3.2.2 – Hydration of Alkenes
Addition of Water to Alkenes (Acid-Catalyzed Hydration of Alkenes)
In the presence of dilute, aqueous acid, water can be added across the double bond of an alkene, producing an alcohol. This is the acid-catalyzed addition reaction of water to an alkene (also called acid-catalyzed hydration). The most common acid catalyst is a dilute aqueous solution of sulfuric acid, H2SO4. This reaction does not occur without an acid catalyst, because water is a weak acid and is incapable of protonating the double bond to push the reaction mechanism forward.
Recall that in addition reactions, one π bond and one σ bond are broken, and two σ bonds are formed. In hydration of an alkene, the C=C π bond and an O–H bond are broken, while a new C–H σ-bond and a new C–O σ bond are formed. In addition, the hybridization at carbon changes: the C=C double bond of reactants have sp2 hybridized carbon atoms, which become sp3 hybridized in the products.
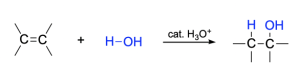
Are You Wondering? The Role of Dilute Sulfuric Acid
Sulfuric acid is a strong acid, so it’s conjugate base (HSO4–) is very weak and won’t act as a nucleophile and add to the carbocation. This will eliminate any competition between water and the acid-workup, allowing for only one product to be obtained (the addition of water). If a strong acid like HCl was used, we would see a mixture of hydration and addition products, since HCl also participates in hydrohalogenation reactions.
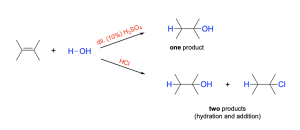
The mechanism for the acid-catalyzed hydration of alkenes is very similar to the mechanism for the addition of a hydrogen halide, HX, to alkenes, and goes through a similar carbocation intermediate. As a result, the reaction therefore also follows Markovnikov’s rule. The hydration mechanism of 1–methylcyclohexene is shown below (Figure 3.2.2.c).
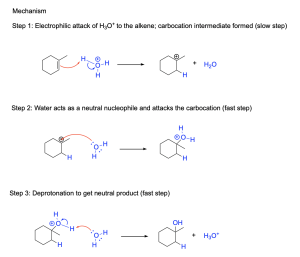
For an unsymmetric alkene as shown in Figure 3.2.2.c, the π bond gets protonated by hydronium, resulting in the formation of a C–H bond at the least substituted position (i.e. the position that has more hydrogens initially). This will create a more stable, more highly substituted carbocation at the other carbon atom from the alkene. Subsequently, water acts as a nucleophile in the second step and attacks the electrophilic carbocation intermediate, which generates an oxonium cation intermediate. Finally, water deprotonates the oxonium cation in the final step to form a neutral alcohol and regenerate the acidic hydronium ion catalyst (H3O+). Since hydronium is consumed in the first step and regenerated in the final step, it can be classified as a catalyst in the reaction mechanism. Overall, this is a three-step mechanism, with the first two steps being the addition mechanism, and the third step being a deprotonation step to yield the neutral alcohol. When compared to acid halide addition, the third step is required in this case because we are using a neutral nucleophile in step 2 (H2O) which yields a positively charged oxonium intermediate that requires deprotonation. The use of an anionic nucleophile (like a halide in acid halide addition) will result in a neutral product.
If the alkene is symmetrical (Figure 3.2.2.d), then the hydrogen could add to either carbon atom, as both would produce the same carbocation intermediate and the same final product. This is similar to hydrohalogenation of symmetrical alkenes (see Chapter 3.2.1).
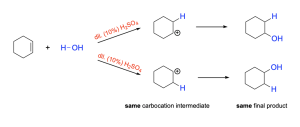
(The full solution to this problem can be found in Chapter 5.2)
The energy profile diagram for the hydration of an alkene is shown in Figure 3.2.2.e. The first step of the reaction (nucleophilic attack from the π electrons) is the rate limiting step and requires the greatest amount of energy, corresponding to the absolute maximum on the curve. This will generate a carbocation intermediate that water will nucleophilically attack to form the corresponding oxonium cation intermediate. The second step has a lower activation energy than the first step because forming a full octet at carbon is favourable and will lead to stabilization. The third step involves deprotonation of the oxonium cation intermediate which will yield the neutral alcohol.
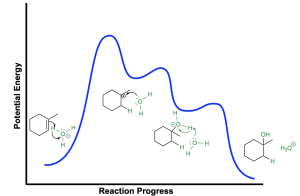
Note the similarities between hydration of an alkene (Figure 3.2.2.e) and the SN1 mechanism that uses a neutral nucleophile (such as water), discussed in Chapter 3.1.3, and shown again here (Figure 3.2.2.f). For example, both mechanisms occur in three steps, with a slow first step, and a carbocation and oxonium cation intermediate. The last two steps of both mechanisms are identical: the electrophilic carbocation is attacked by the neutral water nucleophile, generating an oxonium cation intermediate, that will be deprotonated in the final step to form a new C–OH bond. However, the two mechanisms differ in the first step of the reaction: an SN1 mechanism involves loss of a leaving group, whereas an alkene has no leaving group and is instead protonated in the first step.
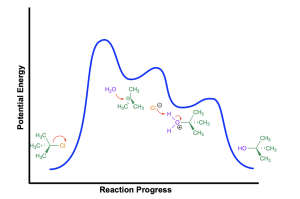
(The full solution to this problem can be found in Chapter 5.2)
The following video includes a worked example from a previous CHEM 1AA3 test or exam that students struggled with. Try solving it on your own before looking at the solution.
The following video is an interactive video that tests your knowledge on acid-catalyzed hydration – click on the video below to get started!
Key Takeaways
- Acid–catalyzed hydrogenation of alkenes is a three-step reaction, using an alkene and water as reactants, and a catalytic acid catalyst, usually H2SO4. This creates an alcohol as a product
- The three steps are as follows, where the first step is rate limiting:
- Step 1: The alkene’s π electrons nucleophilically attack the hydrogen of a hydronium, H3O+, resulting in a carbocation intermediate and water
- Step 2: The carbocation is nucleophilically attacked by water, resulting in an oxonium intermediate
- Step 3: Another water molecule deprotonates the oxonium proton, giving an alcohol and and regenerating the hydronium catalyst as products.
- For acid-catalyzed hydrogenation, it resembles hydrohalogenation such that there is a major and minor product depending on the stability of the carbocation intermediate
Diversity in Chemistry: Tehshik Yoon
Catalysts can come in many forms to enable organic reactions. These can include dilute acid, which was discussed in this chapter, or metal solids, but one of the most interesting catalysts being explored nowadays is light. Tehshik Yoon is a professor at the University of Wisconsin-Madison and is currently researching how light can be harvested in order to drive chemical reactions to reduce waste and improve efficiency. In particular, he is interested in how metal complexes can be used to absorb visible light to selectively produce desired configurations of a molecule. Yoon is also a vocal advocate for diversity, equality, and inclusion in the STEM field. As a Korean-American citizen and an openly gay man, Yoon has commented on the difficulties he had faced when first entering the STEM field and focuses on mentoring and advising efforts to spread awareness and help students himself to improve queer representation in science. More information on Yoon and his journey can be found in his interview with the C&CE journal.