3.4.1 – Sodium Borohydride Reduction of Carbonyls
Sodium Borohydride as a Reducing Agent
In previous chapters, we learned that the term reduction can be defined as the gain of two hydrogens. We also discussed how alkenes can be reduced to alkanes through the addition of hydrogen gas, H2. Carbonyl groups, such as ketones and aldehydes, can also undergo a reduction reaction using the reagent sodium borohydride to yield alcohols. In both reduction of an alkene and reduction of an aldehyde or ketone, the overall reaction involves breaking a π bond, and addition of two new σ bonds to hydrogen atoms.
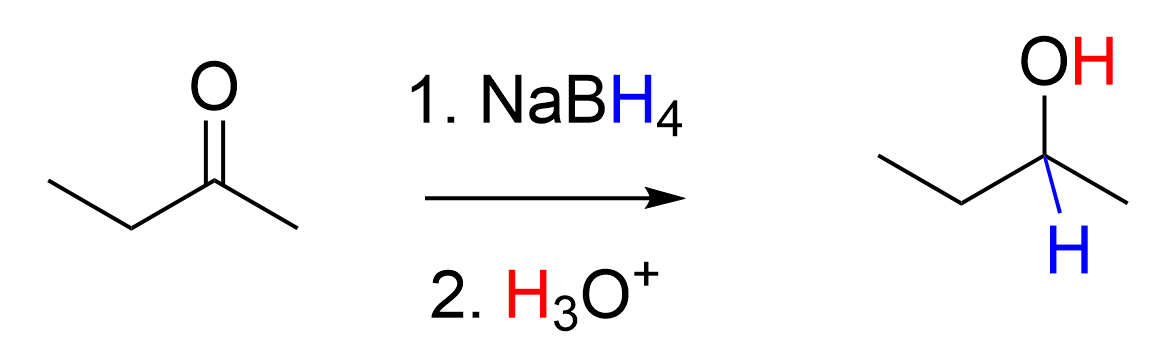
Are You Wondering? Multi-Step Processes in Synthesis
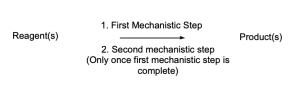
Sodium borohydride (NaBH4) is a unique reagent that acts as a source of nucleophilic hydrogen, also known as a hydride, H–. Sodium borohydride is a salt that consists of a sodium cation and a [BH4]– anion. The central boron is bonded to four hydrogens and contains no lone pairs, giving it a formal charge of 1-. However, formal charge is merely a way of keeping track of electrons, and it does not show where the electron density resides. In BH4–, the electrons are localized not on the boron center, but rather the electron density is found closer to the hydrogen atoms.
In the B–H bond, hydrogen is more electronegative than boron (electronegativities for H and B are 2.20 vs. 2.04, respectively, according to the Pauling scale). This results in the polarization of electrons towards the hydrogen, represented by the partial negative charge on hydrogen and partial positive charge on boron (Figure 3.4.1.c). This can also be rationalized by Boron’s role as a metalloid; hydrogen tends to behave as an anion when bound to metal or metalloid atoms. Due to this high electron density, the hydrogen behaves as though it has a lone pair of electrons and a formal negative charge, depicted as H:–, called hydride. Hydride acts as a nucleophile and can attack an electrophile to deliver a hydrogen atom.
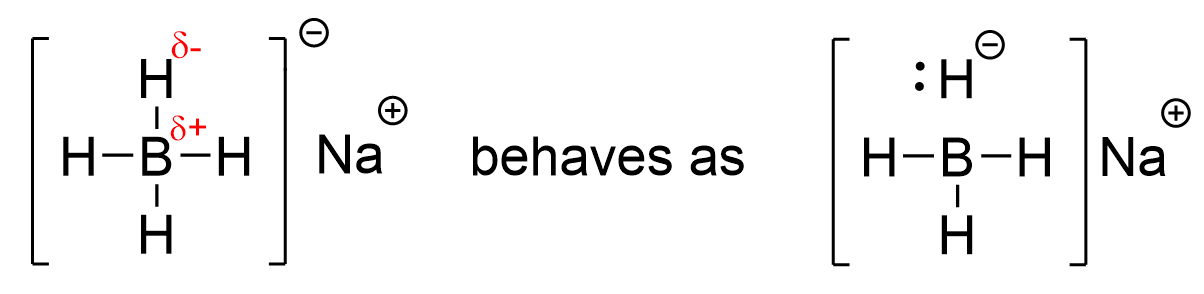
Reduction of Aldehydes and Ketones using Sodium Borohydride
Carbonyl groups, such as aldehydes and ketones, act as an electrophile due to the polar C=O bond, resulting in an electron-poor central carbon (Figure 3.4.1.d). Reaction with sodium borohydride delivers a hydride (H–) to the electron-deficient carbon, forming a new C–H bond. Subsequent treatment with an acid delivers a proton (H+) to the oxygen, forming a new O–H bond. The net result is breaking the C=O π bond, and forming two new σ bonds to hydrogen, to produce an alcohol. Because a carbon-oxygen π bond is broken and two hydrogen atoms were added in the reaction, it is called a reduction reaction, and sodium borohydride is called a reducing agent for carbonyl groups.
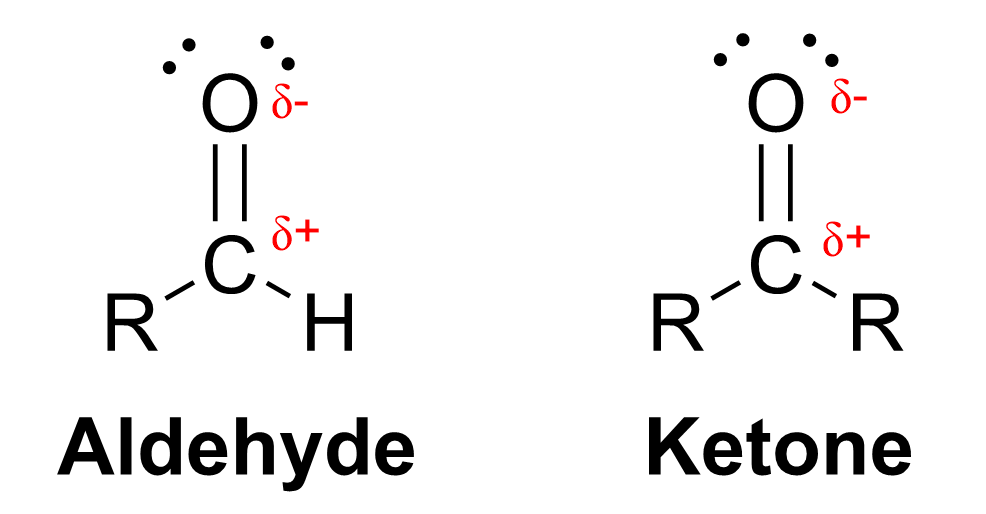
Reducing aldehydes with NaBH4 results in primary alcohols, whereas reducing ketones with NaBH4 generates secondary alcohols (Figure 3.4.1.e).
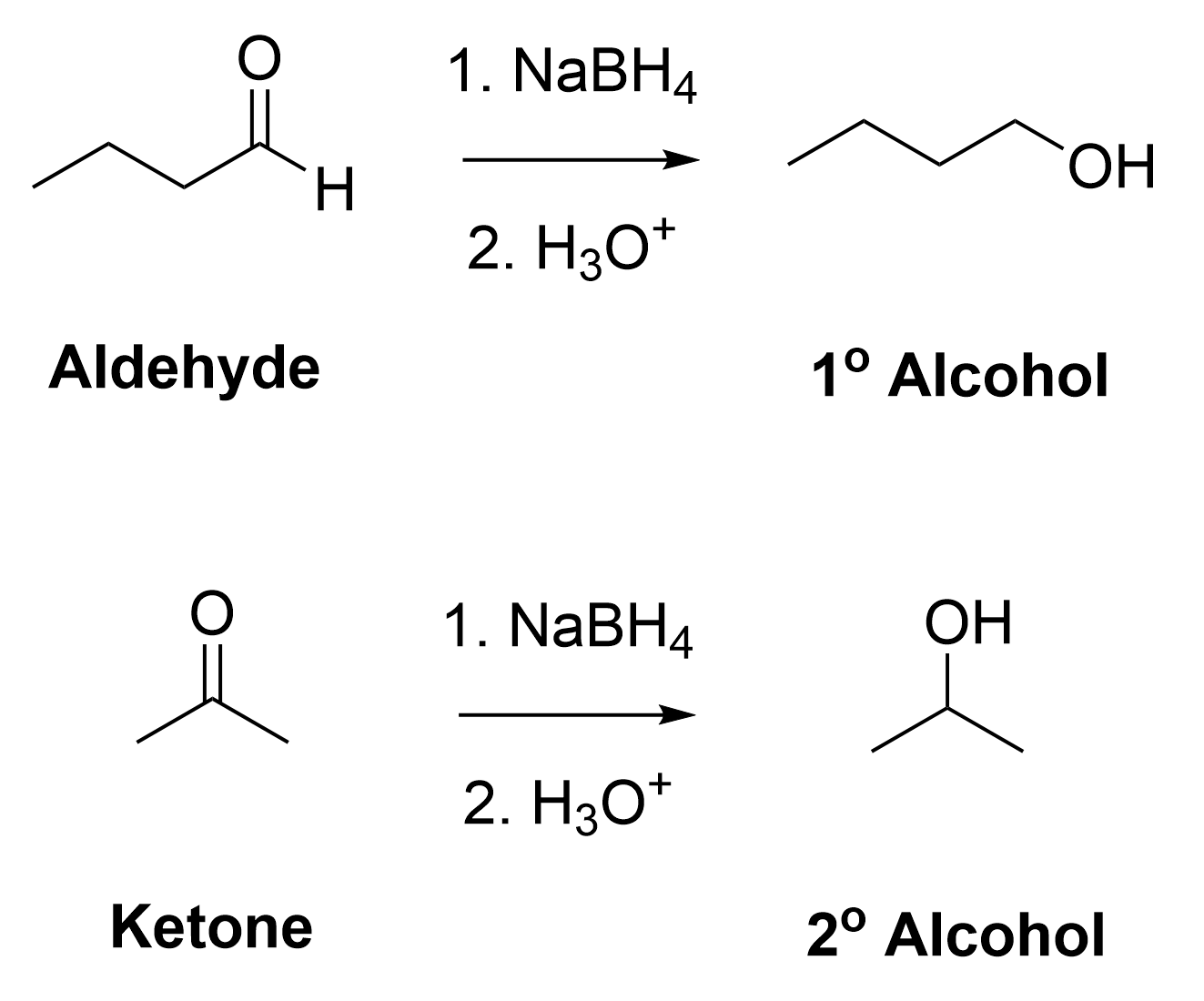
Mechanism of Carbonyl reduction using Sodium Borohydride
This reduction of carbonyl groups is a two-step process, consisting of the nucleophilic attack of hydride to the carbonyl carbon and the protonation of the alkoxide intermediate.
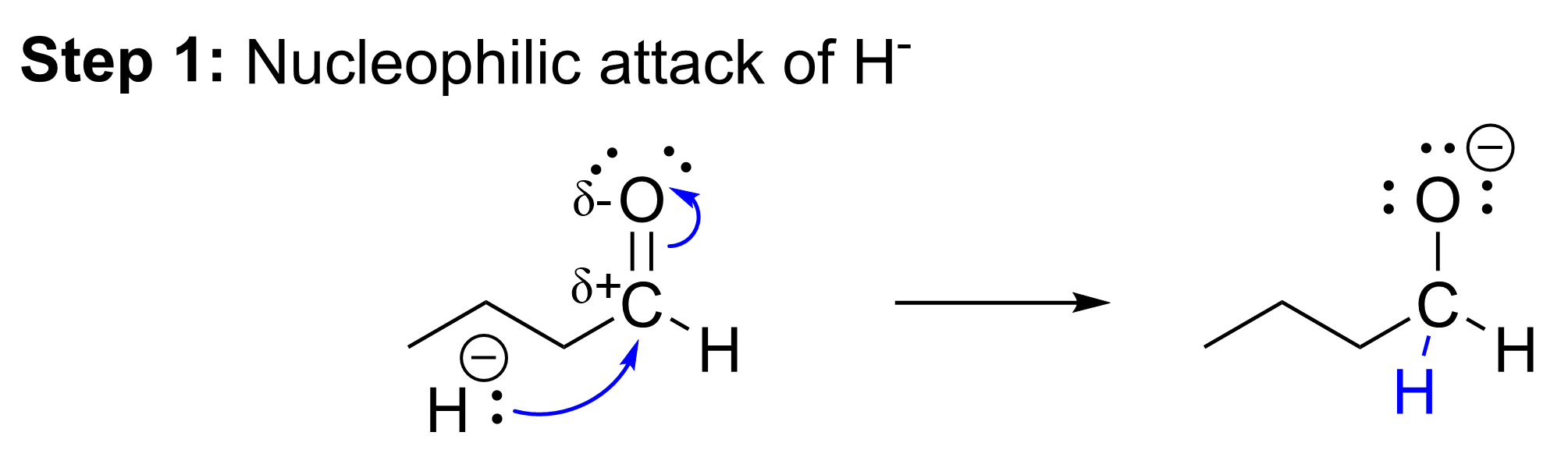
The first mechanistic step is the nucleophilic attack of the hydride from sodium borohydride at the electrophilic carbon centre of the carbonyl group. The lone pair of electrons on hydrogen attacks the carbon centre of the aldehyde to form a new covalent bond. As a new bond is forming, another bond must break to ensure that the octet rule is not violated at the carbon. The π bond preferentially breaks, with the electron pair from the π orbital of the C=O bond moving towards the electronegative oxygen. This step results in the formation of a new C–H bond, the breaking of the carbonyl π bond, and formation of an alkoxide intermediate with a formal negative charge at the oxygen.
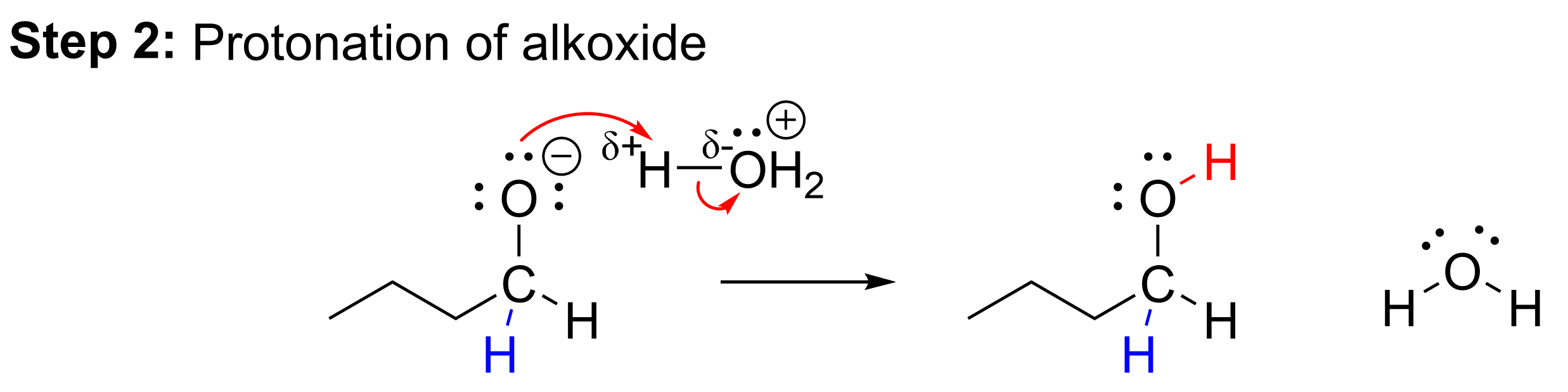
The second mechanistic step of this reaction is the protonation of the negatively charged oxygen in the alkoxide intermediate. As the previous step ended with an anionic alkoxide as the intermediate, it must be protonated to stabilize the molecule and produce a neutral alcohol. This will occur by using acid, written as H3O+. The negatively charged oxygen on the alkoxide intermediate is similar in behavior to hydroxide and is found to be highly basic, like hydroxide. It uses a lone pair of electrons to abstract the proton from the acid. This is an acid-base reaction between H3O+ (the acid) and the anionic alkoxide (the base). This second step results in our final product, a primary alcohol.
The reaction of an aldehyde with sodium borohydride produces a primary alcohol, because aldehydes have one carbon attached to the aldehyde group.
The same reaction process can be seen in the reaction between a ketone and sodium borohydride.
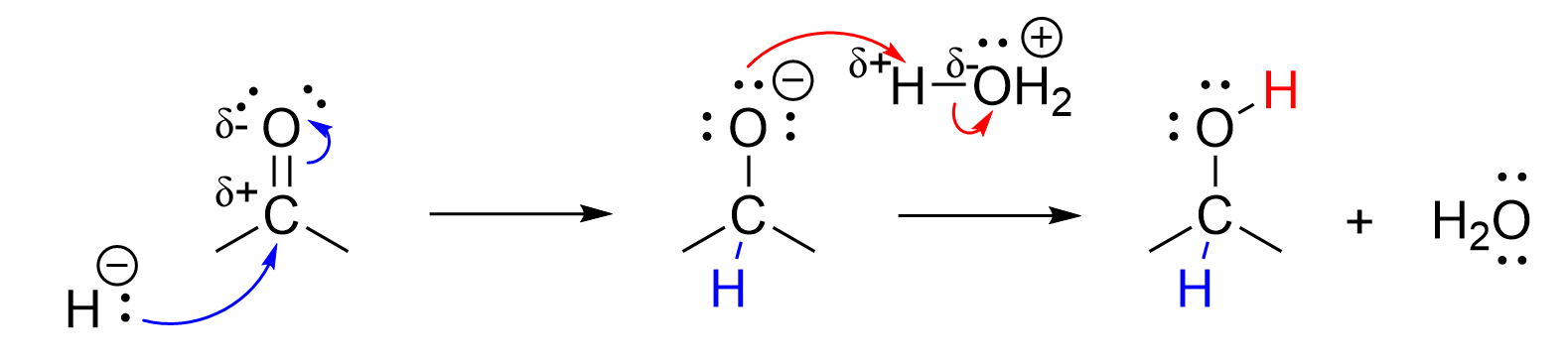
The mechanistic steps for reduction of ketones is functionally identical to the mechanistic steps involved in the reduction of aldehydes. It follows the same pattern of an initial nucleophilic attack from the hydride, followed by the protonation of the anionic oxygen. Reducing a ketone with NaBH4 produces a secondary alcohol, as ketones have two carbon-containing group attached to the central ketone group.
The two-step reaction can be summarized in the following manner, showing the intermediate as well as the starting reactant and product.
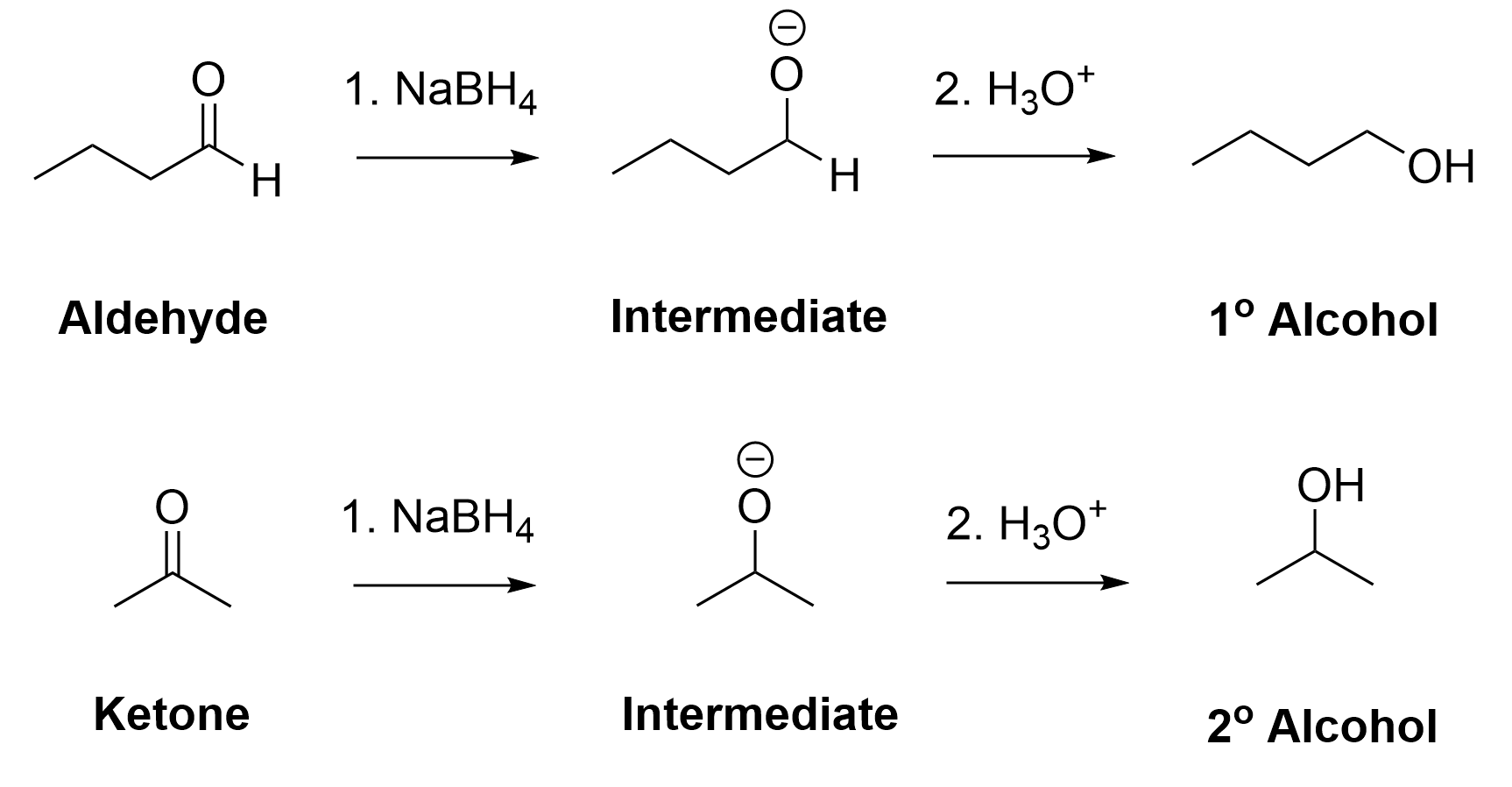
(The full solution to this problem can be found in Chapter 5.2)
Energy Profile of Sodium Borohydride reductions
As this reaction follows a two-step process, we can draw an energy profile to show the changes in energy and the intermediate formed in the reaction. The potential energy minimum in the centre of the energy profile represents the alkoxide intermediate that is synthesized in the first mechanistic step. The two maxima correspond to the two transition states where bond breaking and forming are occurring.
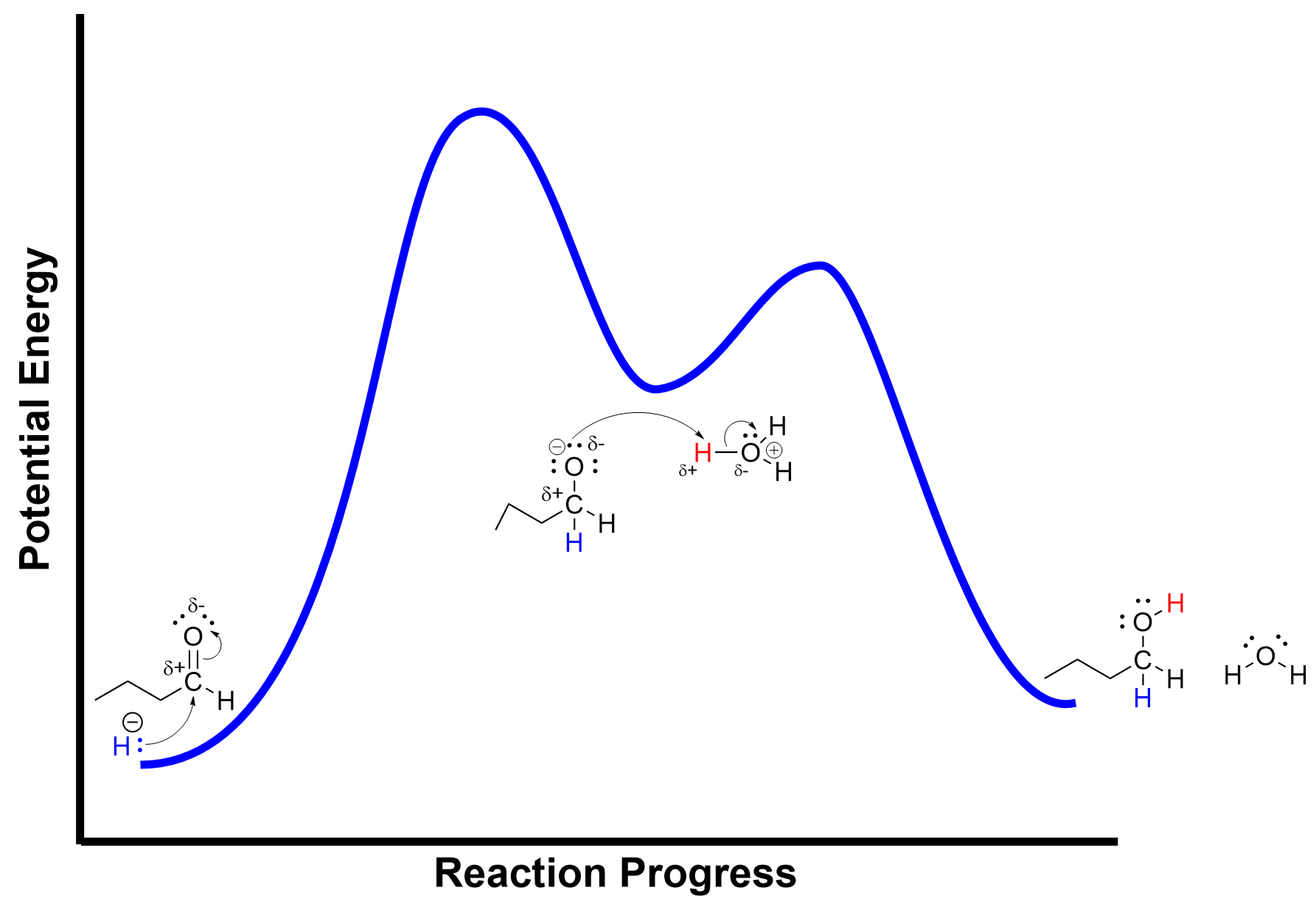
(The full solution to this problem can be found in Chapter 5.2)
Key Takeaways
- Reduction reactions of carbonyl groups, including aldehydes and ketones, can be characterized as the addition of two hydrogens and the removal of one bond to oxygen to create an alcohol product.
- This type of reduction reaction occurs in two steps when starting with an aldehyde/ketone reagent:
-
- Introduction of sodium borohydride (NaBH4), which is a hydride (H–) source. The hydride can attack the electrophilic carbonyl carbon to produce a negatively charged alkoxide intermediate. This step has the highest activation energy because of the charged intermediate.
- Introduction of an acid, which will protonate the alkoxide intermediate, will produce either a primary or secondary alcohol, depending on the starting materials.
- Overall, the first step makes a σ bond to hydrogen, and breaks a π bond to oxygen, and the second step makes a σ bond to hydrogen.
Diversity in Chemistry: Ryōji Noyori
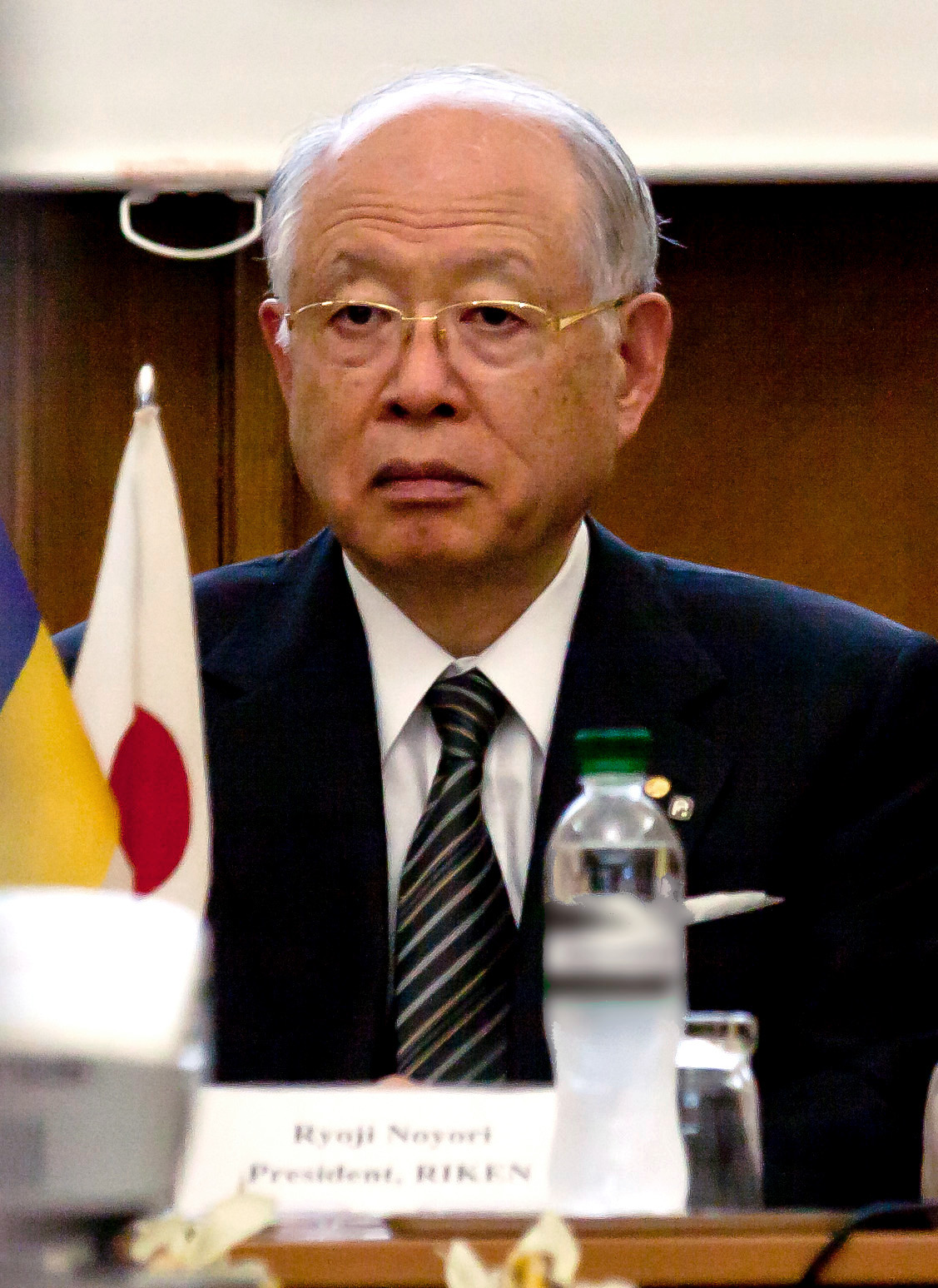
Aside from sodium borohydride reductions, there are various other methods to reduce ketone groups to alcohols. One that is commonly used in present day in the pharmaceutical industry is the Noyori Reaction, pioneered by Japanese organic chemist Ryōji Noyori. This reaction uses a ruthenium (metal)-based catalyst to reduce a ketone to an alcohol. However, the key difference between the Noyori Reaction and a simple sodium borohyride reduction is that the Noyori Reaction is much more selective. It produces a specific configuration of the final product (you will learn more about this in second year organic chemistry), which is not possible with the sodium borohydride reaction, which results in a mixture of the two configurations. For his work, Noyori was awarded one third of the 2001 Nobel Prize in Chemistry. This method is still widely used today in commercial synthesis, such as in the production of levofloxacin, a commonly used antibiotic. More information on Ryoji Noyori can be found on his Nobel Prize page.
Any feedback or comments on this chapter? You may either email chemoer@mcmaster.ca, access this MS Form, or provide a comment in the feedback box below.