4.3 – Aromaticity
Introduction to Aromaticity
One notable feature about homovanillyl alcohol (HVA) is that it is an aromatic compound. HVA contains a benzene ring: a six-membered ring structure consisting of three alternating single and double bonds (Figure 4.3.a).
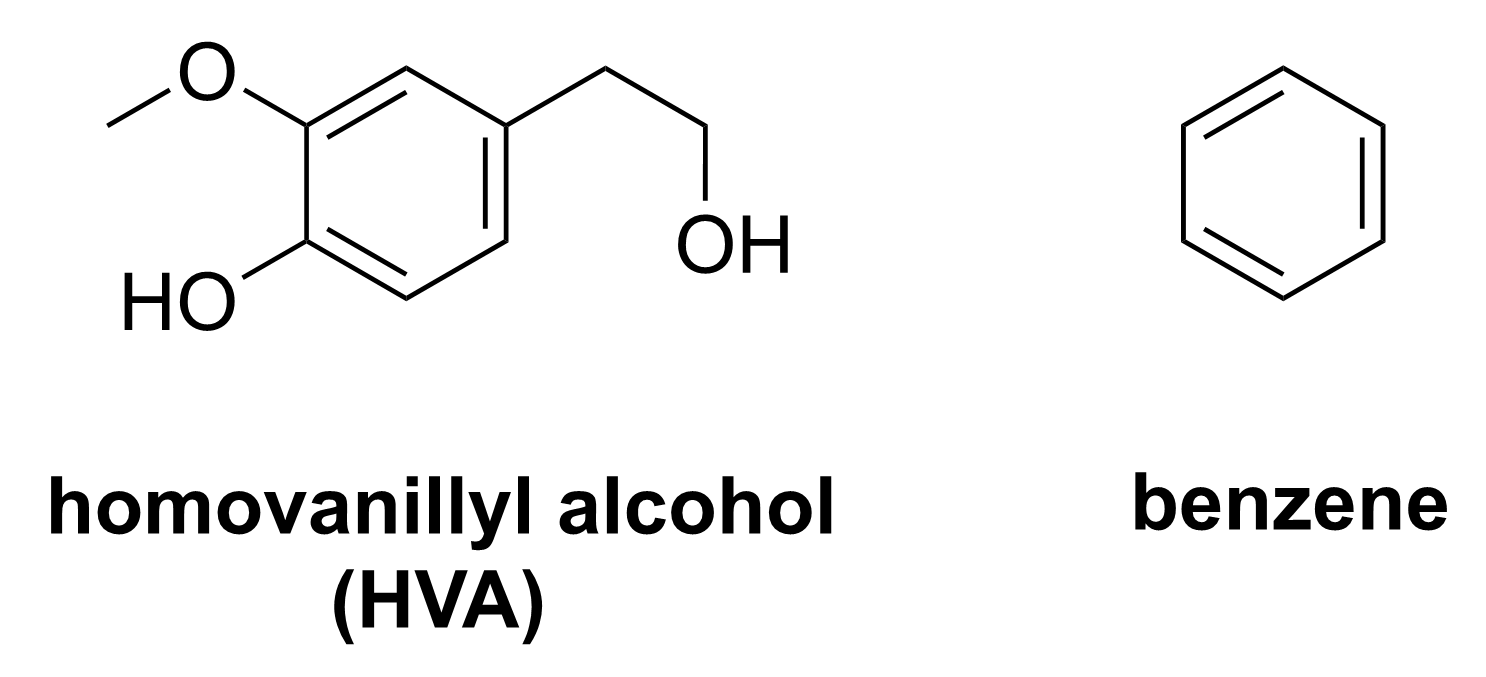
Aromatic structures exhibit different reactivity than alkenes, despite having a similar electronic arrangement. Figure 4.3.b showcases the reaction of bromine (Br2) with cyclohexane, cyclohexa-1,3-diene and benzene. While cyclohexane and cyclohexa-1,3-diene react readily with bromine in a halogenation reaction at room temperature, benzene does not react with bromine under these same conditions. Aromatic structures are found to be significantly more stable than regular alkene structures as they are resistant to addition, halogenation, oxidation and reduction reactions to which alkenes are typically susceptible.
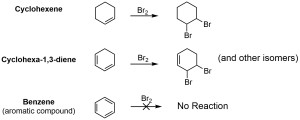
An experimental observation of benzene is that all six of the carbon-carbon bonds are all the same length, 1.39 Angstrom (Å). This length is intermediate between the typical C–C single bond (1.53 Å) and the typical C=C double bond (1.32 Å), suggesting that the bonds have equal bond order and equal energy. In benzene, each carbon-carbon bond has a bond order of 1.5.
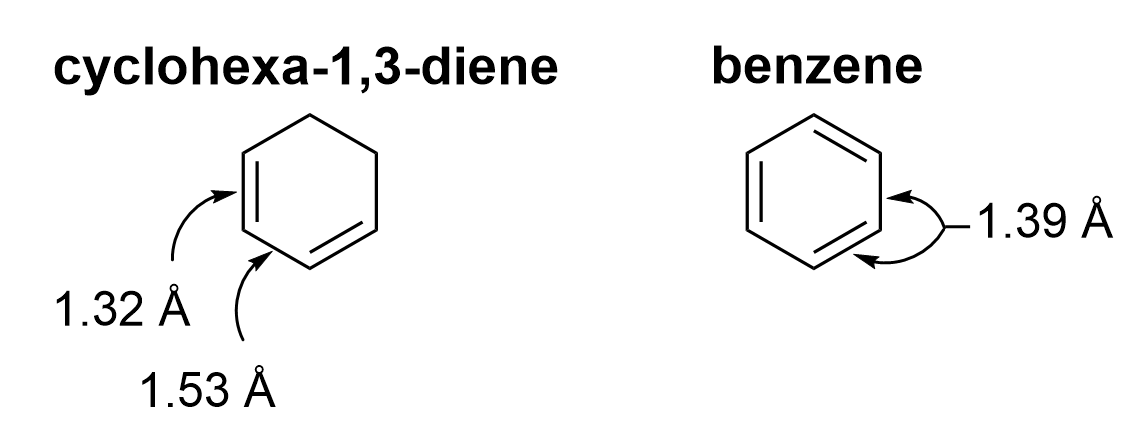
Electron Delocalization in Aromatic Compounds
The defining characteristic of aromatic compounds, and the source of their stability, is the delocalization of electrons within the cyclic structure. In a benzene ring, each carbon is trigonal planar and sp2 hybridized, with one p orbital on each carbon available to overlap and form π bonds. All carbon atoms in benzene occupy the same plane, and all p orbitals occupy the space above and below that plane. Each carbon atom contributes one p orbital and one electron, to form a single aromatic π orbital system with 6 electrons. This aromatic π orbital system occupies the space directly above and below the plane of the ring. The six electrons are considered delocalized as they form one large electron cloud and are free to move anywhere throughout the system (Figure 4.3.c and 4.3.d).
The delocalization of electrons is supported by the observation of a carbon-carbon bond order of 1.5 in benzene. This is also observed with many other ring systems with π electron density, suggesting that aromaticity is a property that is not restricted to benzene alone. Thus, benzene behaves as though all six p orbitals overlap together, rather than as three isolated π bonds.
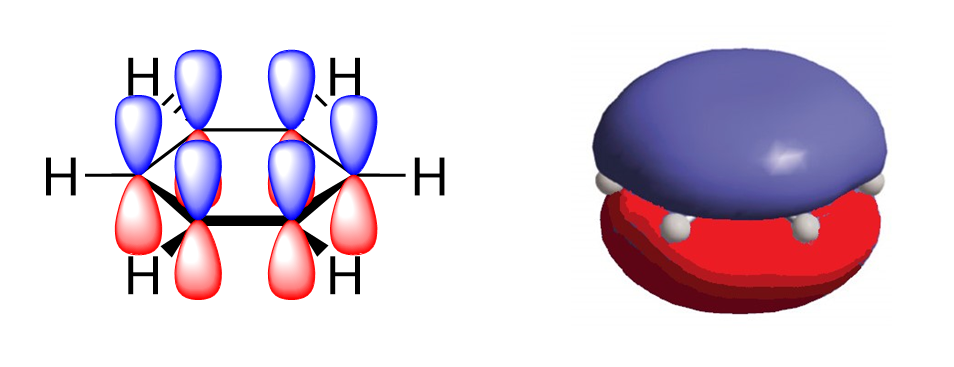
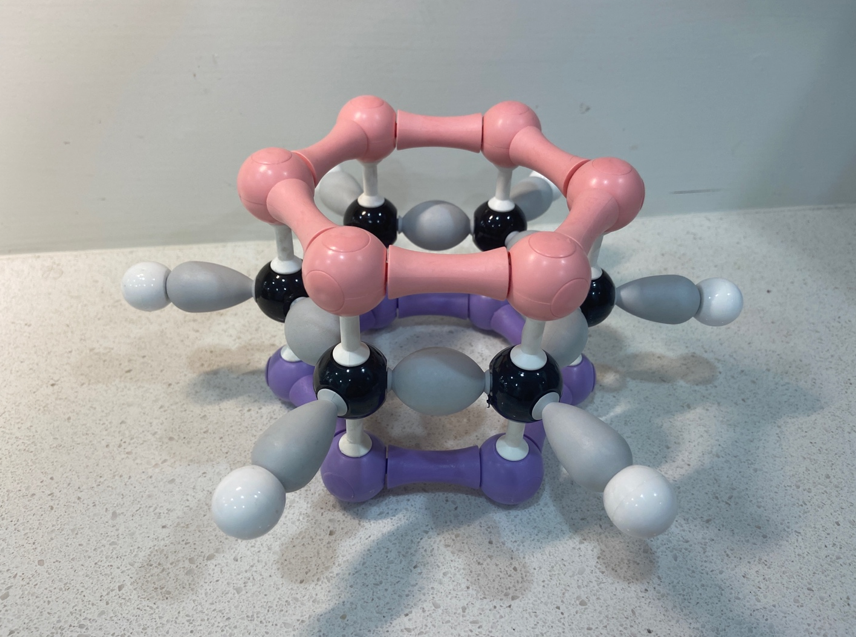
Curved arrows show the movement of electrons through the aromatic compound, demonstrating the electron delocalization (Figure 4.3.d). This results in 2 equivalent line bond representations of benzene, which are referred to as resonance contributors. A double-sided arrow is used to distinguish one resonance contributor from another, as both structures represent the same molecule.
Because the electrons are delocalized throughout the aromatic π orbital system, neither of the individual resonance contributors showing alternating single and double bonds are an accurate representation of the molecule. In reality, the molecule exists as a resonance hybrid, which is a weighted average of all resonance contributors.
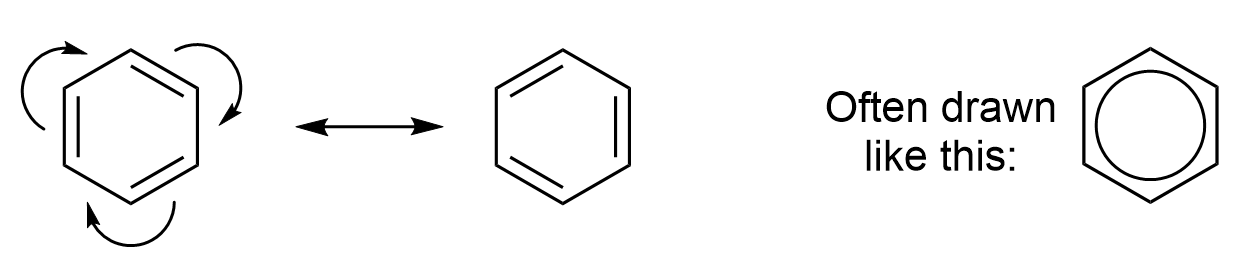
There are numerous kinds of arrows used in organic chemistry. Here is a quick summary of the different arrows you will see. Ensure that you are always using the correct arrow!
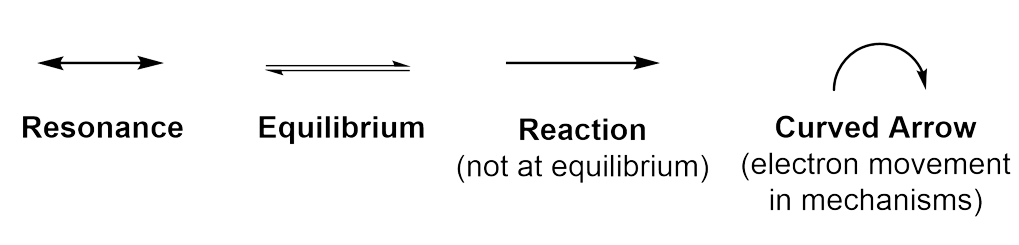
Criteria for Recognizing Aromaticity
Aromaticity is a characteristic that extends beyond benzene rings. Many ring systems that contain π bonds are observed to be resilient to reactions that alkenes are generally susceptible to. Aromatic systems can contain any number of atoms in their rings, and they can contain heteroatoms and/or formal charges within their cyclic system.
The following three criteria are used to predict whether a structure is aromatic:
1. The structure is cyclic
Aromaticity requires a ring structure in the molecule. The cyclic structure can either be the entire molecular skeleton or one (or more) cyclic region(s) within a larger molecule. If there is no ring present, then the compound is not aromatic.
2. All atoms are sp2– or sp-hybridized
Aromaticity requires continuously overlapping p orbitals. As a result, all atoms must be sp2– or sp-hybridized, in order to contribute a p orbital to the π orbital system. If any atoms in the ring are sp3-hybridized, then the compound is not aromatic.
3. The number of π electrons obeys Hückel’s rule
Hückel’s rule states that aromatic compounds must have a specific number of electrons in the aromatic π orbital system. We call this the ‘number of π electrons’. An aromatic compound must have 4n+2 number of π electrons, where n is any whole number (0, 1, 2, 3, …). Note that the value of n is not important: n does not correspond with any property of the molecule. What is important is that the number of π electrons is 2, 6, 10, 14, and so forth. If there are an odd number of π electrons, or 4n π electrons (e.g., 4, 8, 12, etc.), then the compound is not aromatic.
For example, benzene contains six π electrons, one in each p orbital at each carbon atom (Figure 4.3.g). Thus, benzene follows Hückel’s rule and is aromatic. When counting electrons, ensure that only π electrons in the cyclic system are being counted in Hückel’s rule. For example, benzaldehyde (Figure 4.3g) is an aromatic molecule with six π electrons in the ring. Although benzaldehyde also contains a π bond in the carbonyl group, those electrons are not counted towards Hückel’s rule. Naphthalene (Figure 4.3g) is another example of an aromatic compound containing 10 π electrons (Figure 4.3.g). As a bicyclic structure, it is made of 10 sp2-hybridized carbons, with 10 π electrons, one in each p orbital, which follows Hückel’s rule.
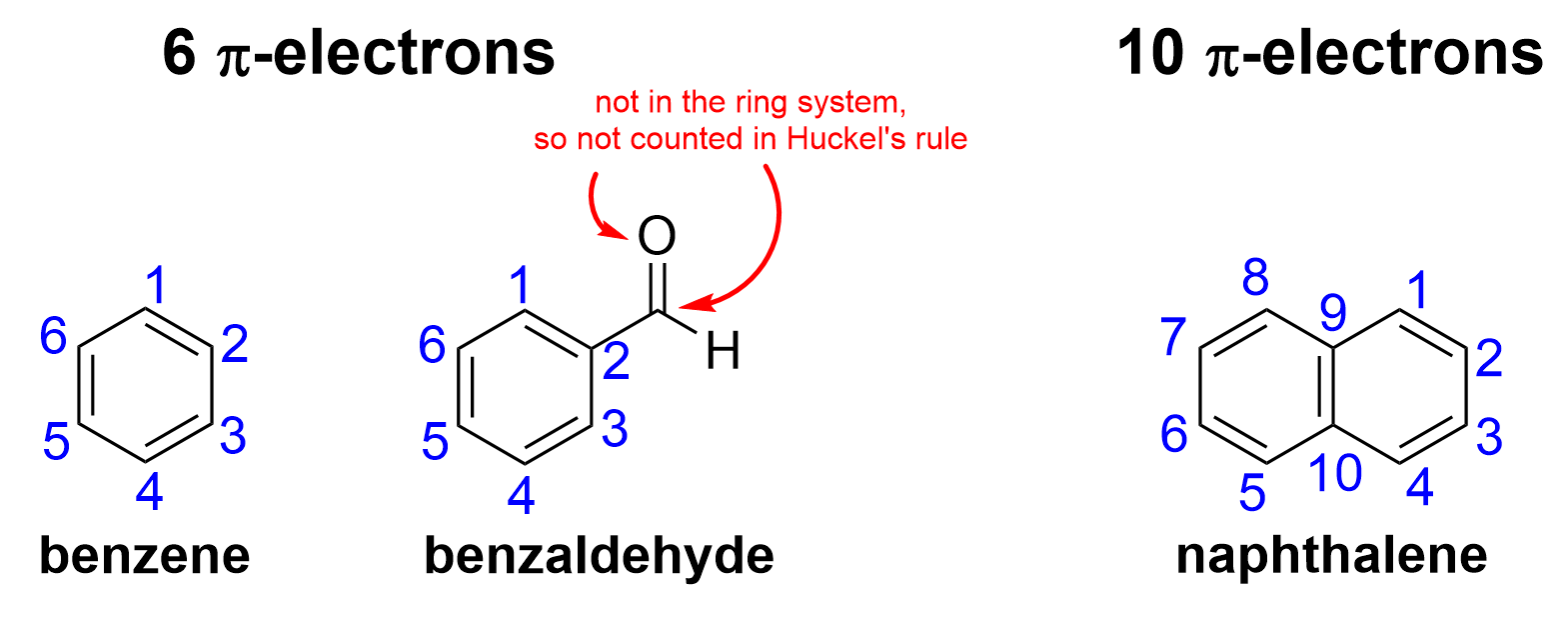
Are You Wondering? Sp-Hybridized Atoms in Aromatic Structures
Earlier, it was mentioned that the atoms in an aromatic structure must be either sp2– or sp-hybridized to contribute at least one p orbital to the pi orbital system. Despite so, most aromatic structures are observed to only have sp2-hybrdized atoms. In fact, sp-hybridized atoms are rarely seen in aromatic structures as they require the presence of an alkyne in a cyclic structure, which are highly unstable. Alkynes prefer to be in a linear geometry of 180o to maximize repulsion of the orbitals, and thus, are highly strained in a ring system and are prone to degrading or reacting.
They may exist as brief intermediates, however, such as benzyne. As the name suggests, a benzyne is similar in structure to a benzene, with the replacement of one double bond for a triple bond (as seen below). It has never been isolated and is only inferred to exist based on the products of certain reactions. Using Hückel’s rules, we can classify a benzyne as an aromatic structure. It is cyclic and comprised entirely of sp2– or sp-hybridized atoms. Lastly, it contains 6 π electrons.
You may ask yourself if the molecule actually contains 8 π electrons due to the presence of the second set of p orbitals on the alkyne. However, as these p orbitals are in a different plane perpendicular to the aromatic system, it is not counted when counting π electrons for Hückel’s rules.
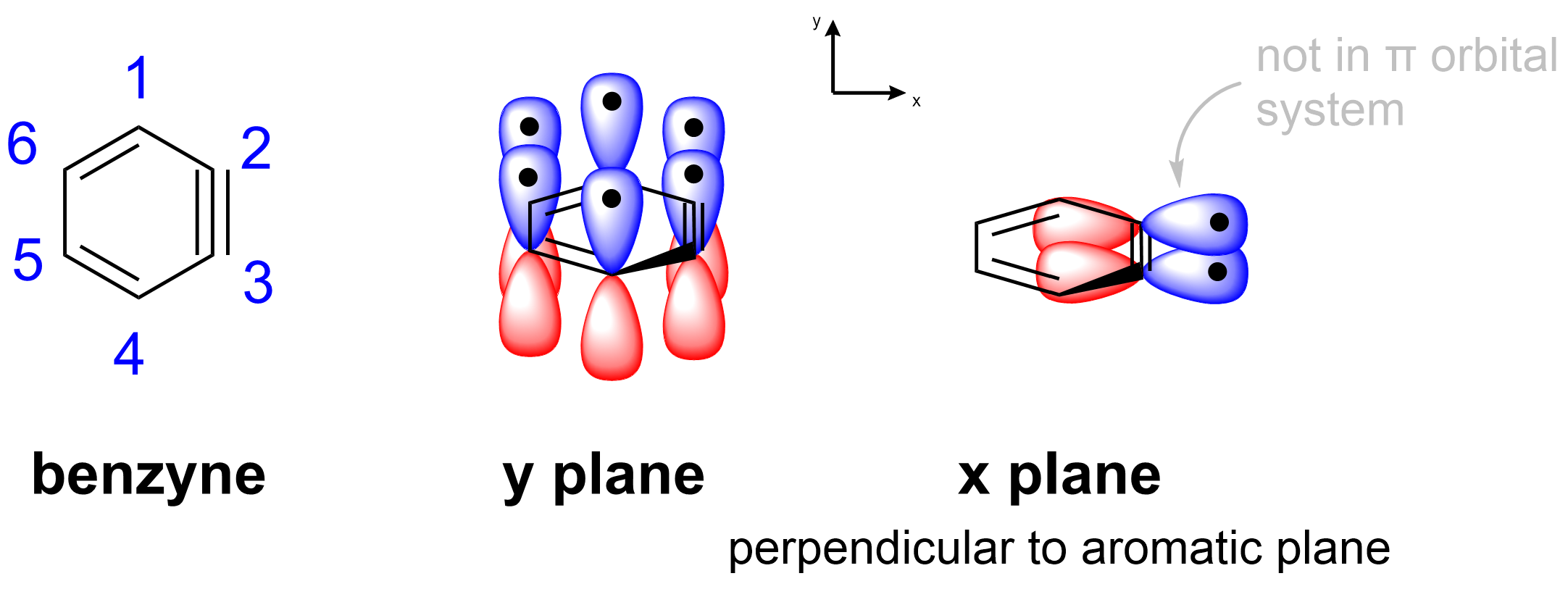
Identifying Aromatic Ions
Aromatic structures can also be cationic or anionic and satisfy all the criteria for aromaticity explained above.
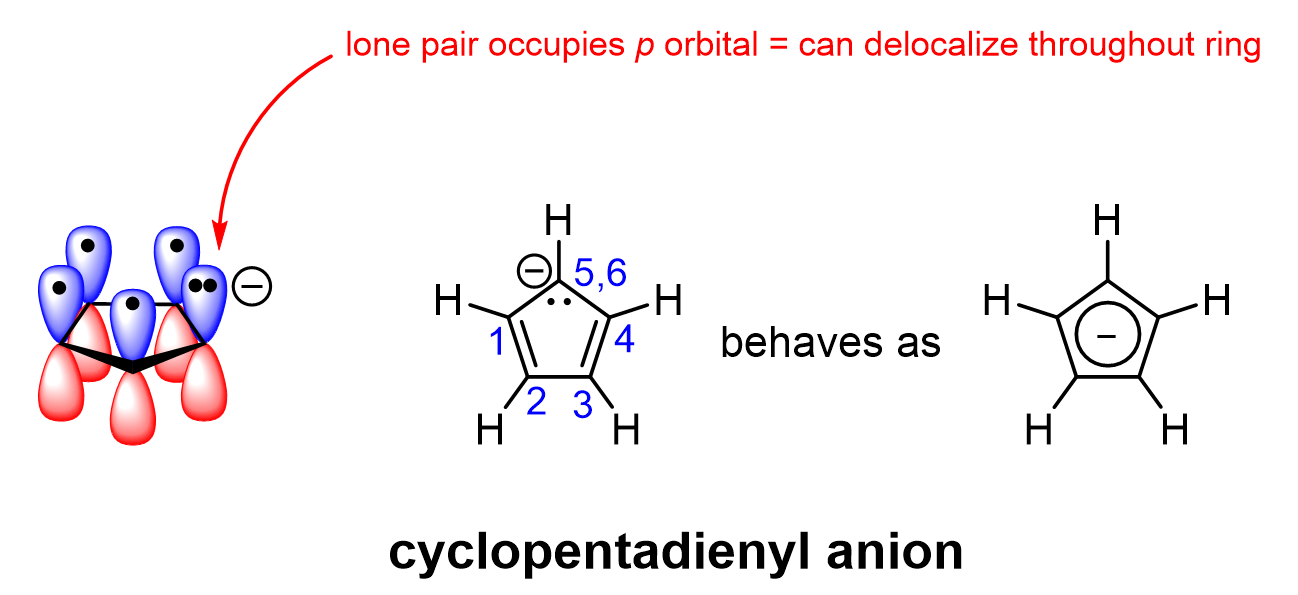
For example, consider the five-carbon anionic aromatic ring, cyclopentadienyl anion. This molecule contains an anionic carbon atom with a lone pair (Figure 4.3.h). This anionic carbon atom, although it is not part of a π bond, is experimentally observed to have trigonal planar geometry, making it sp2-hybridized. The lone pair of electrons must therefore occupy the unhybridized p orbital. This p orbital overlaps with all the other p orbitals in the ring to form the aromatic π orbital system. This allows all π electrons, including the lone pair, to delocalize throughout the ring. Therefore, all five carbon atoms in cyclopentadienyl anion are sp2-hybridized, with overlapping p orbitals, and a total of six π electrons to satisfy Hückel’s rule. Moreover, each carbon in the cyclopentadienyl anion has been experimentally observed to bear some of the negative charge due to the electron delocalization.
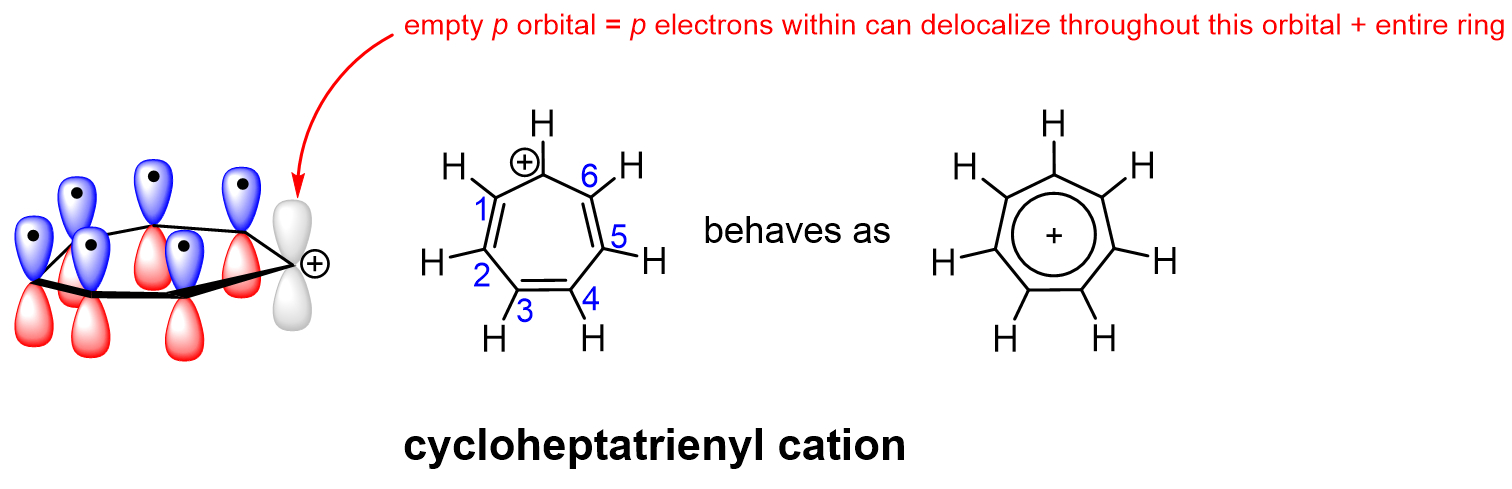
In contrast, the seven-membered cationic aromatic ring, cycloheptatrienyl cation, has a cationic carbon atom (Figure 4.3.i). The cationic carbon is sp2-hybridized, with an empty p orbital. The empty p orbital overlaps with all the other p orbitals in the ring to form the aromatic π orbital system. This allows all π electrons to delocalize throughout the ring. Although the cationic carbon does not have an electron in its p orbital to contribute to the electron cloud, its empty p orbital still allows it to participate in aromaticity. Therefore, all seven carbon atoms in cycloheptatrienyl cation are sp2-hybridized, with overlapping p orbitals, and a total of six π electrons to satisfy Hückel’s rule. Moreover, each carbon in the cycloheptatrienyl cation has been experimentally observed to bear some of the positive charge due to the electron delocalization.
Identifying Heteroaromatics
Heteroaromatics, also called heterocyclic aromatic compounds, are aromatic compounds that contain heteroatoms in the aromatic ring. A heteroatom is an atom other than carbon, such as boron, nitrogen, oxygen, sulfur, etc. Heteroatoms also contribute a p orbital and a certain number of electrons to the π orbital system. For example, a heteroatom can contribute one electron (from a π bond), zero electrons (from an empty p orbital), or two electrons (from a filled p orbital with a lone pair). Below are examples of a heteroatom contributing one electron or two electrons to the π orbital system.
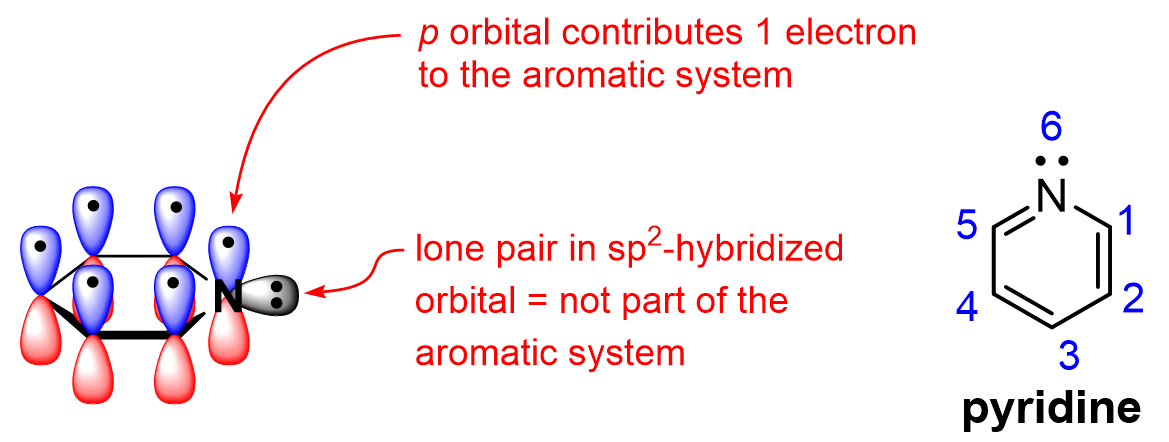
A common heteroaromatic molecule is pyridine (Figure 4.3.j), a six-membered ring containing one nitrogen atom. Experimental observations show that the nitrogen atom has a trigonal planar geometry, making it sp2 hybridized, with an unhybridized p orbital. Since the nitrogen atom is already using its p orbital to contribute to the aromatic π orbital system, the lone pair must occupy the sp2 hybridized orbital. The sp2 hybrid orbital is perpendicular to the π orbital system, and is therefore not part of the π orbital system. The electrons in the sp2 hybrid orbital are not included when counting electrons for Hückel’s rule. Therefore, there are six π electrons in the π orbital system.
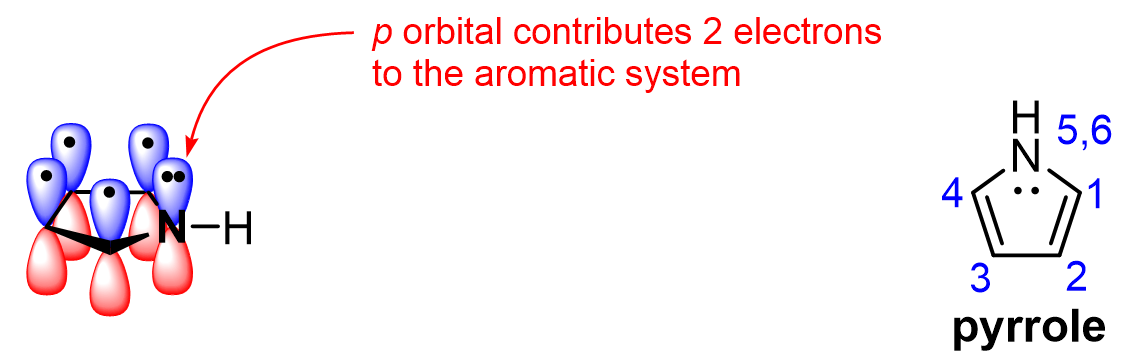
Another common heteroaromatic is pyrrole (Figure 4.3.k), a five-membered ring containing one nitrogen atom. Like pyridine, experimental observations show that the nitrogen atom has trigonal planar geometry, making it sp2 hybridized, with an unhybridized p orbital. Unlike pyridine, the nitrogen is not part of any double bond, and contains only single bonds to all its neighbouring atoms. Thus, the lone pair of electrons must occupy the unhybridized p orbital.
As mentioned above for cyclopentadienyl anion (Figure 4.3.h), the electrons that form the π system of an aromatic ring do not have to come from a π bond. Instead, nitrogen can use its lone pair to contribute two π electrons to the aromatic system to satisfy Hückel’s rule with six π electrons. In fact, pyrrole is isoelectronic with cyclopentadienyl anion.
In this example, you might have expected nitrogen to be tetrahedral and sp3 hybridized to maximize the distance from other neighbouring electron pairs. However, the sp2 hybridized nature of the nitrogen atom allows the electron pair to delocalize across the ring, which is a stabilizing force, making it thermodynamically favorable.
(The full solution to this problem can be found in Chapter 5.3)
(The full solution to this problem can be found in Chapter 5.3)
(The full solution to this problem can be found in Chapter 5.3)
Aromatic Molecules in Biology: DNA
Aromatic structures are heavily abundant in biological systems due to their stabilizing characteristics. For example, the nitrogenous bases in deoxyribonucleic acid (DNA), which form the building blocks of our genetic material, are made up of heteroaromatics.
For example, thymine and cytosine (Figure 4.3.l, top) have six-membered heterocyclic rings in which all atoms are sp2 hybridized, with an unhybridized p orbital that can overlap to form the aromatic π system. Like the resonance contributors shown for benzene (Figure 4.3.d, above), thymine and cytosine also have various resonance contributors, which can be drawn to better indicate the sp2 hybridization of the ring atoms.
Adenine and guanine (Figure 4.3.l, bottom) have two fused rings with 9 atoms. All atoms in the rings are sp2 hybridized. Nine unhybridized p orbitals overlap to form the aromatic π system, containing ten π electrons, which obeys Hückel’s rule. For adenine and guanine, note that the singly bonded nitrogen atom in the five-membered ring is sp2 hybridized, like pyrrole (Figure 4.3.k). For guanine, a resonance contributor can be drawn to better indicate the sp2 hybridization of the ring atoms.
Because of the sp2 hybridization and the aromatic π system, these molecules are planar and highly stable. Planarity allows the nitrogenous bases to easily stack and align in the DNA double helix, while stability limits DNA degradation.
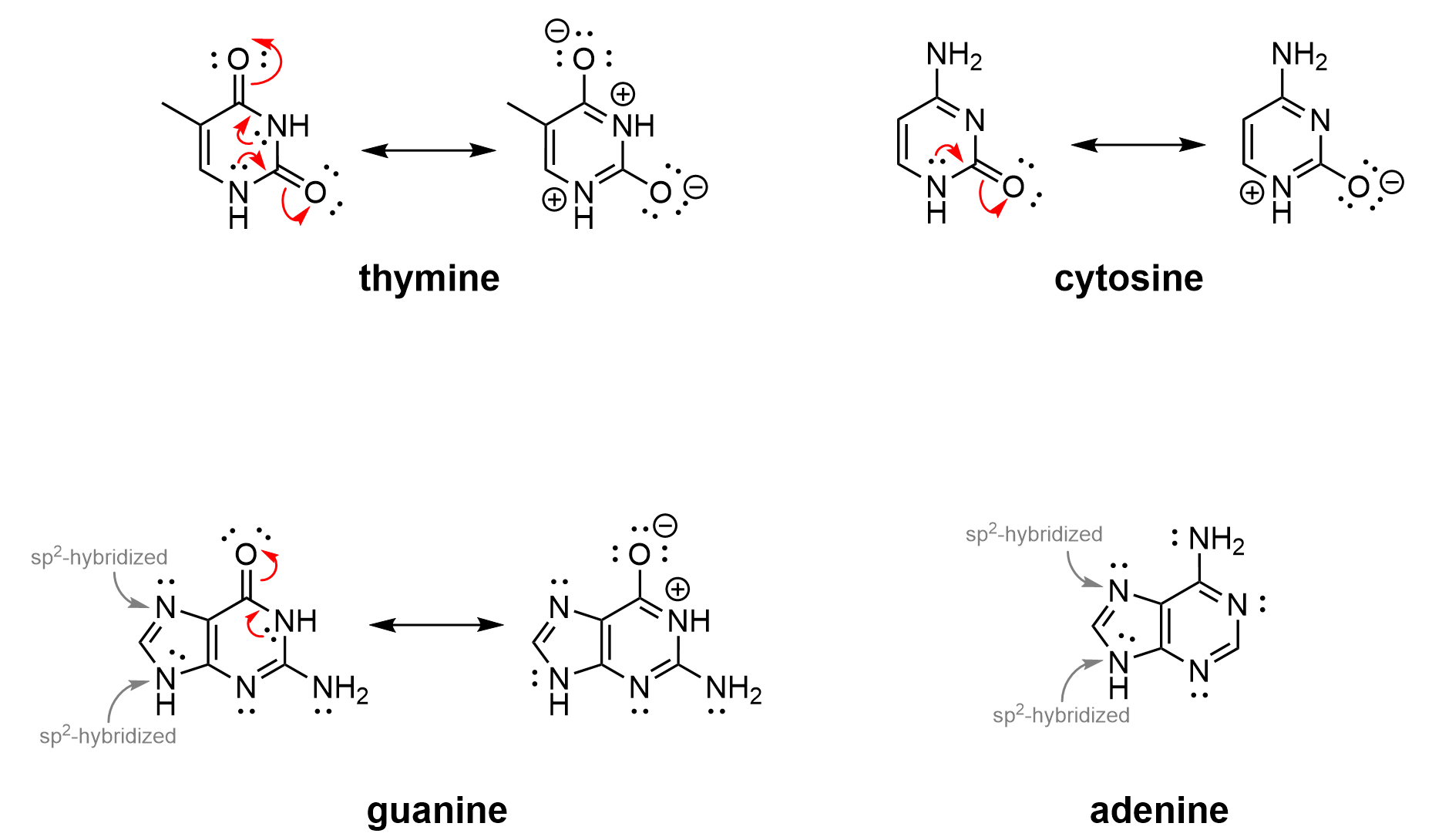
Are You Wondering: Resonance of Aromatic Molecules
As you may recall, in CHEM 1A03 there was content concerning how resonance structures contribute to the stability of molecules. The movement of electrons in resonance structures is a bit beyond the scope of CHEM 1AA3, but below is an explanation of how it works for those who are curious.
Recall that resonance structures occur as movements of electron pairs which form new bonds, then breaking a neighbouring bond in the process. Carbonate is a great example of this, where a lone pair of oxygen can form a double bond to carbon (Figure A). The carbon has to break its existing double bond to another oxygen to ensure it follows the octet rule. If carbon could not do this, then the resonance cannot occur.
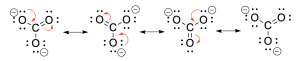
Misconceptions may occur with molecules that may appear non-aromatic, which need resonance for further evaluation of aromaticity. One such molecule is caffeine (Figure B).
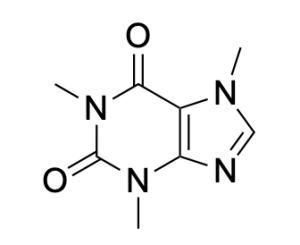
Caffeine appears non-aromatic in the six-membered ring structure, until its nitrogen lone pairs are considered. They can form double bonds, since the neighbouring carbon can lose a bond to the double-bonded oxygen (Figure C).
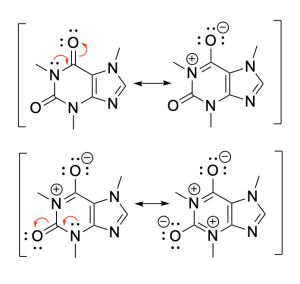
The resonance structure at the very end is aromatic, as evidenced in Figure D, with a simplified version of the ring structure indicating 10 π electrons. This follows Hückel’s rule. Despite the final structure having 4 formal charges, the structure’s aromaticity stabilization favours this conformation’s formation.
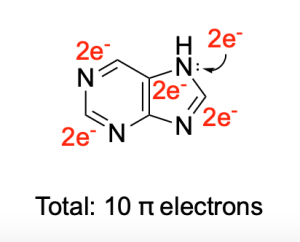
The following videos include two worked examples from a previous CHEM 1AA3 test or exam that students struggled with. Try solving them on your own before looking at the full solution.
Key Takeaways
- Aromatic structures exhibit different reactivity than alkenes, as they are more stable and resistant to addition, halogenation, oxidation and reduction reactions, which alkenes typically undergo.
- In benzene or aromatic compounds, each carbon atom contributes one p orbital and one electron, to form a single aromatic π orbital system which occupies the space directly above and below the plane of the ring.
- The electrons are considered delocalized as they form one large electron cloud and are free to move anywhere throughout the system. The movement is represented through curved arrows, and are referred to as resonance contributors.
- The three characteristics of an aromatic compound are: it is cyclic, it is sp or sp2 hybridized, and it follows Hückel’s rule.
- There are also anionic and cationic aromatic compounds where the extra electron (anionic) can contribute to an even number of pi electrons following Hückel’s rule, or the lack of an electron helps in maintaining Hückel’s rule and allowing for an empty p-orbital to interact with the system allowing for electrons to be delocalized in the ring/move around.
- Heteroaromatics are aromatics containing heteroatoms, where the heteroatom contributes 0, 1, or 2 electrons to the pi orbital system, which can help in maintaining Hückel’s rule.
- Aromatic compounds are found in biological systems sue to their stability, especially DNA, as the nitrogenous bases (adenine, thymine, cytosine, guanine) are made of heteroaromatics.
Key terms in this chapter:
Key term | Definition |
Aromatic compound | A property of certain molecules that make it extremely stable due to the delocalization of the electrons located in the p orbitals, forming an aromatic π orbital system. There are three criteria for a molecule to be aromatic. It must (1) contain a ring structure, (2) be comprised of either sp2-hybrdized or sp-hybridized atoms, and (3) follow Hückel’s rule. |
Angstrom | A unit of length, equal to 0.1 nanometer. |
Hückel’s rule | 1 of the 3 requirements of aromaticity which represents the amount of π electrons needed to maintain aromaticity calculated by the formula 4n+2 where n represents a non-negative whole number (0, 1, 2…). |
Heteroatoms | An atom other than carbon, such as oxygen, nitrogen, and sulfur. |
Diversity in Chemistry: Rosalind Franklin
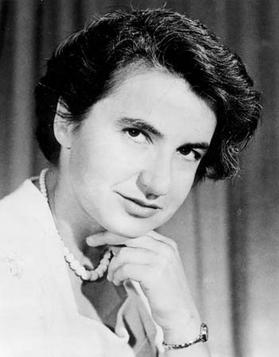
The aromaticity of the nitrogenous bases in DNA gives it a highly planar structure, which allows it to form the double helix structure. This structure was elucidated in 1953 by the pair of scientists James Watson and Francis Crick, winning them the Nobel Prize in Medicine in 1962. However, unbeknownst to many, Rosalind Franklin, a British-Jewish chemist, contributed heavily to this discovery with her role in this research going unrecognized for most of her life. Franklin was a scientist at King’s College in London, using X-ray diffraction to study and deduce the structure of DNA. Alongside herPh.D student, Franklin took the famous Photo 51, an X-ray image capturing the helical structure of DNA in its B-form. This, alongside an unpublished report with her conclusions, was shown to Watson and Crick. These helped facilitate the discovery of the DNA double helix model, with the pair going as far as saying that this discovery would not be possible without Franklin’s data. Many have agree that she should have been awarded a part of Nobel Prize (which did not give out posthumous awards), with her contributions being a hot topic of discussion (there is even a musical about her titled “Double Helix” performed at Bay Street Theatre in New York!). More information on Rosalind Franklin can be found on her biography page on the Rosalind Franklin University of Medicine and Science website.
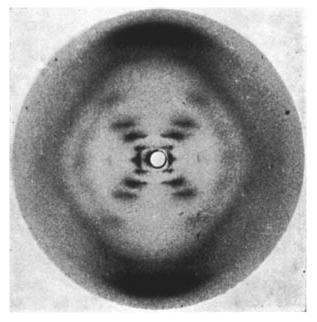
Below is an snapshot of Franklin’s academic tree, showing connections to other scientists discussed in the textbook.
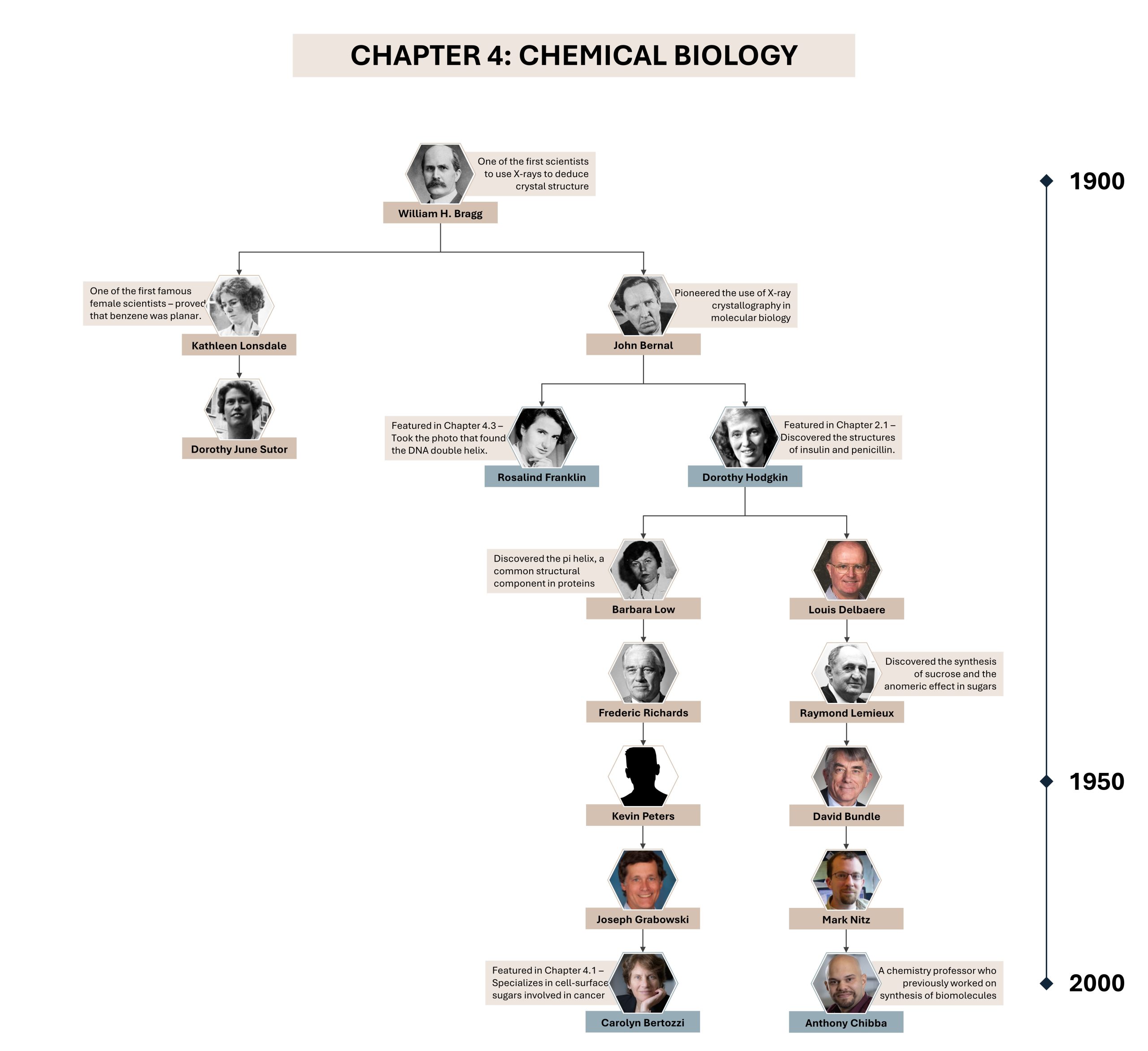
The rise of women science still came with its difficulties, however. Aside from Rosalind Franklin’s photo being infamously used without her consent, Dr. Dorothy Sutor, another female X-ray crystallography, was publicly shamed after publishing papers proving that hydrogen bonds could be formed bonding to hydrogens bonded to carbon atoms, which was written off and claimed to have “data problems”. Her work was only recognized 50 years down the road. More information on Sutor and other similar women can be found here and here.
Any feedback or comments on this chapter? You may either email chemoer@mcmaster.ca, access this MS Form, or provide a comment in the feedback box below.
A property of certain molecules that make it extremely stable due to the delocalization of the electrons located in the p orbitals, forming an aromatic π orbital system. There are three criteria for a molecule to be aromatic. It must (1) contain a ring structure, (2) be comprised of either sp2-hybrdized or sp-hybridized atoms, and (3) follow Hückel’s rule.
A unit of length, equal to 0.1 nanometer.
1 of the 3 requirements of aromaticity which represents the amount of π electrons needed to maintain aromaticity calculated by the formula 4n+2 where n represents a non-negative whole number (0, 1, 2…).
An atom other than carbon, such as oxygen, nitrogen, and sulfur.