4.1 – Overview of Chemical Biology
What is Chemical Biology?
A thorough understanding of chemistry is helpful to solve numerous problems in other science disciplines. One of the major applications of chemistry is to biological systems. This discipline is called chemical biology. Chemical biology is a rapidly growing field that does not have a precise definition. However, the main characteristic of chemical biology is using principles of chemistry to solve complex biological problems.
When the field of chemical biology first developed, the primary goal was to use small molecules to interact with biological systems such as proteins, nucleic acids, or other cellular components (Figure 4.1.a). The goal was to modulate the behavior of these biological systems to explore how the cellular system could be perturbed. This way, scientists could better understand the underlying biochemistry of cellular processes, or change biological outcomes to aid in drug discovery. The scope of the field has now greatly expanded, including novel research such as the synthesis of chemically modified proteins and DNA, alongside imaging and diagnostics.
We will highlight principles and techniques of chemical biology through the following case study. Specifically, we will examine how the field of chemical biology draws its inspiration from nature, and how we can use natural phenomena to develop new therapeutics to treat diseases.
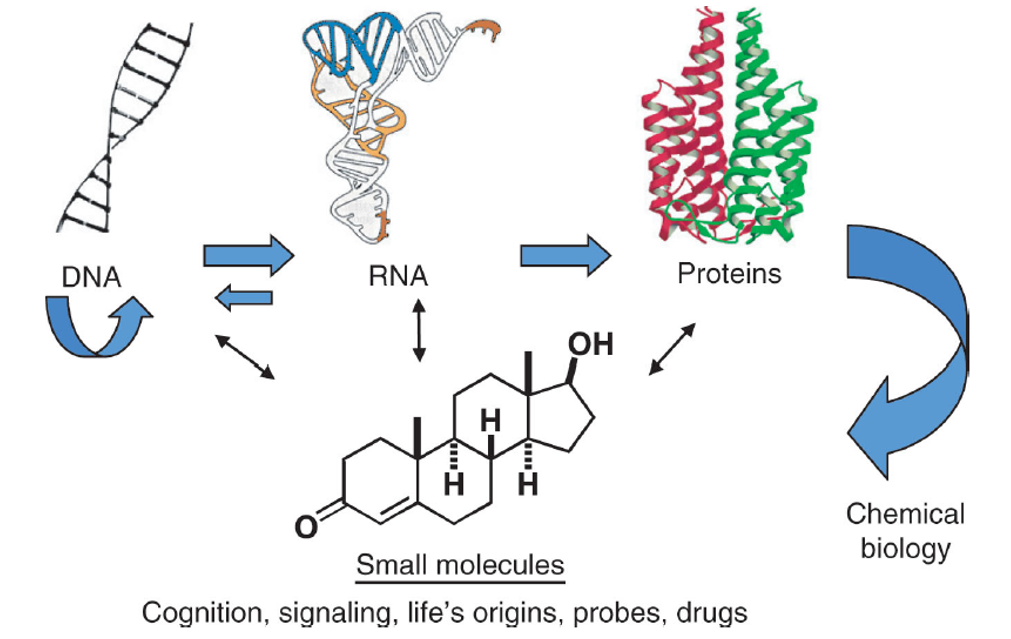
Case Study: The Brainwashing of Bees
This case study explores the social structure of bee colonies. In a bee colony, there is a single queen bee, many drones (males) and many worker bees (female). The drones’ main function is to fertilize the queen and then die instantly. The worker bees are responsible for building and maintaining the hive, gathering resources, and ensuring the survival of the queen.
The dominance of the queen bee and the hierarchy of the colony is established and maintained through a mixture of chemicals, called the Queen Mandibular Pheromone (QMP). QMP is secreted from the jaw of the queen bee and exerts several effects on the bees in the colony. For example, QMP attracts young worker bees to the queen, so they can groom and feed her. In turn, they gather samples of QMP and spread it throughout the colony. QMP also inhibits rearing of new queen bees, influences comb-building, and controls worker bees’ development.
Dopamine (Figure 4.1.b, right) is a neurotransmitter involved in the “reward center” of the brain, controlling mood, attention, and memory. In insects, dopamine signaling is necessary for learning how to avoid unpleasant stimuli, called aversive learning. However, when young worker bees are exposed to QMP, their brain dopamine levels, as well as their expression of the dopamine receptor gene, are altered.
In Vergoz et al. (2007), researchers sought to understand: (1) whether exposure to QMP alters young worker bees’ ability to establish aversive memories; (2) what aspect of QMP is responsible for these effects. If you would like to learn more about this study, click on the following link to access the journal article: https://doi.org/10.1126/science.1142448.
QMP contains several compounds, with the most vital compound to ensure the survival of the queen being homovanillyl alcohol (HVA) (Figure 4.1.b, left). The structure of HVA is quite similar to the structure of dopamine (Figure 4.1.b, right). HVA contributes to the effects of QMP on dopamine signaling in the brains of worker bees. Researchers found that HVA impairs the formation of aversive memories in young worker bees. In contrast, older worker bees, who are responsible for leaving the hive to forage, are unaffected by HVA, and can establish aversive learning.
The researchers postulated that because high exposure to QMP leads to unpleasant side effects, blocking aversive memories ensures that young workers will not associate QMP with these unpleasant side effects. As mentioned earlier, dopamine plays an important role in the formation of averse memories. As HVA is structurally very similar to dopamine, it can interfere with dopamine binding to its receptor by taking the place of dopamine in the dopamine-receptor binding interaction, resulting in a lack of aversive memory formation . In short, the researchers established that HVA is the component of QMP that allows young worker bees to be “brainwashed” by the queen. The young worker bees do not establish aversive learning and are less inclined to flee from the unpleasant experience of QMP. This results in the development of the social hierarchy necessary for hive function and survival of the colony.
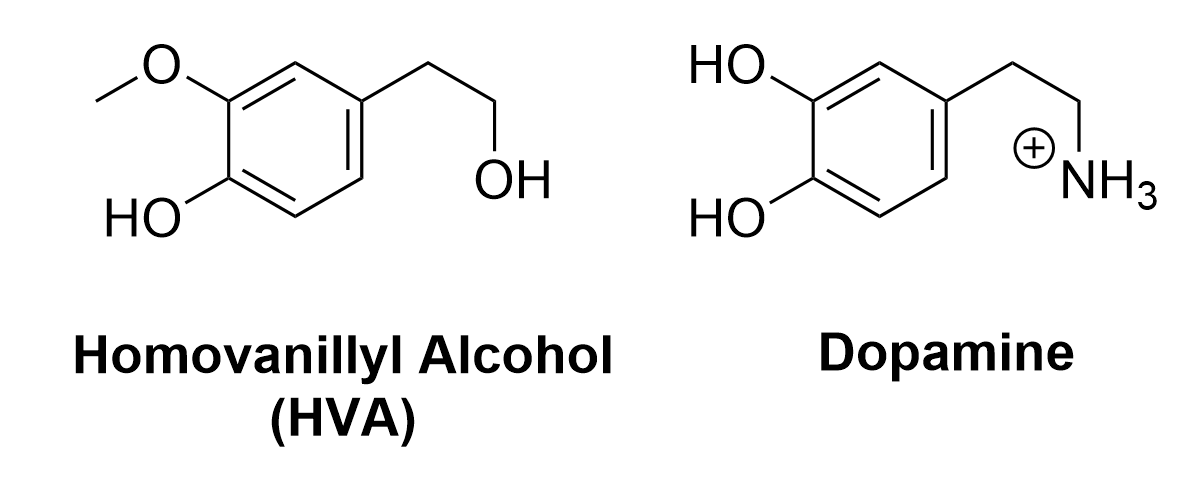
The Therapeutic Potential of HVA and HVA-like Compounds
Queen bees have a selective way to control young worker bees’ memories, for a short part of their lifespan. Understanding this phenomenon presents opportunities for HVA or HVA-like molecules to be used as therapeutics for altering dopamine levels in animals, including humans.
However, altering dopamine levels in the brain is not an easy task. For example, several health conditions are characterized by abnormally high dopamine concentrations, such as schizophrenia and certain kinds of psychoses. At the same time, numerous diseases are characterized by abnormally low concentrations of dopamine, such as Parkinson’s Disease and Attention Deficit Disorder. A therapeutic agent designed to suppress dopamine concentrations must be specific so that it only slightly suppresses dopamine concentrations, or so that it does so only in a certain area of the brain.
The Drug Discovery Process
The case study described here uses experiments on live animals, with replicate measurements, and waiting periods between experimentation. Instead, we wish to synthesize and screen thousands of drug candidates in a fast and efficient manner. This way, we can discover the most effective therapeutics for a target goal, like reducing dopamine levels.
For every successful drug that enters the market, there are thousands of unsuccessful drugs that were synthesized and screened for activity. The drug discovery pipeline is a lengthy process which requires a lot of time and resources to produce effective and safe medications for public use. The typical strategy for the initial discovery of new drugs tends to follow a three-step process, shown below in Figure 4.1.c.
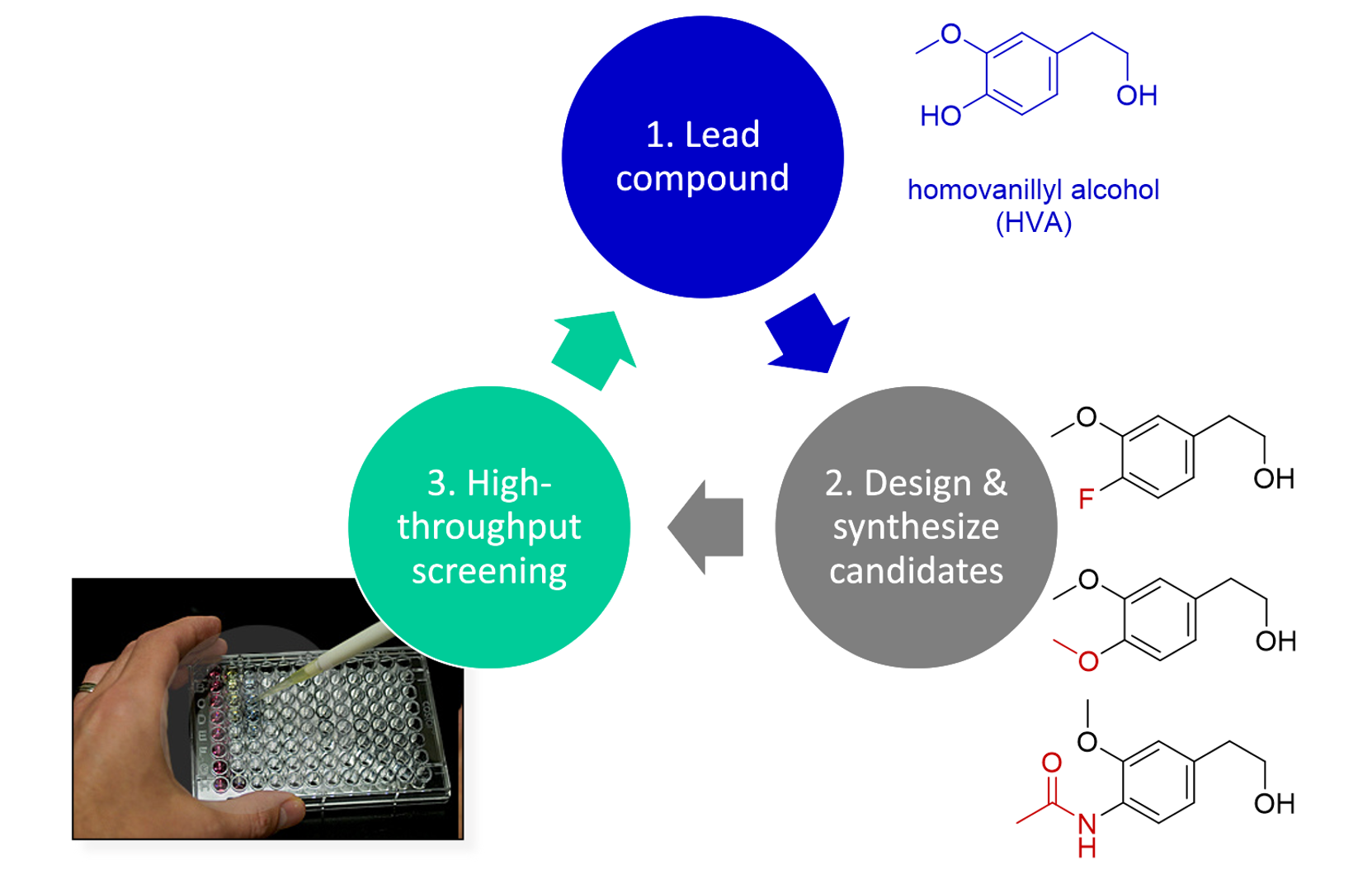
The first step is to obtain a lead compound. A lead compound is some structure which has an effect on the biological target of interest. It can either be an existing drug that was synthesized for a different purpose, or a product that is found in nature such as homovanillyl alcohol (HVA). HVA has been shown to decrease dopamine production in bee neural cells. Due to the potential therapeutic applications of lowering dopamine levels, HVA is used as a model compound in the drug discovery process.
The second step is to synthesize a library of compounds to act as candidates to test for drug-like activity. These are typically modelled after the lead compound and contain similar core structures in hopes of eliciting a similar or improved response. The library of candidates shares the same core structure, but they have variation in their outer functional groups. This allows scientists to determine which combination of functional groups produce the optimal response from the target. This is done through a process known as combinatorial chemistry.
The third step is to test the library of compounds to see if the desired effect can be obtained. This is typically done through a form of in-vitro experiments known as assays. An assay is an experiment that seeks to measure the activity or presence of a molecule. One approach for performing assays is through a high-throughput screening process that allows the rapid and efficient testing of a variety of compounds in parallel.
The following chapters will discuss the chemical biology principles involved in achieving this goal, including:
- Designing experimental protocols with a multitude of control experiments – Chapter 4.2
- Efficiently testing several drug candidates using high-throughput screening – Chapter 4.2
- Exploring common structural features of drug candidates such as aromaticity – Chapter 4.3
- Synthesizing a large library of compounds using combinatorial chemistry – Chapter 4.4
Diversity in Chemistry: Carolyn Bertozzi
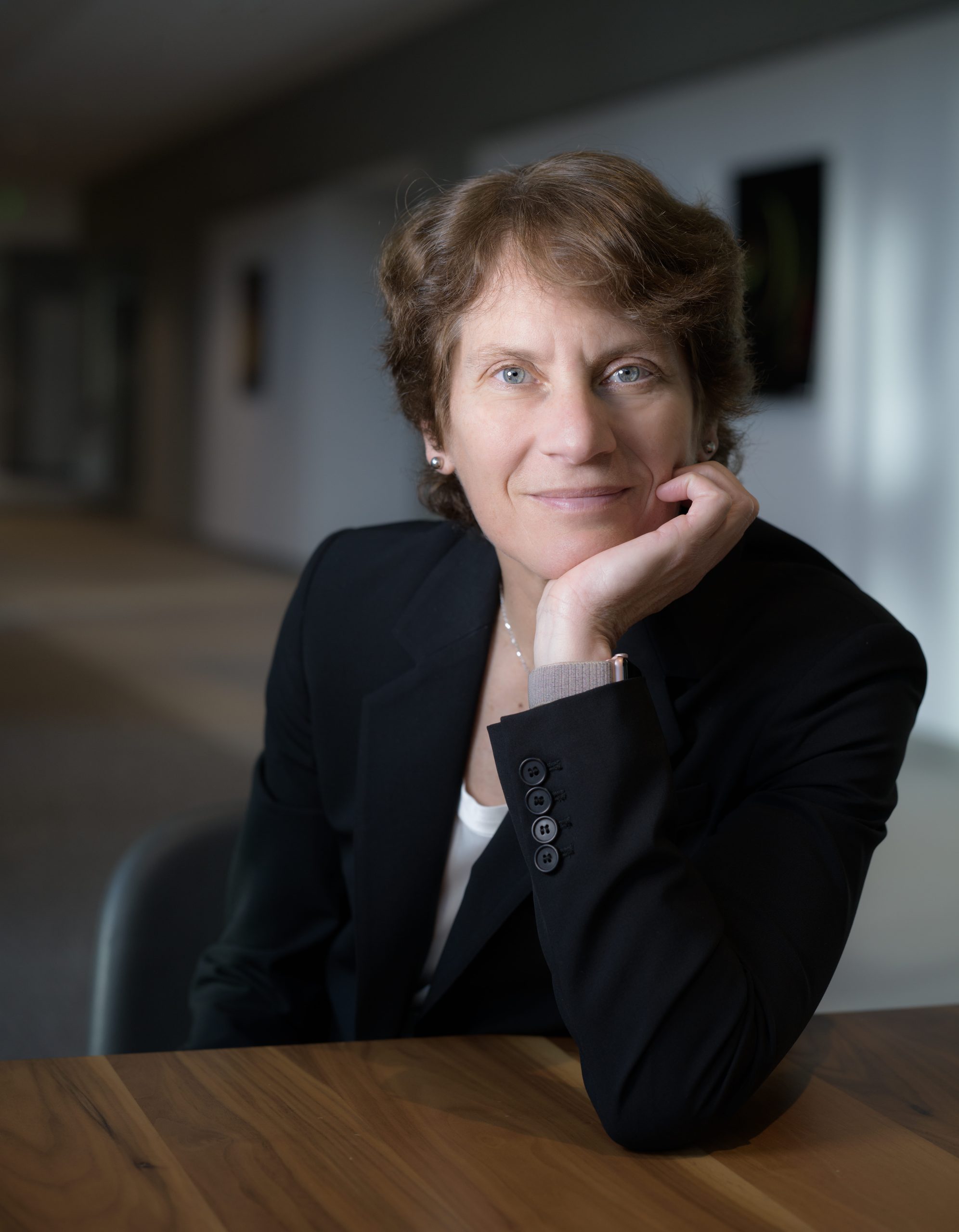
In order to follow a target of interest within a cell or living system, a molecule known as a probe must react with it. This reaction must occur in a busy environment, with hundreds of other cell components present, and it must not interact or interfere with normal cell processes. This is a subfield of chemical biology known as bio–orthogonal chemistry. This field was developed by scientist Carolyn Bertozzi in 1999, with the name given in 2003. Bertozzi is a glycobiologist who studies oligosaccharides (polymeric sugars) found on the cell surface, and studies how they act when affected by disease. She was awarded one third of the 2022 Nobel Prize in Chemistry for her developments in bio-orthogonal chemistry, developing the widely used strain promoted alkyne azide cycloaddition. This is an extremely favorable and quick reaction that forms no byproducts using relatively rare functional groups not found in cells, thus, not perturbing the cell environment. Bertozzi is currently a chemistry professor at Stanford University and is an open member of the LGBT community, acting as a role model in academia and science. More information about Bertozzi can be found on her profile on the Proceedings of the National Academy of Sciences (PNAS) website.
Key Takeaways
Key terms in this chapter:
Key term | Definition |
Chemical Biology | A scientific field between branching chemistry and biology. The discipline often involves the application of chemical techniques, analysis, and small molecule to the study and manipulation of biological systems |
Pheromone | A chemical that is secreted and released outside the body to affect individuals of the same species. These tend to trigger a specific response in the receiving individual, often causing hormonal or behavioural changes. |
Lead compound | A compound that already exists and its effects are well characterized. It is used to derive novel compounds to test for a desired therapeutic effect. This is done by changing the lead compound’s functional groups. |
In-vitro experiments | Experiments that are conducted in cells or microorganisms outside of their normal biological context. This includes cells grown in a well plate or flasks. |
Assay | An experiment used to measure the activity or presence of a molecule. |
High-throughput screening | A process used to screen many compounds of their therapeutic potential. Automated and robotic processes are utilized to run multiple assays in parallel, or all at once, to determine this. The two characteristics of this process is that it uses fast assays and that it uses massively parallel assays, where the large number of wells and automated processes enable this to occur. |
Any feedback or comments on this chapter? You may either email chemoer@mcmaster.ca, access this MS Form, or provide a comment in the feedback box below.
A chemical that is secreted and released outside the body to affect individuals of the same species. These tend to trigger a specific response in the receiving individual, often causing hormonal or behavioural changes.
A compound that already exists and its effects are well characterized. It is used to derive novel compounds to test for a desired therapeutic effect. This is done by changing the lead compound’s functional groups.
Experiments that are conducted in cells or microorganisms outside of their normal biological context. This includes cells grown in a well plate or flasks.
An experiment used to measure the activity or presence of a molecule.