4.2 – High-Throughput Screening
High-Throughput Screening
With thousands of potential compounds to test each day, scientists require methods to quickly test multiple compounds simultaneously to expediate the process. This is a process known as high-throughput screening (HTS). In HTS, robotics and automated processes are utilized to run multiple assays in parallel to determine whether a compound has the potential to be used as a therapeutic.
There are two characteristics of HTS which increase screening efficiency: the development of a fast assay, and the ability to perform parallel assays. To be useful in drug development, assays must be fast so that researchers can efficiently characterize various compounds. Using HVA as an example, viable drug candidates must replicate the effect of HVA on bees in a hive. While it is possible introduce a compound into a beehive to test its influence on worker bee dopamine levels, it would take weeks to properly observe the effects of these compounds, and you would need a separate hive for each test candidate. This is an ineffective way to screen thousands of compounds. Instead, bee neural cells can be grown in the lab and tested on directly. This approach isolates the biological component of bees that scientists are interested and allows for a streamlined testing process. The potential drug candidates can then be assayed with the neural cells to determine whether they inhibit dopamine production.
The second characteristic of HTS is the ability to perform parallel assays, where multiple compounds can be tested simultaneously for their therapeutic potential. This is commonly done in multi-welled plates (Figure 4.2.a). These plates contain many wells which can all be analyzed simultaneously. Each well represents a single experiment and contains a combination of a different drug candidate and bee neural cells to determine the compounds effect on dopamine production. This way, many different compounds can be tested simultaneously. In addition, the experiments are performed at small volumes (< 0.5 mL per well), resulting in a minimum of resources required to perform each experiment. This is especially beneficial considering the costs associated with harvesting bee neural cells and producing large libraries of test compounds.
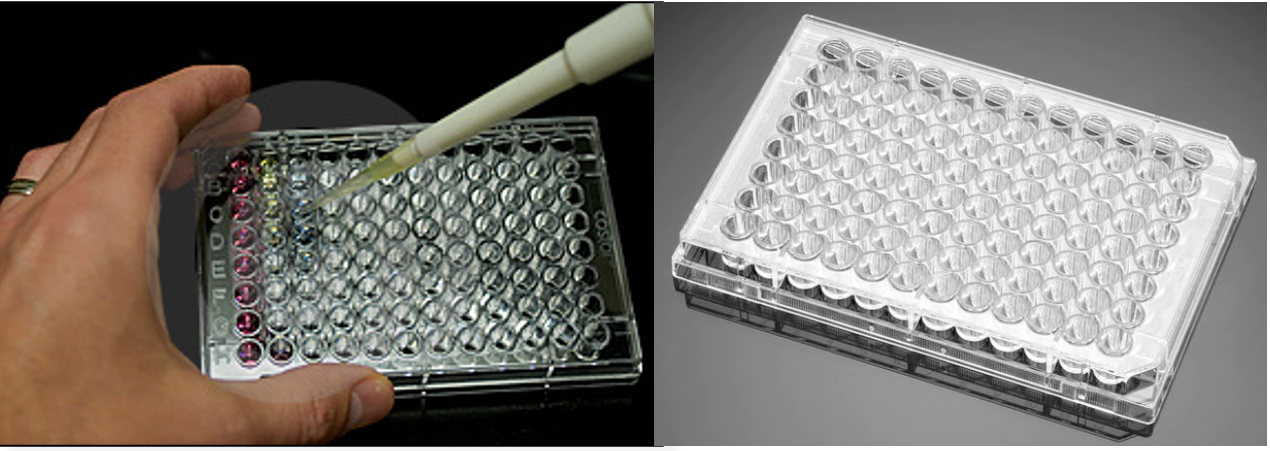
Enzyme-linked Immunosorbent Assays
An enzyme-linked immunosorbent assay, commonly referred to as an ELISA, is a type of HTS assay that can identify the presence of a specific molecule. These assays utilize a series of protein interactions to 1) bind to a molecule of interest, 2) identify that the molecule was present and bound in step (1), and 3) visualize the presence of the molecule through a colourimetric change.
Returning to the example using HVA, ELISAs are used to visualize whether test compounds altered dopamine production levels in bee neural calls (Figure 4.2.b). For this purpose, bee neural cells are cultured in a lab, and then added to a 96 well plate. The drug candidates can be assayed with the cells, with a different candidate added into each well. In this assay, a primary antibody, is added to each well which selectively binds dopamine with high affinity. The well is then washed to ensure that only primary antibodies bound to dopamine remain present in the wells. A second antibody is subsequently added, which is covalently bound to the enzyme horseradish peroxidase (HRPase). This secondary antibody binds to the first antibody, which is bound only to dopamine. The wells are then washed again to remove any unbound secondary antibody. In the final step, a blue substrate is added into the wells. This substrate reacts with HRPase to form a green product that is detected using UV absorbance.
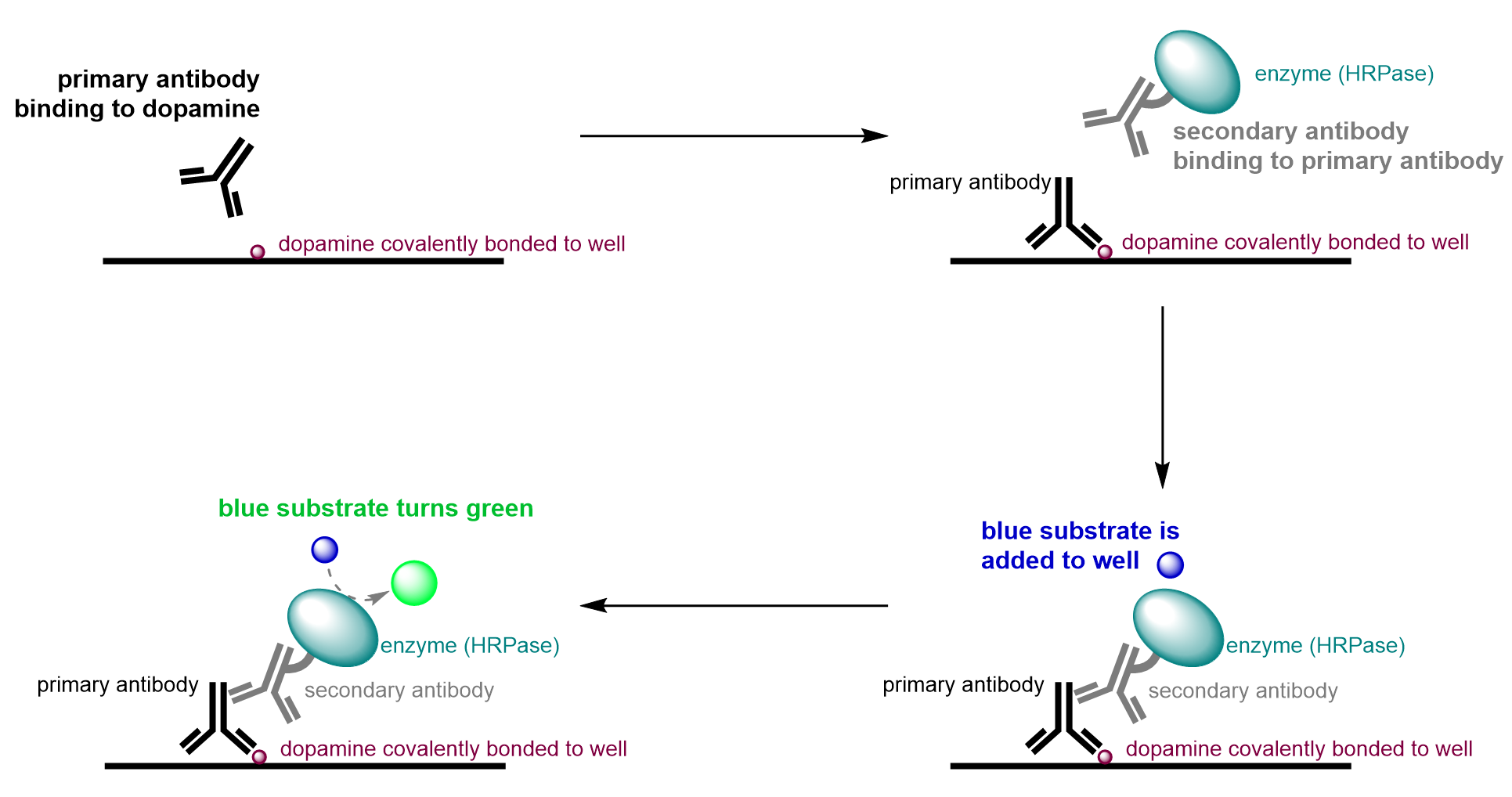
Given the experimental design, HRPase must be present to convert the blue substrate to a green product. Since the presence of the HRPase linked secondary body is dependent on the presence of the primary antibody, which in turn is dependent on the presence of dopamine, wells only appear green if dopamine is present. If dopamine is not present, there will be no binding cascade of antibodies, and the well will remain blue. This is because the primary antibody will have no dopamine to bind to in the first step and will therefore be removed when washing. Thus, the secondary antibody linked with HRPase will have no binding partner as there is no primary antibody present and will also be washed away. Without the enzyme in the well, the substrate will remain blue as a result of the initial lack of dopamine in the well.
In this case, the therapeutic goal of this assay is to find a test candidate to inhibit dopamine production. Thus, the desired outcome is a blue colour. The well being blue tells us that the compound in the well is inhibiting dopamine production, since blue indicates there’s no dopamine present for any antibodies to bind to. A well that appears blue after completion of the assay is referred to as a hit. After the HTS stage, the compounds that generate hits will be used to inform the design of the next generation of drug candidates and may undergo more rigorous testing to determine other characteristics, such as molecular stability, and human toxicity and dosage.
Are You Wondering? The Binding Capabilities of Antibodies
The underlying principle of an ELISA is the ability of antibodies to bind with high selectivity to a molecule of interest. Antibodies are proteins produced by your immune system to identify foreign molecules and cells.
The function of an antibody is derived from its structure. They are composed of two main regions: a fragment crystallizable (Fc) region and a fragment antigen binding (Fab) region.
The Fab is highly variable and gives antibodies the ability to bind to virtually any molecule. In an ELISA, the Fab of the primary antibody is what recognizes and binds to the molecule of interest, such as dopamine.
The Fc region, in comparison, is highly conserved between antibodies of the same class, even if they have different Fab regions. In an ELISA, the conserved Fc region of the primary antibody is recognized by the Fab region of the secondary antibody. Because Fc regions are functionally identical for antibodies within the same class, the secondary antibody of an ELISA can be used to identify many primary antibodies.
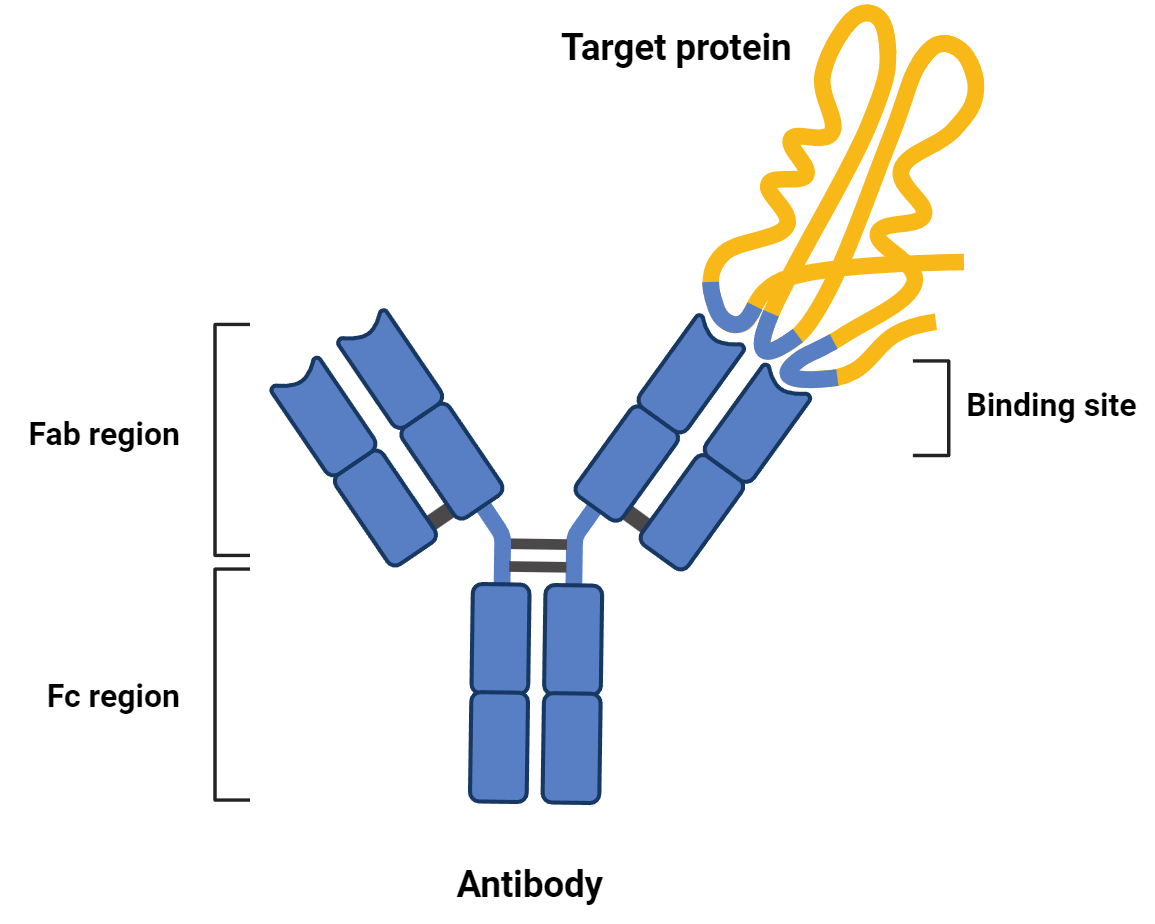
Setting Up Controls
When running any assay, it is of utmost importance to have controls. Control experiments ensure that the experimental design of an assay is sound and that the different components of the assay are working as intended. This ensures that the results of the assay are accurate, and not a result of interfering or unpredicted factors. Control experiments can contain different combinations of components used in the experiment and/or expected products from the assay. In most cases, many different control experiments are run for a given assay.
Controls can be categorized into two types: positive controls and negative controls. Positive controls are control wells that are expected to produce a positive signal, such as the colourimetric change from blue to green in the ELISA described previously. On the contrary, negative controls are expected not to produce any change in signal, such as no colour change from blue to green.
Figure 4.2.c represents a set of 6 control experiments used in the ELISA mentioned throughout this chapter. Each well contains a different combination of experimental components and is intentionally designed to validate different aspects of the experiment and that the measurement made matches expectation.
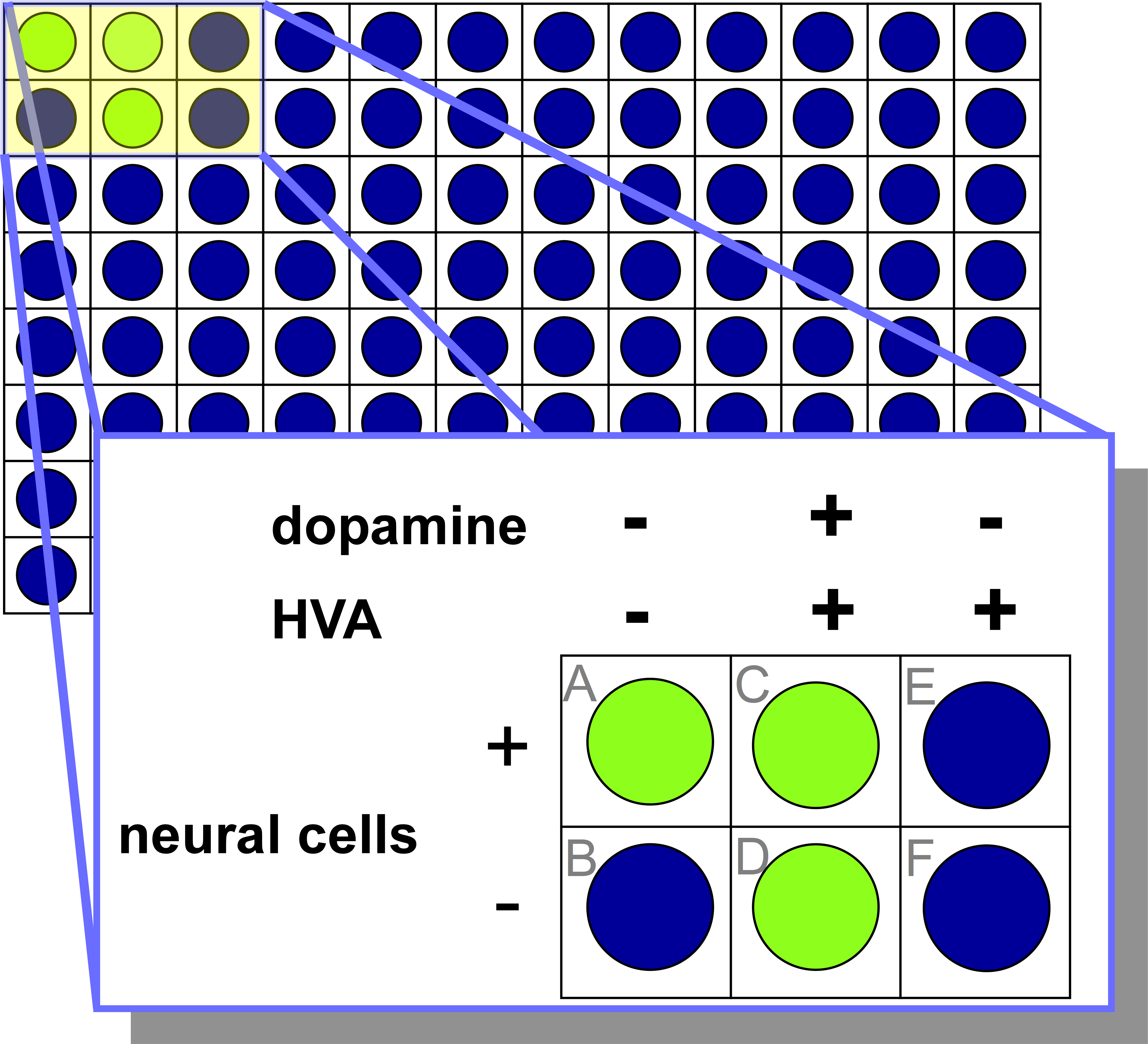
The control well labeled ‘A’ contains only bee neural cells and lacks HVA or exogenous dopamine. This well is a positive control as it is designed to ensure that the neural cells are naturally producing dopamine under the assay conditions, and that it is identifiable by the ELISA (green signal).
The control well labeled ‘B’ contains no dopamine, HVA, or bee neural cells. This well is a negative control, as it verifies that dopamine is required to produce a green signal. No dopamine will be present or produced in this well, and as a result, no green signal is produced. The blue colour of this well validates that the ELISA will not proceed if no dopamine is present.
The control well labeled ‘C’ contains bee neural cells, HVA and dopamine. Although HVA inhibits dopamine production in bee neural cells, the addition of exogenous dopamine in this well results in positive signal in the ELISA. This well is a positive control since green signal is detected and ensures that dopamine can be detected in the presence of both HVA and bee neural cells.
The control well labeled ‘D’ contains HVA and dopamine, and no bee neural cells. Since dopamine is added to this well, the expectation is that it will be detected by the ELISA and a positive signal will result. This well is a positive control since the green signal is observed and demonstrates that HVA does not interfere with the detection of dopamine by the ELISA.
The control well labeled ‘E’ contains both bee neural cells and HVA and has no exogenous dopamine. Since HVA is an inhibitor of dopamine in bee neural cells, the expectation is that the well will remain blue because no dopamine will be produced by the bee neural cells. This well is a negative control, since no signal is measured, and verifies that the dopamine production can be inhibited by HVA.
The control well labeled ‘F’ contains only HVA, and no dopamine or bee neural cells. Since there is no dopamine added, and no bee neural cells present to produce dopamine, the expectation is that there will be no signal measured through the ELISA. This well is designed to test whether HVA, a structure that is structurally similar to dopamine, could potentially bind to the primary antibody and result in the detection of a green signal. This well is a type of negative control to test that HVA alone does not elicit a signal in the ELISA.
Since the experimental measurements in Figure 4.2.c match the expectations, it can be concluded that the ELISA is working as expected. None of the components interfere with the assay in an unexpected manner, and this provides confidence that any results obtained from this assay will be accurate.
(The full solution to this problem can be found in Chapter 5.3)
Obtaining a Hit
Controls are experiments to ensure that the assay is working as intended, with positive denoting a positive signal (i.e. green color), and negative denoting a lack of signal (i.e. blue color). This has no correlation to what the desired results of the experiment are.
In contrast, hits are related to what the scientists want to see from the experiment. In this scenario, the assay is screening for molecules that can inhibit dopamine production – thus, the desired results are low dopamine concentration, denoted by a blue well. Because of this, a positive result means the desired outcome is seen, with low dopamine being represented by a blue well. In contrast, negative results mean the compound does not have any dopamine-reducing effects. This is the undesired outcome, with high dopamine represented by a green well. The terms ‘true’ and ‘false’ can also be applied to explain the validity of the results. True means that the results are caused by the drug candidate, whereas false means that factors other than the compound are leading to the observed results. Through the development of this highly efficient and automated process, thousands of compounds can be screened daily. With this technique, they can determine the most potent dopamine inhibitors from a large library of compounds for subsequent testing.
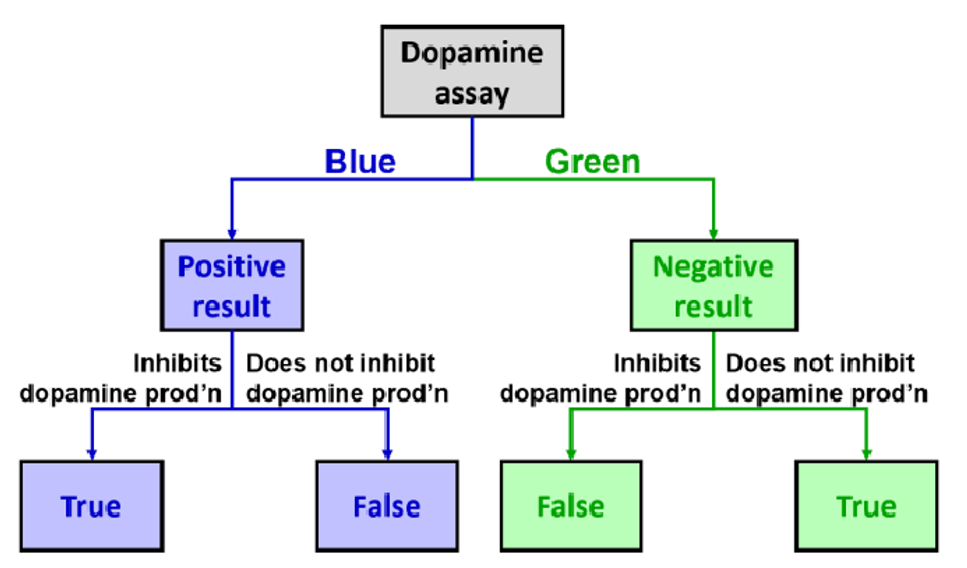
A true positive in the context of the ELISA is when a hit is obtained, and it is a result of the test compound inhibiting dopamine production in the bee neural cells in a similar fashion to HVA. In a true positive hit, the well will remain blue, as there is no dopamine in the well to be detected.
A false positive is when a hit is obtained, but it is not a result of the test molecule inhibiting dopamine production in the bee neural cells Instead, it may be for other reasons, such as a mistake in the assay design where a component was not added to the well, or the test compound prevents dopamine production through an alternative mechanism, such as through cytotoxic killing of the bee neural cells As the word “false” suggests, this drug candidate is not actually inhibiting dopamine production in the desired manner but the results suggest that it is.
It is also possible to observe wells that are the opposite to the desired results, which means the neural cells are producing dopamine as normal which we call a miss . These can also be classified into either true or false negatives, as these are undesirable results.
A true negative is a well that produces negative results, meaning it does not result in the desired effect. The well would appear green as dopamine is produced normally. This is because of the compound itself, as it has no inhibitory properties.
A false negative is a well that appears to produce a negative result, but in reality contains a test compound that is capable of inhibiting dopamine production. This type of result could be due to a litany of reasons; a contamination of dopamine in the media, or the antibodies binding to something other than dopamine both represent scenarios where the ELISA results suggest dopamine is present even when the test compound inhibits dopamine production in bee neural cells. As the word “false” suggests, the results incorrectly suggest the drug candidate is responsible for the green colour.
Are You Wondering? The True and False Results of COVID-19 Rapid Antigen Tests
By 2023, almost every student has likely contracted SARS-CoV-2, also known as COVID-19. These are typically discovered via take-home rapid antigen kits and are performed by the students themselves. Although the mass production and distribution of these kits assisted in decreasing the pressures on the healthcare industry, the tests are not infallible. These terms for determining hits from an assay can similarly be applied to COVID tests.
For example, a true positive is when you test positive on the kit, and you are actually positive for COVID. Similarly, in a dopamine assay, positive results are seen, and it is actually positive due to the effects of the compound. On the contrary, a false positive is when you test positive for COVID, but you do not have the virus. You would assume that there is some mistake with the test – a similar thing can occur in the HVA assay. You can see positive results in the dopamine assay, but it is caused by another variable with the compound not having any dopamine-reducing effects.
A true negative on a COVID test occurs when you test negative for the virus and you are not sick. This term can also be used in the HVA assay, as it means there are negative (undesirable) results, which are truly because of the compound’s characteristics. A false negative COVID test means that you test negative on the kit, but in actuality, you have the virus. There was some sort of other problem with the COVID test that gave you the wrong results – the same theory applies to scientific assays. A false negative could occur as the well could turn green to indicate high dopamine, which is not the desired results, but it is due to another variable and not because of the properties of the compound itself.
The following videos include two worked examples from a previous CHEM 1AA3 test or exam that students struggled with. Try solving them on your own before looking at the full video solutions.
(The full solution to this problem can be found in Chapter 5.3)
Key Takeaways
- ELISA is a type of assay used in HTS to determine the absence or presence of a compound of interest colourmetrically. ELISA works with the following mechanism:
- A primary antibody (Antibody 1) is added to specifically binds to the compound of interest.
- A secondary antibody (Antibody 2) is introduced and binds to Antibody 1. Antibody 2 is itself bound to an enzyme, horseradish peroxidase, which changes the colour of an added substrate from blue to green.
- This chain indicates the presence of the desired molecule from any colour changes that occur.
- The steps of ELISA process are as follows:
- Introduce Antibody 1, which binds to the compound of interest.
- Wash any unbound leftover antibody.
- Introduce the Antibody 2-horseradish peroxidase compound.
- Wash any unbound leftover antibody again.
- Control experiments are necessary to ensure the experiment is working as intended, and can be categorized as either positive or negative controls. Positive means positive outcome (colourimetric change) is expected, while the opposite is true with a negative control: a negative outcome (no colourimetric change) is expected.
- True vs False Negatives and Positives: True positives and true negatives are both “true” outcomes of an experiment, which accurately determine the effects of the drug candidate, and lead to the desired results and undesired results respectively. False positives and false negatives are results falsely being observed as a desired or undesired outcome due to other variables or experimental error.
Key terms in this chapter:
Key term | Definition |
Assay | An experiment used to measure the activity or presence of a molecule. |
High-throughput screening | A process used to screen many compounds of their therapeutic potential. Automated and robotic processes are utilized to run multiple assays in parallel, or all at once, to determine this. The two characteristics of this process is that it uses fast assays and that it uses massively parallel assays, where the large number of wells and automated processes enable this to occur. |
Enzyme-linked immunosorbent assay | Also known as an ELISA, it is a type of assay used to determine if a molecule of interest is present through colourimetric change. The assay works using horseradish peroxidase as a coloured indicator, which is bound to a secondary antibody. This secondary antibody will bind to a primary antibody that is specific for the molecule of interest. ELISAs are used in high throughput-screening as they can be performed in 96-well plates and give a coloured response to quickly determine the presence of a molecule |
Control experiments | Control wells performed alongside the actual experiment to ensure that the experimental design is sound and that the different components of the assay are working as intended. The controls ensure experimental reliability, and that results are not due to other interfering or unpredictable factors. Control experiments may have combinations of components used in the experiment or expected products from the assay, and contain both a positive and negative control. |
Positive controls | A control well expected to produce a positive signal. For example, in the ELISA assay, the colourimetric change from blue to green is a positive signal. |
Negative controls | A control well expected to produce a negative signal. For example, in an ELISA assay, the lack of colour change is considered a negative signal for controls. |
Hits | A compound in an assay which has been determined, through the assay, to have a desired effect. |
Miss | A compound in an assay which has been determined, through the assay, to have a non-desired effect. |
Diversity in Chemistry: From Yalow to Milstein
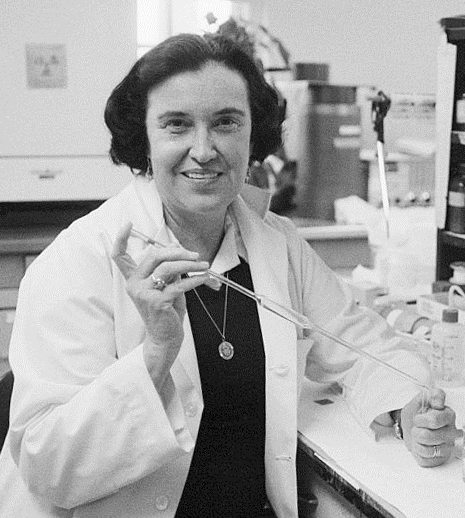
Assays are not only limited to being used for determining potency of potential drug candidates. There are numerous other assays developed for high through-put screening for other purposes, such as identifying analytes in a complex solution. Rosalyn Yalow, a Jewish-American scientist, was the second woman to win a Nobel Prize in Medicine in 1977 for her groundbreaking development of a new assay. Termed a radioimmunoassay, she developed a method of using radioactive isotopes to identify and measure minute concentrations of analytes in blood, such as proteins, vitamins, and drugs, measuring as low as the picogram range. This technique was first applied to determine the cause of type II diabetes, using radioactive iodine binded to insulin, as she had her own personal interest in this condition as her own husband had diabetes. Yalow succeeded in a period where women were undermined for their scientific abilities, with a young Yalow considering pursuing a career in teaching instead of science. More information on Rosalyn Yalow can be found on the Nobel Prize website.
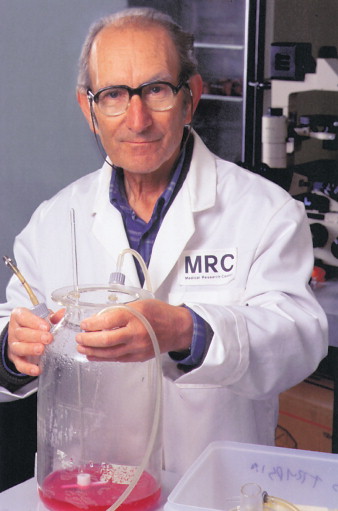
Antibodies are commonly employed everywhere for many purposes, whether it be for radioimmunoassays, ELISAs or the commonly used COVID-19 rapid antigen tests. But how exactly are they produced for these purposes? Thank César Milstein, an Argentinian-born British biochemist. In 1975, Milstein, alongside a postdoctoral student in his lab, developed a novel method for the mass production of monoclonal antibodies called the hybridoma technique. This led to the expansion of antibody use in both diagnostics and therapeutics, winning him the 1984 Nobel Prize in Medicine. This technique involved the development of hybrid cells. By taking the antibody-producing immune cells (called B cells) out of a mammal and fusing it with immortal cells that reproduce indefinitely, they could harvest a theoretically infinite number of antibodies. Aside from his scientific achievements, Milstein devoted himself to help advance science in developing countries, and never patented his hybridoma technique as he believed it was mankind’s intellectual property. More information on César Milstein can be found on his biography page on the American Association of Immunologists page.
Any feedback or comments on this chapter? You may either email chemoer@mcmaster.ca, access this MS Form, or provide a comment in the feedback box below.
A process used to screen many compounds of their therapeutic potential. Automated and robotic processes are utilized to run multiple assays in parallel, or all at once, to determine this. The two characteristics of this process is that it uses fast assays and that it uses massively parallel assays, where the large number of wells and automated processes enable this to occur.
Also known as an ELISA, it is a type of assay used to determine if a molecule of interest is present through colourimetric change. The assay works using horseradish peroxidase as a coloured indicator, which is bound to a secondary antibody. This secondary antibody will bind to a primary antibody that is specific for the molecule of interest. ELISAs are used in high throughput-screening as they can be performed in 96-well plates and give a coloured response to quickly determine the presence of a molecule
Control wells performed alongside the actual experiment to ensure that the experimental design is sound and that the different components of the assay are working as intended. The controls ensure experimental reliability, and that results are not due to other interfering or unpredictable factors. Control experiments may have combinations of components used in the experiment or expected products from the assay, and contain both a positive and negative control.
A control well expected to produce a positive signal. For example, in the ELISA assay, the colourimetric change from blue to green is a positive signal.
A control well expected to produce a negative signal. For example, in an ELISA assay, the lack of colour change is considered a negative signal for controls.
A compound in an assay which has been determined, through the assay, to have a desired effect.
A compound in an assay which has been determined, through the assay, to have a non-desired effect.