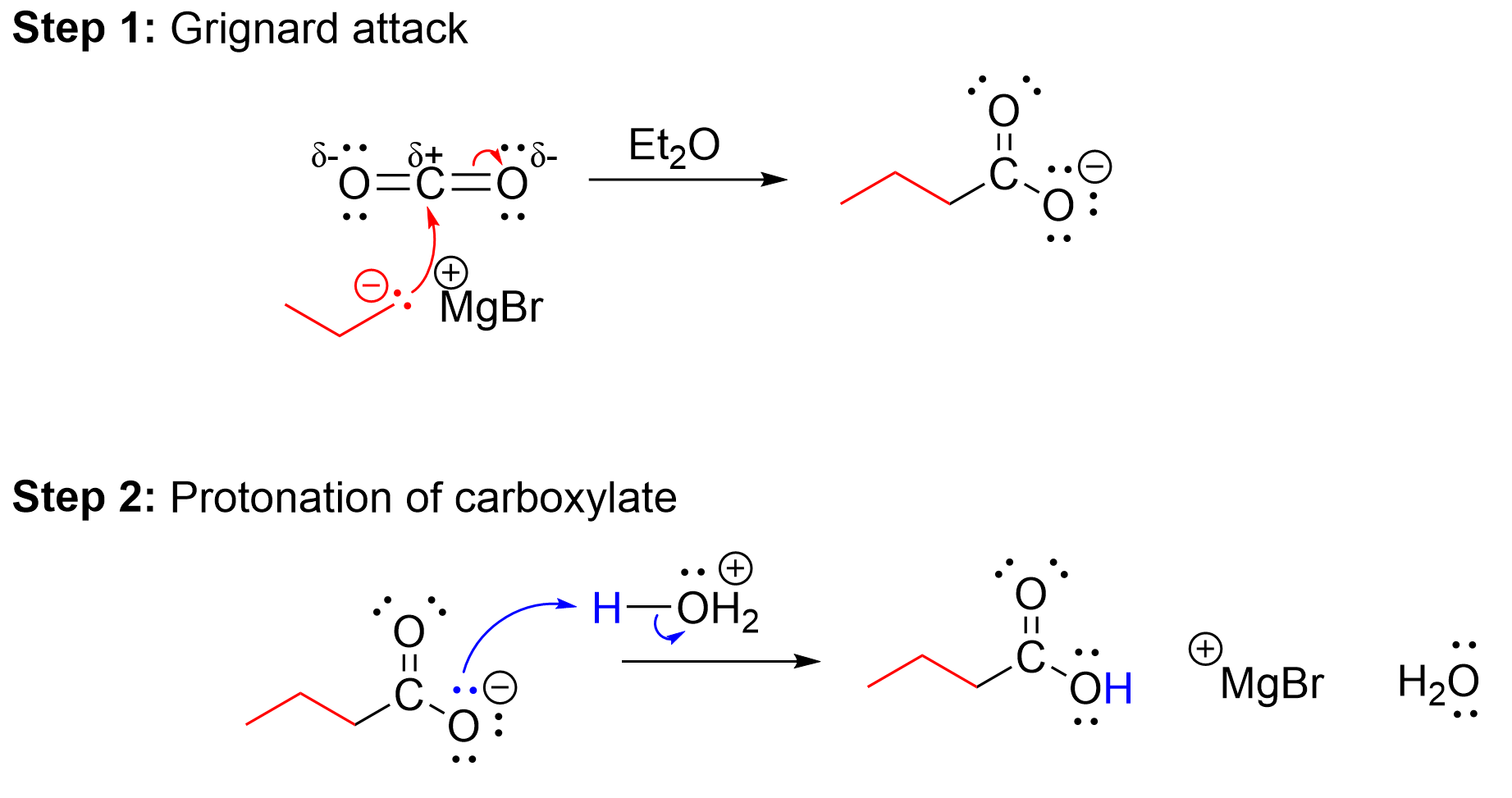
(The full solution to this problem can be found in Chapter 5.2)
The Synthesis of Esters
An ester can be synthesized through an SN2 reaction (Figure 3.5.f), as discussed in Chapter 3.1.2. A carboxylate acts as a nucleophile due to the lone pairs on the negatively charged oxygen, while an alkyl halide acts as an electrophile.
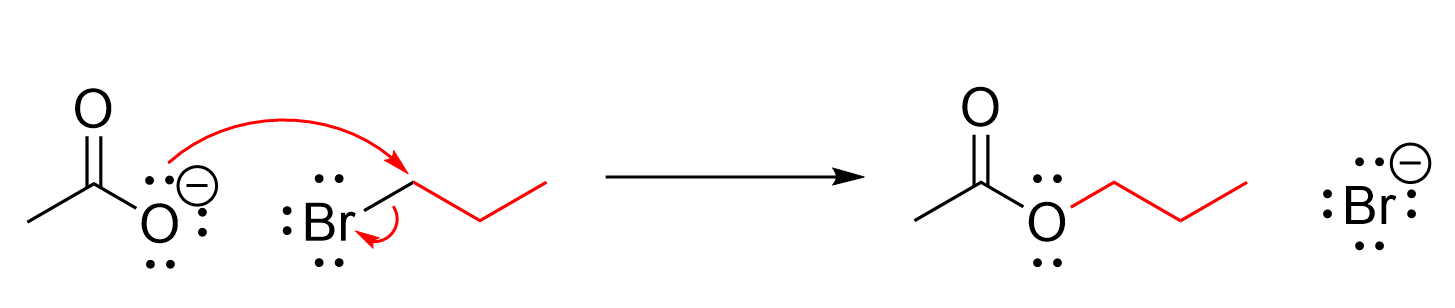
The carboxylate uses a lone pair of electrons on oxygen to attack the electrophilic carbon. With the introduction of a new bond, the bromine atom leaves simultaneously, taking the pair of electrons from the covalent bond with it to form a bromide anion. Recall that as an SN2 reaction, these events happen via a concerted mechanism in one step, and primary and methyl alkyl halides work best due to the reduced steric bulk. This reaction introduces a new carbon chain to the oxygen, forming an ester.
Esters can also be formed through an acid catalyzed reversible reaction of carboxylic acids and alcohols. This reaction is an equilibrium, and appreciable amounts of all reactants and products are found at equilibrium. The forward reaction to form an ester is called esterification, while the reverse reaction to break apart the ester is called ester hydrolysis.
In the acid catalyzed esterification reaction, a carboxylic acid and alcohol react to produce an ester and water under heated conditions with an acid catalyst. The reverse reaction is known as ester hydrolysis. Hydrolysis involves using water (hydro) to break a molecule apart (lysis), in this case an ester. The products of ester hydrolysis are a carboxylic acid and an alcohol.

These reactions are equilibrium processes, and thus, we can take advantage of Le Chatelier’s principle to push the reaction one way or the other. For example, to drive the forward reaction, a high concentration of alcohol is used, and the amount of water used is minimized. Conversely, to drive the reverse reaction, a greater amount of water is used. An acid catalyst and heat are required for both the forward and reverse reactions.
(The full solution to this problem can be found in Chapter 5.2)
Key Takeaways
- A carboxylic acid is a functional group containing a central carbon atom bound to both a carbonyl group and a hydroxyl group – where carbonyl carbon is electrophilic.
- There are several carboxylic acid derivatives containing a central carbonyl group with a single bond to an electronegative atom/group.
- Carboxylic acids are weakly acidic and form the conjugate base carboxylate in water.
- Carboxylic acids are synthesized by either oxidation of a primary alcohol or aldehyde, or through a Grignard reaction with carbon dioxide.
- An ester can be synthesized through an acid catalyzed reversible reaction of carboxylic acids and alcohols (esterification).
- Subsequently, the reverse reaction of esterification is ester hydrolysis, both reactions are in equilibrium and follow Le Chatelier’s principle.
Diversity in Chemistry: Agnes Pockels
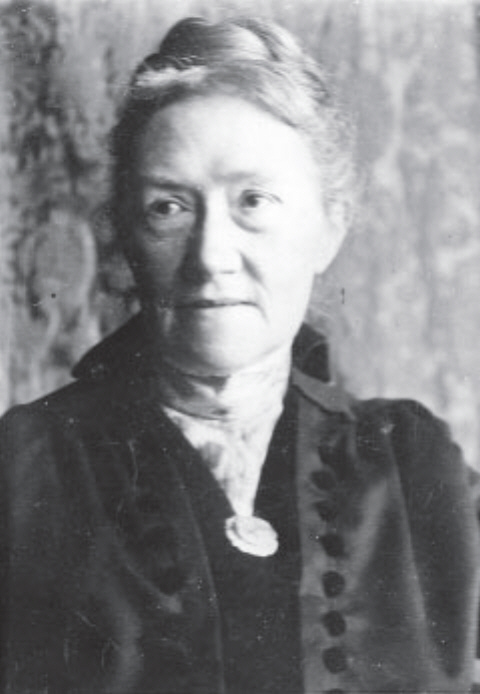
A common use of carboxylic acids in our everyday life is for soaps, which are made from fatty acid carboxylate salts. Although most of us would probably not bat an eye at how these soaps interact with liquids, Agnes Pockels found an interest in surface tension after observing soapy water while doing the dishes at home. As a young child, Pockels developed an interest in science, but as women in Germany were not allowed to attend university, she self-studied at home using her brother’s textbooks. At 18 years old though, inspired by her observations while washing dishes, Pockels started conducting experiments in her own home by constructing a slide trough to measure surface properties of soapy water. Her invention, later on, played a role in developing the widely used Langmuir-Blodgett Trough used in surface chemistry today. With her brother’s connections, who was a developing scientist at the time, she was able to obtain a publication in 1891 in the well-renowned Nature journal with no formal scientific training. Although she never received a formal appointment for her research, she was acknowledged by commentators after Langmuir won the Nobel Prize in Chemistry in 1932, and is considered a pioneer of surface chemistry.
Study Notes – Chapter 3.5
Any feedback or comments on this chapter? You may either email chemoer@mcmaster.ca, access this MS Form, or provide a comment in the feedback box below.