3.6 – Synthesis
Through stepwise reactions, organic chemists can take a simple molecule and add different functionalities to make a single, complex molecule. In this chapter, we will examine how to synthesize more complex molecules through one or more reactions, using the organic reactions we have covered so far in this course (Figure 3.6.a).
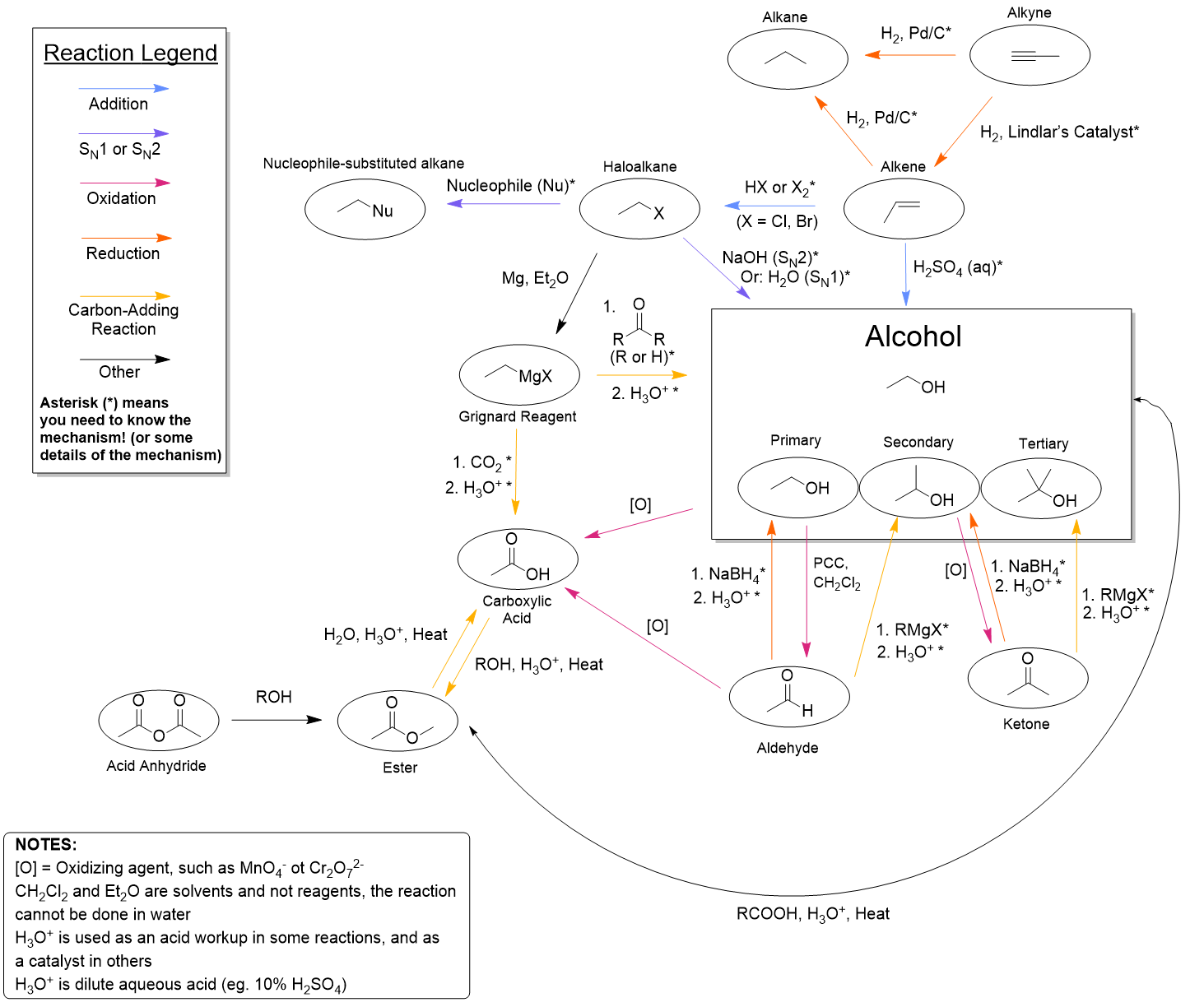
Reactions can be classified roughly into two different categories: (1) functional group interconversion reactions and (2) skeletal structure expansion reactions.
Most of what is covered in this course is categorized as functional group interconversion reactions. These reactions take one functional group, such as an alcohol, and convert it to something else, such as a carboxylic acid.
Reactions that expand the skeletal structure increase the number of carbon atoms in a molecule. The Grignard reaction is one example, which results in the formation of a new carbon-carbon bond. Esterification is another example as it introduces more carbon atoms. Note that reactions which expand the skeletal structure may also involve a change of functional group. For example, the Grignard reaction not only forms a new C–C bond, but also reduces a carbonyl group to an alcohol. In addition, esterification changes a functional group (carboxylic acid and alcohol, or carboxylate and alkyl halide) to an ester.
Some students find synthesis questions difficult due to the sheer number of reactions. Here are some tips to consider when approaching any synthesis question:
- Count the carbons
- Identify changes in functional groups
- Work backwards
- Consider a series of reactions
Count the Carbons
The first step when approaching any synthesis question is to count the number of carbons. Take note whether the number of carbons on the molecule has changed. If there are more carbon atoms in the product compared to the reactant, then (at this point) you can assume that it must have occurred through (1) a Grignard reaction or (2) an esterification reaction.
A sample question is shown in Figure 3.6.b.i.
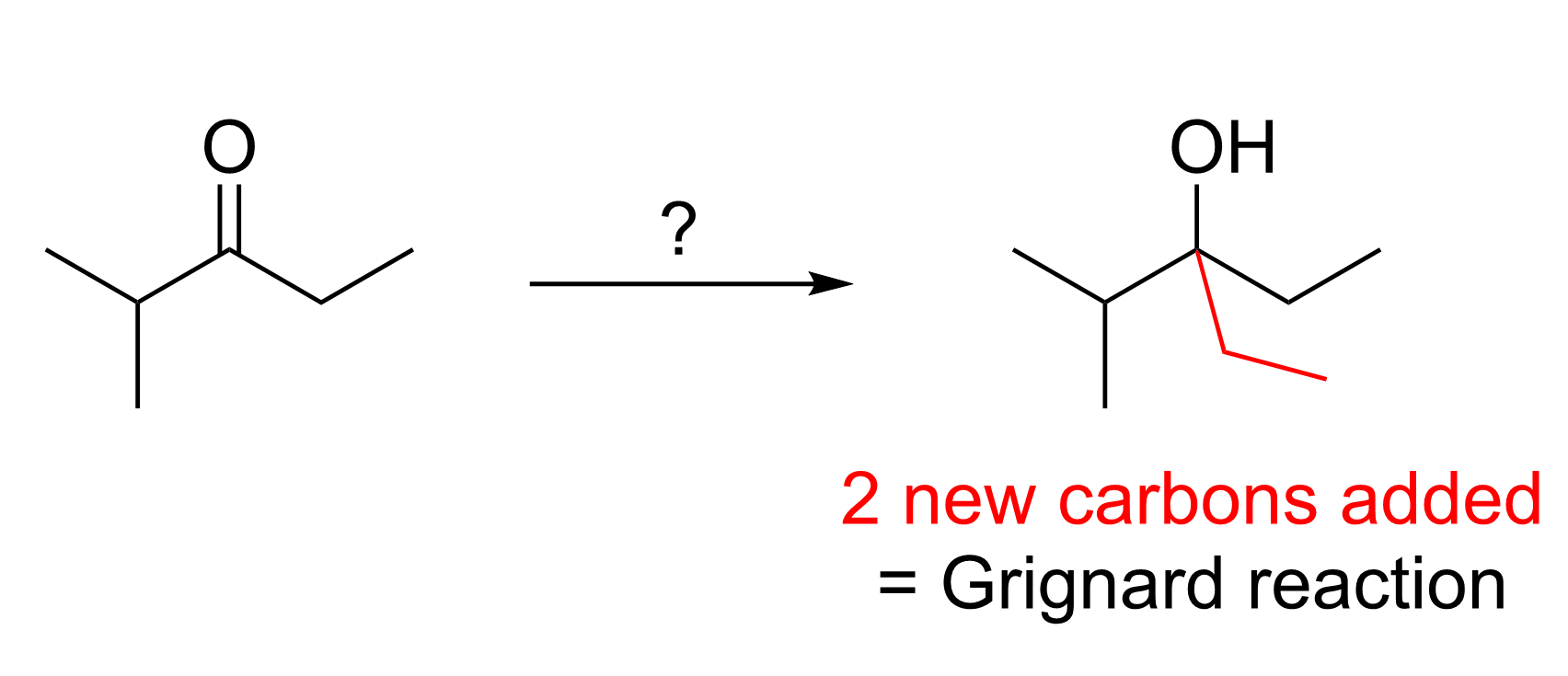
In this example, we can see that there has been the addition of two carbons to the starting substrate. As we only know one C–C bond forming reaction, we can conclude that a Grignard reaction must have taken place. A Grignard reaction uses a Grignard reagent (R–MgX), which contains a nucleophilic carbon centre, to attack a carbonyl center of an aldehyde or ketone, forming a new C–C bond.
Since two new carbon atoms (plus five attached hydrogens) are seen on the product, the Grignard reagent must have two carbon atoms. Thus, the reactant must be C2H5MgX (where X = Cl, Br). Recall that Grignard reactions involve a second step in which the alkoxide intermediate is protonated with a mild acid, as discussed in Chapter 3.4.2. The solution to this sample question is shown below in Figure 3.6.b.ii.
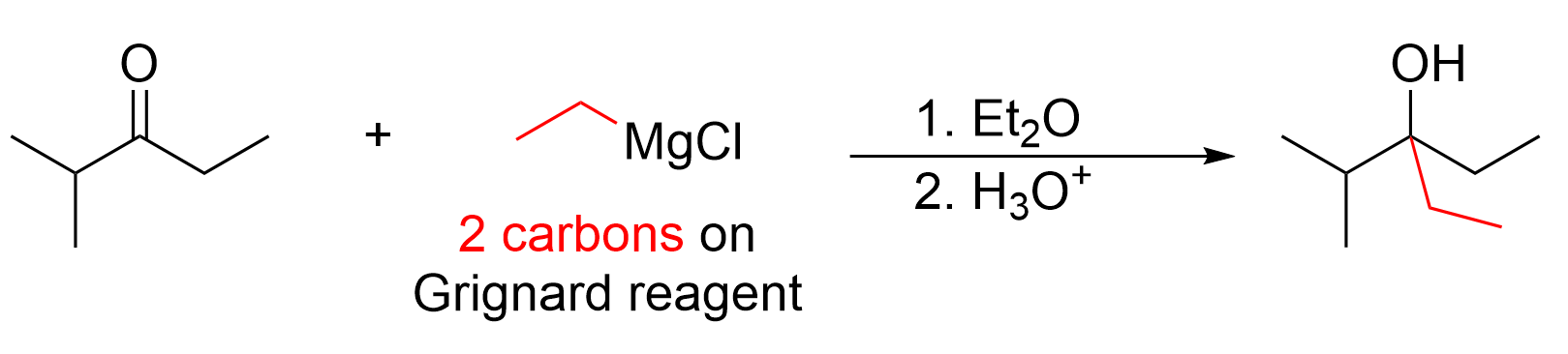
Thus, counting carbon atoms, and identifying that a new C–C bond has formed, allows us to quickly determine that a Grignard reaction has occurred.
(The full solution to this problem can be found in Chapter 5.2)
(The full solution to this problem can be found in Chapter 5.2)
(The full solution to this problem can be found in Chapter 5.2)
Identify Changes in Functional Groups
The next step is to identify the functional groups in the reactants and products, noting any changes. After you determine how the functional group is changing, you can think about what type of reaction can be used to make the change.
Let’s think about the following synthesis question below in Figure 3.6.c.i.
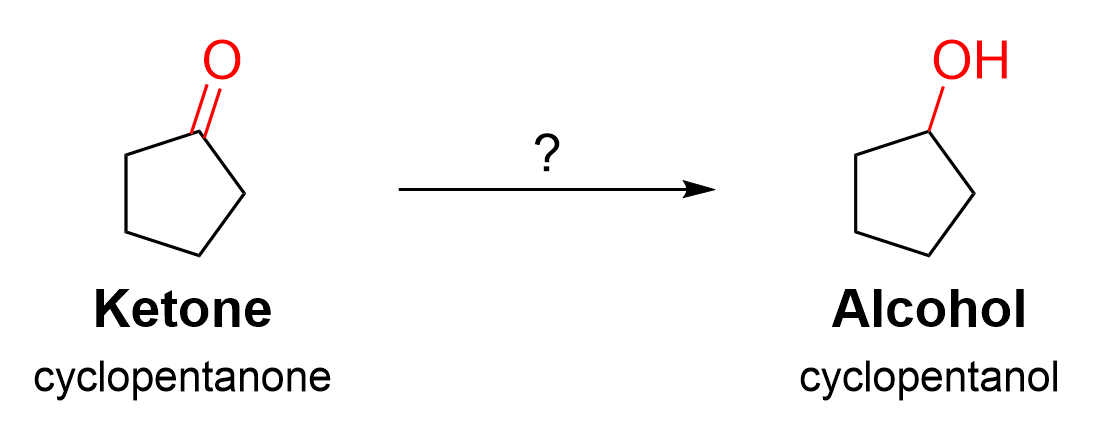
In this example, the starting material contains a ketone functional group, which is converted to a secondary alcohol. No carbon atoms have been added to expand the skeletal structure, which means a Grignard reaction did not occur. The loss of a π bond here signifies a reduction is occurring. Recall in Chapter 3.4.1. that the reduction of carbonyls is performed with sodium borohydride (NaBH4). Thus, it can be concluded that this reduction reaction occurred with NaBH4, followed by protonation of the intermediate alkoxide.
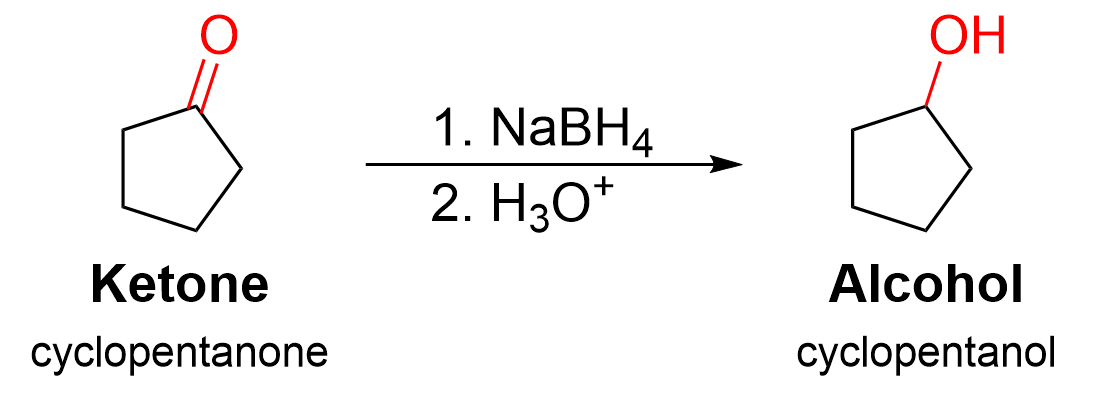
(The full solution to this problem can be found in Chapter 5.2)
(The full solution to this problem can be found in Chapter 5.2)
(The full solution to this problem can be found in Chapter 5.2)
Work Backwards
You may also be faced with a question where you must determine the starting molecule given the product and reagents. These questions require you to work backwards. If you can recognize what reaction is being performed, you can think about what the molecule looked like before the reaction took place. Figure 3.6.d.i. shows an example of this type of question.
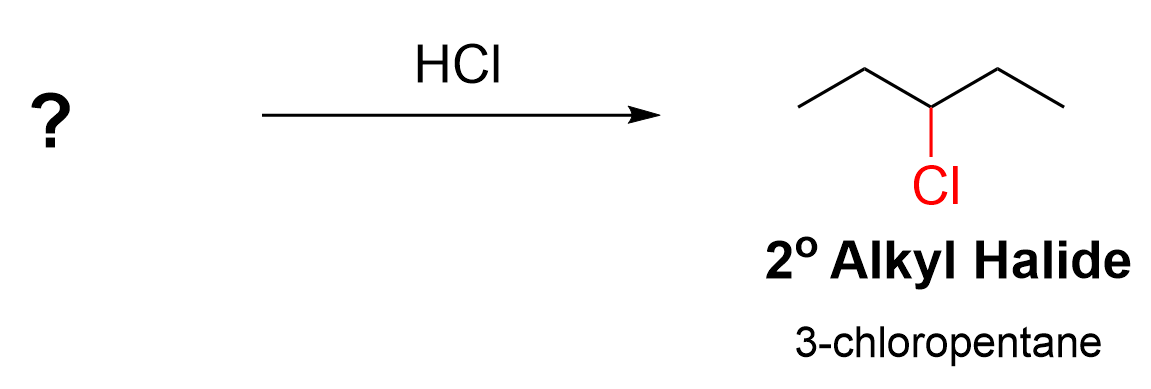
The product is an alkyl halide, 3-chloropentane. The only reagent is HCl. You should be able to recognize that hydrohalogens (H–X) are used in the hydrohalogenation of alkenes. Next, we must then determine the structure of the alkene starting material (pentene), including where the C=C double bond is located. There are two positions on a pentene where the double bond can reside: pent-1-ene and pent-2-ene. Which alkene would lead to 3-chloropentane as a product? Let’s examine all possibilities.
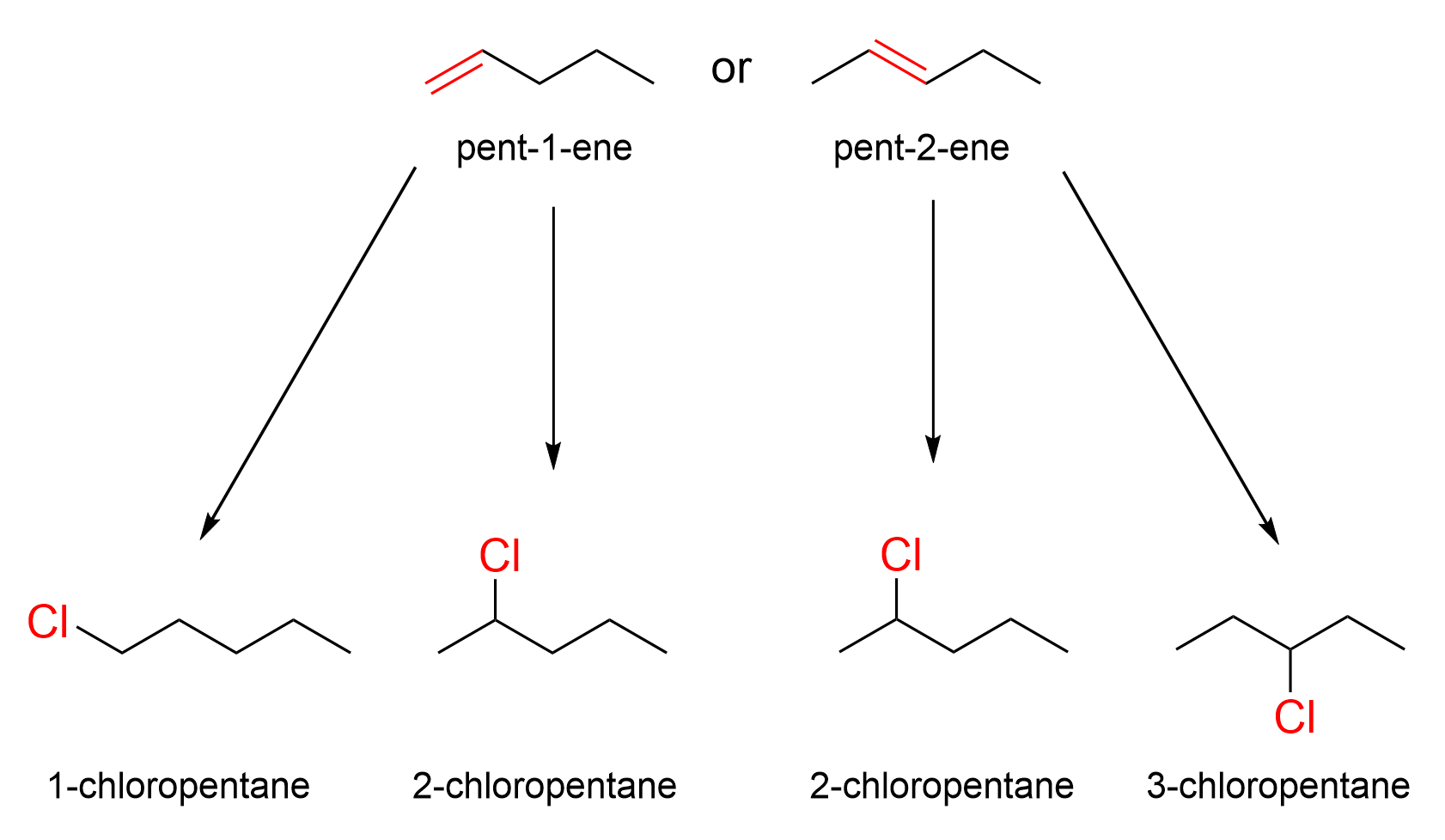
If the starting reagent was pent-1-ene, then the major and minor products are 2-chloropentane and 1-chloropentane, respectively. Neither of these compounds are the target molecule. However, when pent-2-ene is the starting molecule, one of the products is 3-chloropentane, along with 2-chloropentane. Thus, the starting material must be pent-2-ene, as seen below in Figure 3.6.d.iii.
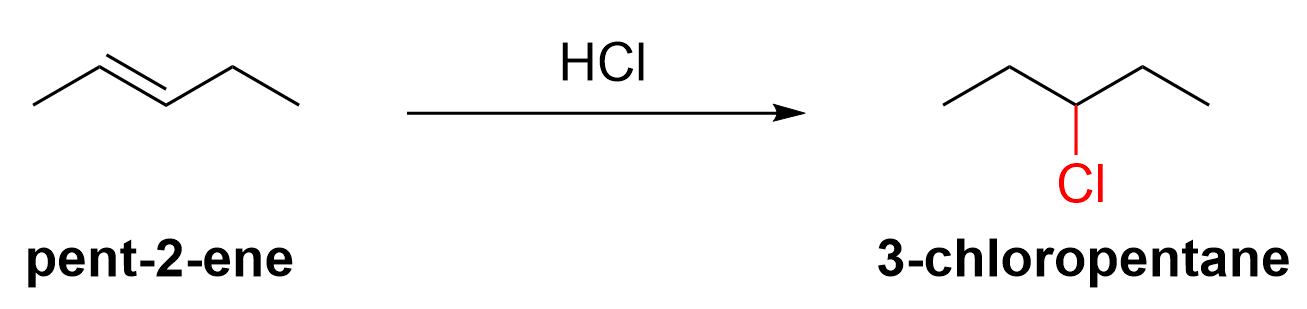
Figure 3.6.e.i. gives a more difficult example of this kind of question.
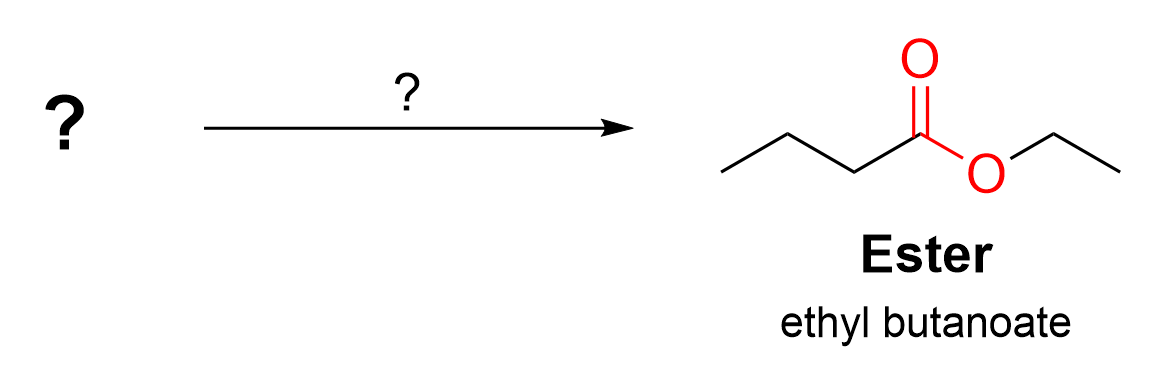
In this example, the product is an ester, and the reagents are NOT given. Thus, we must think of what possible reactions can be used to synthesize this molecule. Recall that from Chapter 3.5, there are two ways to produce an ester: (1) an SN2 reaction using a carboxylate nucleophile and an alkyl halide electrophile; or (2) an acid-catalyzed esterification reaction using a carboxylic acid and an alcohol.
First, we will examine the SN2 reaction. To determine the reagents in this substitution reaction, the ester can be split into a carboxyl component, and the alkyl chain to the right of the carboxyl group (Figure 3.6.f.ii). The carboxylate portion (butanoate) on the left includes the ester functionality as well as the carbon chain on that side of the molecule. This portion of the molecule is derived from the nucleophile, which contains an oxygen atom with a lone pair of electrons and formal negative charge. Meanwhile, the ethyl chain (right) is derived from the electrophile, an alkyl halide. As discussed in Chapter 3.1.2., the carboxylate nucleophile attacks the alkyl halide electrophile, ejecting the halide as an anionic salt.
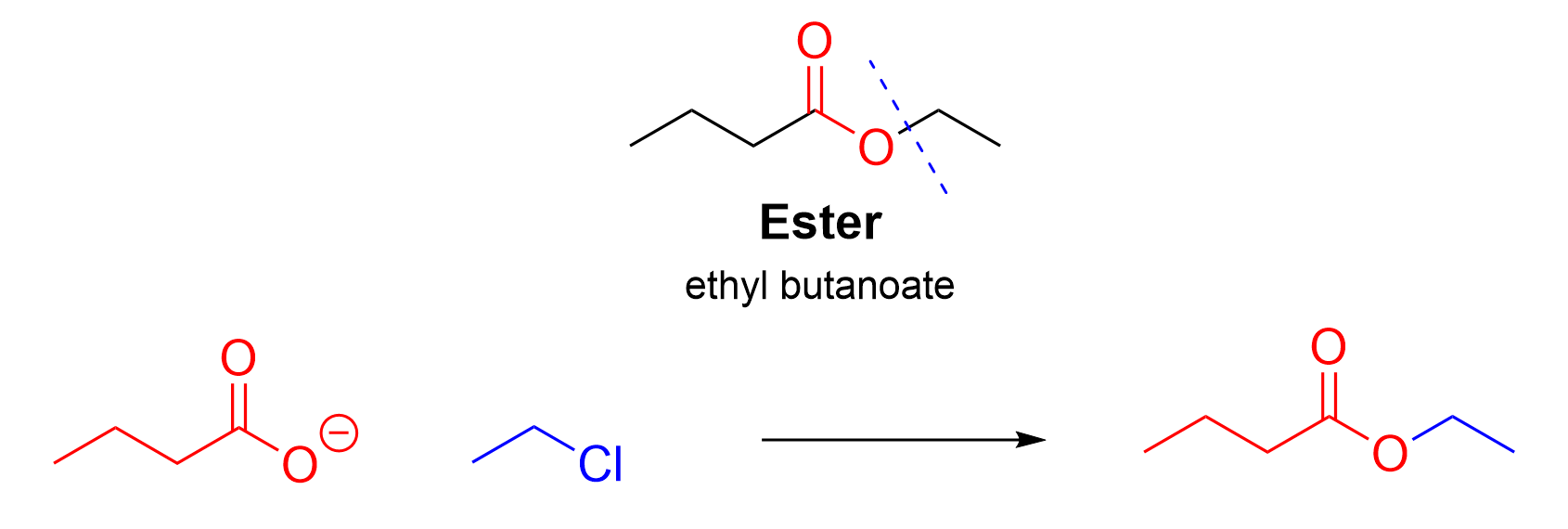
Second, we will examine the acid-catalyzed esterification reaction. The same molecule can be synthesized using a carboxylic acid and an alcohol, this time splitting the ester product in the middle of the ester group (Figure 3.6.f.iii). The portion of the molecule that contains the carbonyl group (left) is derived from the carboxylic acid, while the other portion of the molecule (right) is derived from the alcohol.
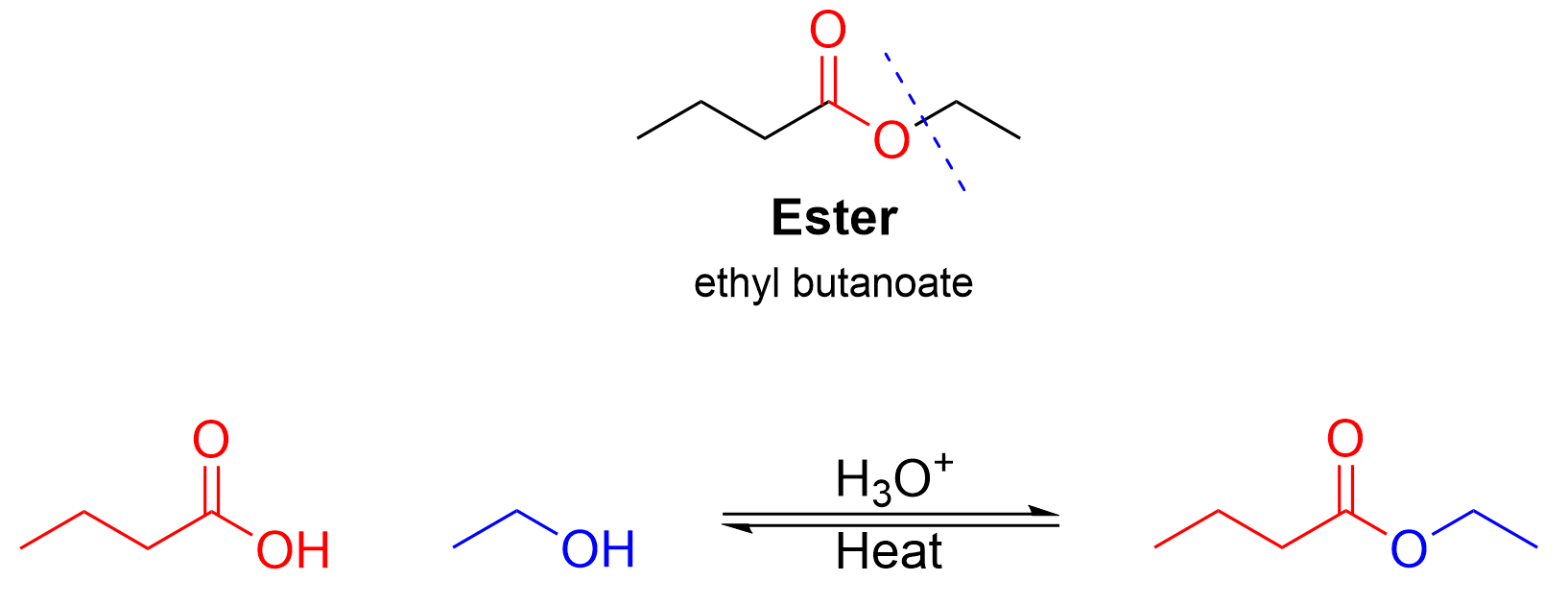
Consider a Series of Reactions
In other examples, however, the answer is not so straightforward. Sometimes there is no single reaction that can change one functional group to another, but rather a series of reactions. As an example, look at the following question below in Figure 3.6.f.i.
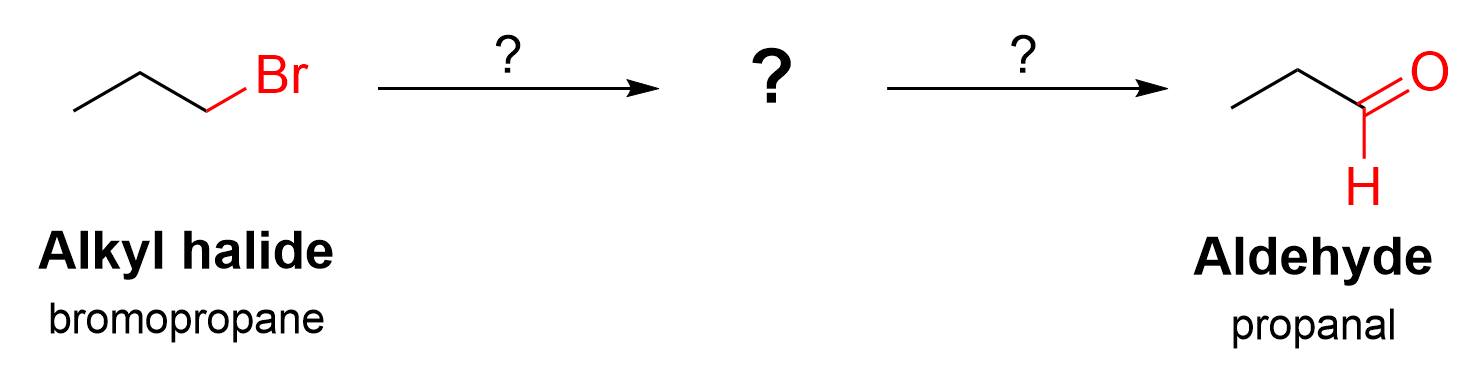
The starting substrate in this synthesis is an alkyl halide, bromopropane, and the product is an aldehyde, propanal. As indicated by two arrows, the overall transformation occurs in a series of two reactions, where the product of the first reaction is the reactant in the second reaction.
The first step is to count the number of carbon atoms, noting that no carbon atoms have been added to the structure. This means that a Grignard reaction (or esterification) did not occur.
The next step is to notice the changes in the functional groups. There is no single reaction that converts an alkyl halide to an aldehyde. Instead, we must think about what functional groups an alkyl halide can be transformed into, and what functional groups can then be converted into an aldehyde.
An alkyl halide can be transformed into a Grignard reagent (as seen in Chapter 3.4.2), or into an alcohol via a nucleophilic substitution reaction (as seen in Chapter 3.1). Meanwhile, an aldehyde can be produced by oxidizing a primary alcohol (Chapter 3.3.). Thus, the most likely product after the first reaction, which serves as the reactant in the second reaction, is the primary alcohol.
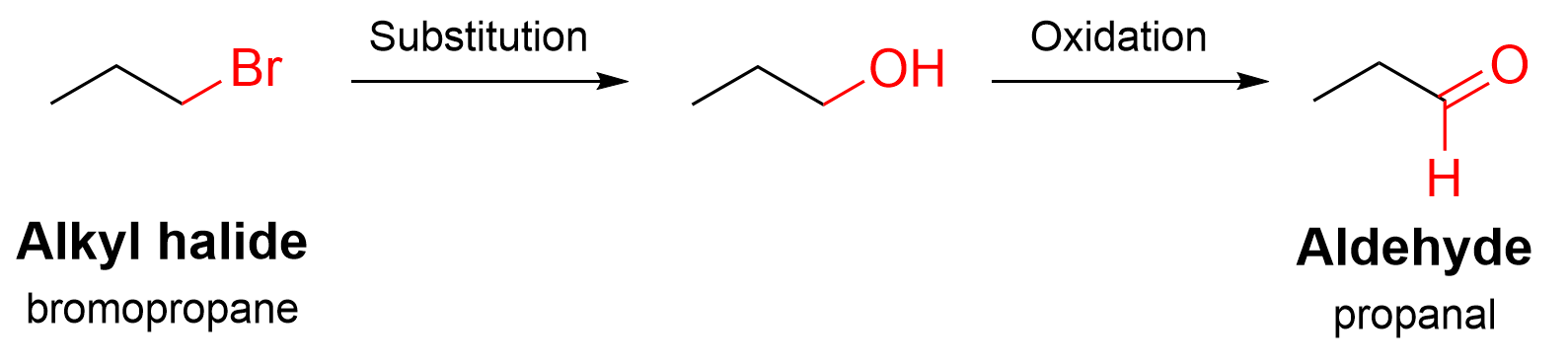
Now that we know which reactions occur and in what order, we can start working our way through to determine what reagents are used in each step. First, a substitution reaction occurs: an electron-rich nucleophile attacks the alkyl halide and replaces the Br. The nucleophile required in this case is hydroxide (OH–).
Second, oxidation of the alcohol produces an aldehyde. Recall that there are several kinds of oxidizing agents: K2Cr2O7 or K2MnO4 will oxidize a primary alcohol twice, leading to a carboxylic acid; PCC in CH2Cl2 is a more selective oxidizing agent, which allows the reaction to stop at the aldehyde. Thus, in this case, we should choose PCC in CH2Cl2.
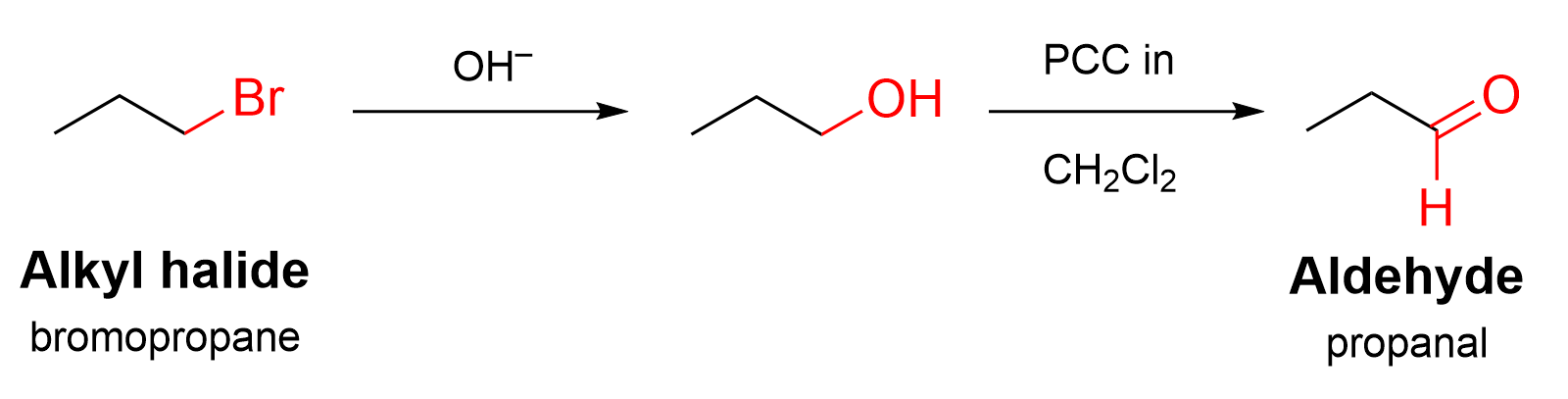
The following video includes a worked example from a previous CHEM 1AA3 test or exam that students struggled with. Try solving it on your own before looking at the solution.
Practice Questions
(The full solution to this problem can be found in Chapter 5.2)
(The full solution to this problem can be found in Chapter 5.2)
(The full solution to this problem can be found in Chapter 5.2)
(The full solution to this problem can be found in Chapter 5.2)
Key Takeaways
This may seem overwhelming, especially as you first start to work your way through a question. Rest assured that with more practice, synthesis questions will come more easily to you. To summarize, here is a list of questions to ask yourself when approaching a synthesis question.
- Count the carbons — Has the skeletal structure changed? If so, a Grignard reaction or esterification likely occurred.
- Identify changes in the functional groups — Which reaction(s) and which reagent(s) can be used to change one functional group to another?
- Work backwards — Think about what bonds could be broken or formed to reach the desired product. Now that I know this reaction was used, are there different reagents I must choose between?
- Consider a series of reactions — If the transformation cannot be accomplished using a single reaction, consider a series of reactions where the product of one reaction serves as the reactant for the next reaction.
Diversity in Chemistry: Donna Nelson
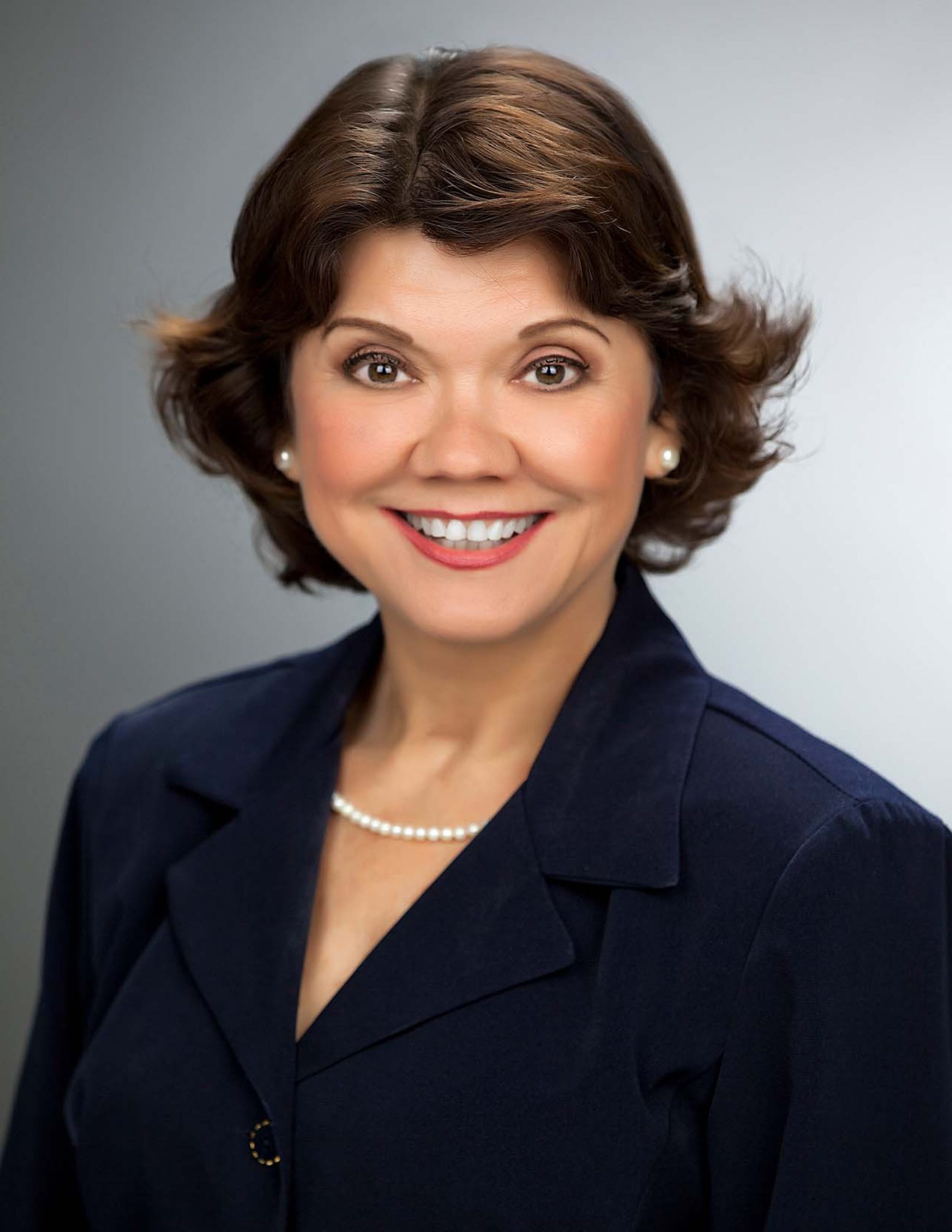
Synthesizing chemicals is not only done in science labs, but it is also done on screen as well. Recent media, such as TV showsBones andBreaking Bad, have started to incorporate science as a running theme. Despite being viewed for entertainment, shows take their scientific fundamentals very seriously. Donna Nelson, a chemistry professor of Indigenous ancestry, acted as a scientific advisor for AMC’s famous Breaking Badseries, which centers around an organic chemist. Nelson mainly assisted with organic nomenclature and stoichiometric calculations, such as helping write dialogue and providing chemical structures used as props on set. In addition to her work with Breaking Bad, she was also appointed as 2016 President of the American Chemical Society, the world’s largest scientific society with over 155,000 members. Nelson is also very passionate about education and diversity, conducting research on underrepresented groups in science faculties across American universities and aiding in improving chemistry textbooks.An interview with Donna Nelson can be found on the C&EN news site, exploring her role on set.
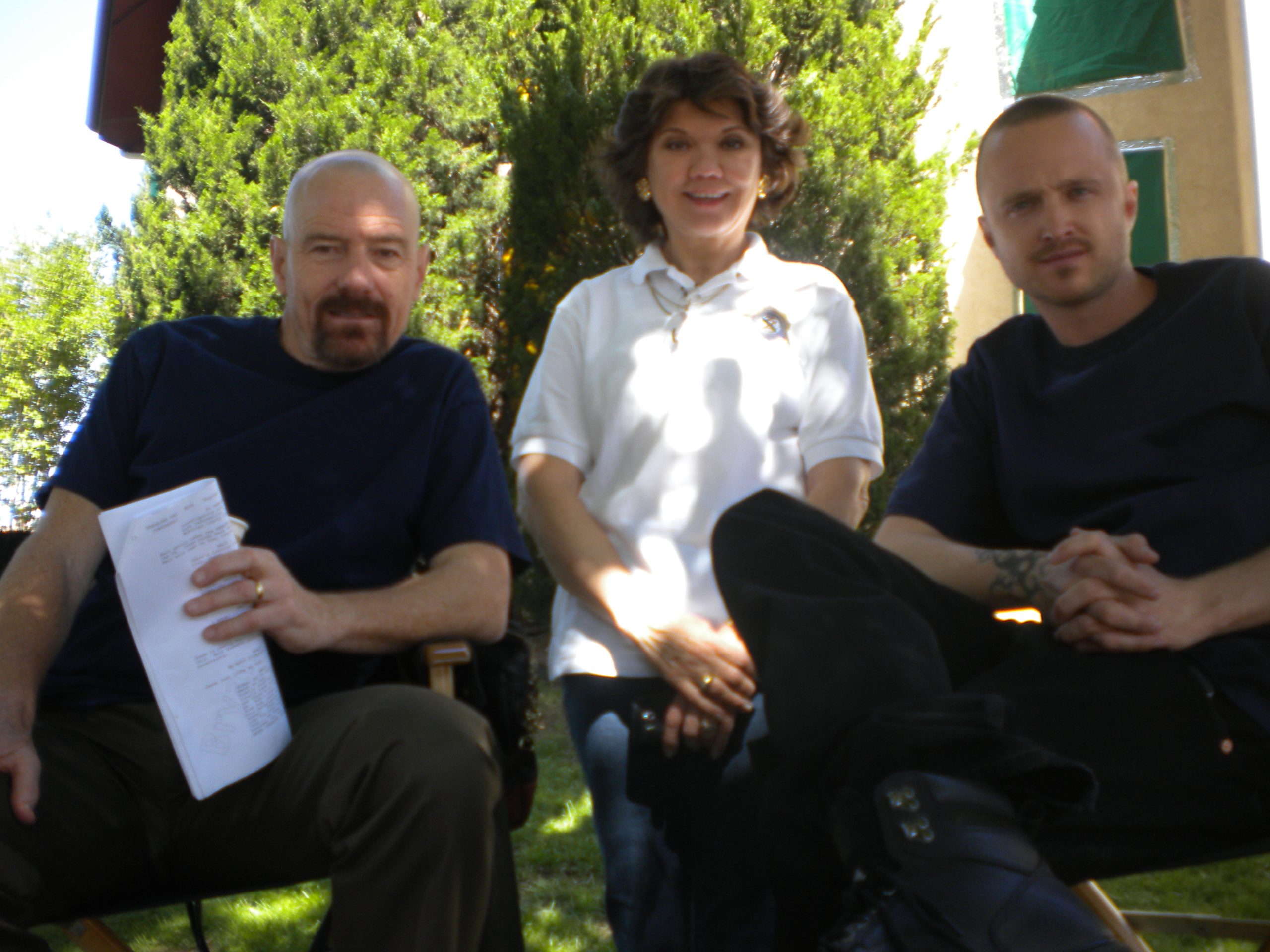
Below is an snapshot of Nelson’s academic tree, showing connections to other scientists discussed in the textbook.
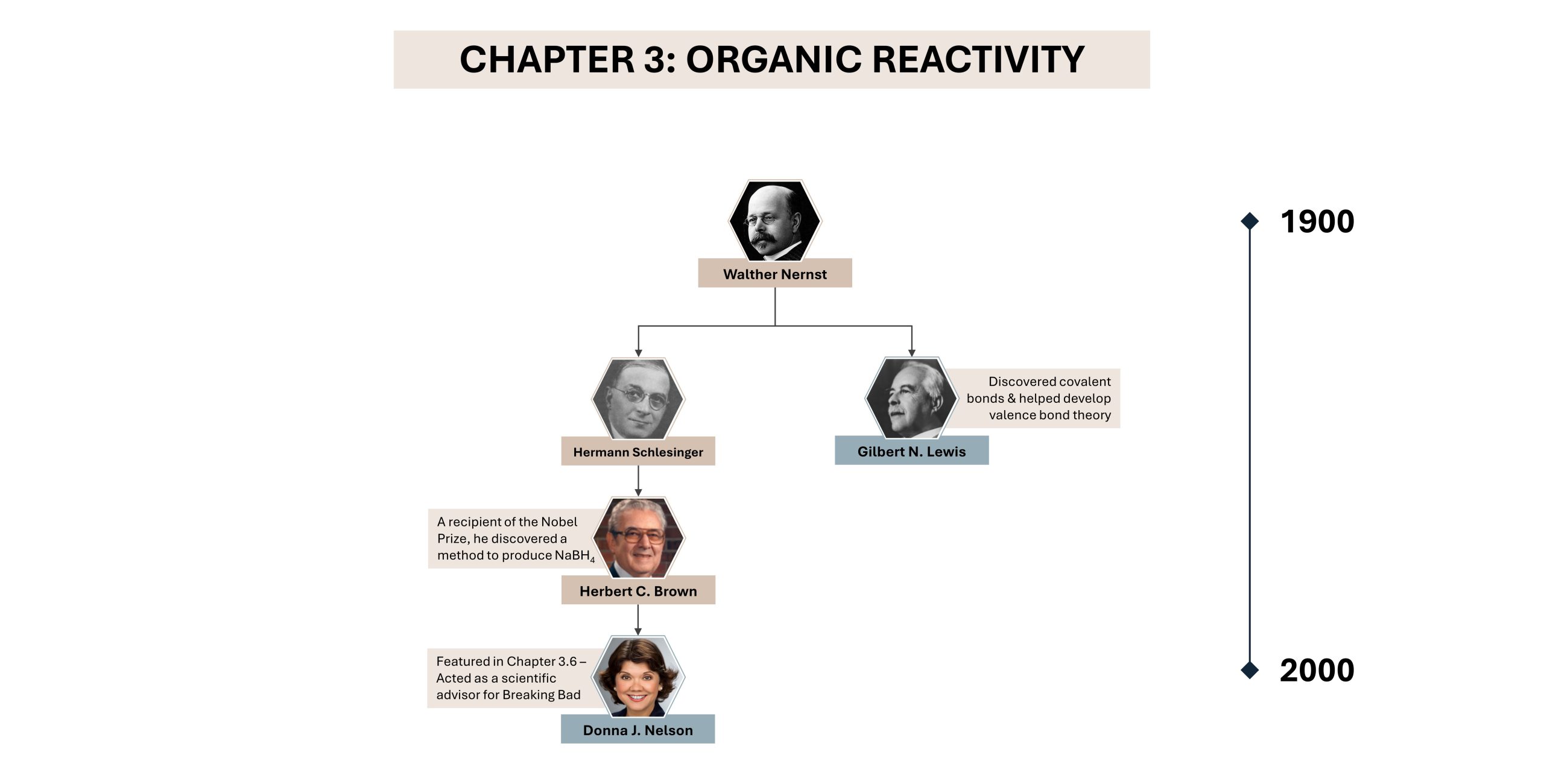