3.2.1 – Hydrohalogenation of Alkenes
Hydrohalogenation of Alkenes
The addition reaction of a hydrogen halide molecule, such as HCl or HBr, to an alkene produces an alkyl halide as a product. This process is known as a Hydrohalogenation reaction.
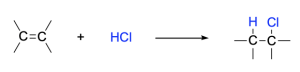
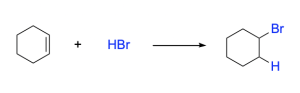
The mechanism (Figure 3.2.1.c.) for hydrohalogenation is a two-step process. In the first step, the alkene is protonated by HBr, resulting in a carbocation intermediate and a halide anion (such as Br–). In this protonation step, the π bond is broken to allow for formation of a sigma bond to the proton. This results in a carbon that loses π electron density and becomes cationic. In the second step, the halide ion formed in the first step acts as a nucleophile to attack the electrophilic carbocation intermediate. The result of the second step is that a new C–Br σ bond is formed.
Recall that in addition reactions, one π bond and one σ bond are broken, and two σ bonds are formed. In this mechanism, the first step involves breaking the H–Br σ-bond and the C=C π-bond and forming a new C–H σ-bond, while the second step involves forming a new C–Br σ bond. In addition, the hybridization at carbon changes: the C=C double bond of reactants have sp2 hybridized carbon atoms, which become sp3 hybridized in the products.
This reaction mechanism has some similarities with the SN1 mechanism discussed in Chapter 3.1.3. For example, hydrohalogenation and unimolecular nucleophilic substitution (SN1) are similar in that both mechanisms occur in two steps and involve a carbocation intermediate. In addition, the second step of both mechanisms is identical: the electrophilic carbocation is attacked by the nucleophilic halide to form a new C–X bond. SN1 reactions and hydrohalogenation differ in the first step of the reaction: an SN1 mechanism involves loss of a leaving group, whereas an alkene has no leaving group and instead is protonated in the first step.
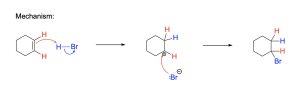
In the above examples, the alkenes are symmetrical in structure, with each alkene carbon existing in identical bonding environments. As a result. it is observed that there is no preference between which atom the halide or hydrogen atom are added to This is because the reaction pathway will proceed via an identical carbocation intermediate, so there is no preference on which side the C–H vs. C–X bond is formed, and the final product will be the same.
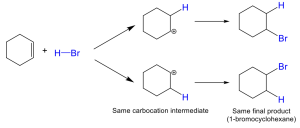
For an asymmetrical alkene, where the double bonded carbons atoms have different substituents, the placement of the H and X atoms across the π bond is critical. For example, in the following reaction (Figure 3.2.1.e.), two possible products could be produced: 1-bromo-1-methylcyclohexane and 1-bromo-2-methylcyclohexane. Experimental observations demonstrate that 1-bromo-1-methylcyclohexane is the main product for the reaction. To explain and understand the outcome of the reaction, we need to consider the mechanism of the reaction and the stability of the intermediates formed.
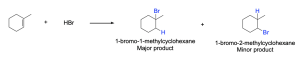
Are You Wondering? The Difference Between a Major and a Minor Product
In reactions where multiple products can form, the product that is formed in over 50% of the theoretical yield is referred to as the major product. This distribution in products is due to the energy required to form it. The major product requires less energy to form, so more will form per unit time.
In reactions where multiple products can form, the product that is formed in less than 50% of the theoretical yield is referred to as the minor product. This distribution in products is due to the energy required to form it. The minor product requires more energy to form, so less will form per unit time.
When the nucleophilic π bond attacks the electrophilic proton from H–X, a carbocation intermediate is formed. Recall carbocation stability (see Chapter 3.1.3): higher substituted carbocations are the most stable due to greater neighbouring electron density stabilization, while lowly substituted carbocations are less stable due to a lack of neighbouring electron density available to provide charge dissipation.
The energy profile diagram for the hydrohalogenation of symmetric alkenes is shown below:
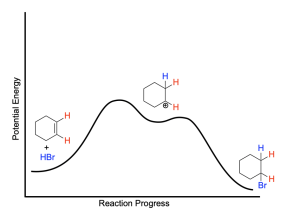
The first step of the reaction, protonation of the alkene, yields the carbocation intermediate. Since the carbocation intermediate is unstable and high in potential energy, there is a large activation barrier associated with this step resulting in it being the rate limiting step. The second step, nucleophilic attack of the carbocation by bromide, results in the final alkyl halide product.
Since the first step of the addition mechanism is rate limiting, we will focus on it to justify the differences in product formation. Just as carbocation stability is important when discussing the unimolecular nucleophilic substitution (SN1) mechanism, so too does it play a role in the hydrohalogenation mechanism (Figure 3.2.1.g). The first step of the hydrohalogenation mechanism adds a new C–H bond to one carbon atom and a formal positive charge to the other carbon atom (the carbocation). When looking at the possible carbocation intermediates formed, if the C–H bond was created on the more substituted carbon (tertiary position), then a secondary carbocation would form on the other carbon atom, leading to the 1–bromo–2–methylcyclohexane product (Figure 3.2.1.g, bottom). On the other hand, if the C–H bond was created on the less substituted carbon (secondary position), then a tertiary carbocation is formed on the other carbon atom, leading to the 1–bromo–1–methylcyclohexane product (Figure 3.2.1.g, top). Since a tertiary carbocation intermediate is more stable than a secondary carbocation intermediate, the tertiary carbocation intermediate will have a smaller activation energy barrier associated to its formation, and will form faster. As a result, more tertiary carbocation intermediate produced per unit time result in more alkyl halide product produced per unit time, leading to a higher abundance of 1-bromocyclohexane (major product).
Therefore, when performing hydrohalogenation reactions to alkenes, the electrophilic hydrogen atom is added to the least substituted carbon because it results a more substituted and more stable carbocation The halide will then attack the more substituted position in the second step of the reaction. This reaction is said to be regioselective – there is a preference to which carbon atoms the hydrogen and halide form sigma bonds too. In this case, we call this Markovnikov regioselectivity , named after Vladimir Markovnikov who studied alkene addition reactions extensively in the mid 1800s. As a result, we apply something known as Markovnikov’s rule to hydrohalogenation reactions, which states that the carbon that is already bonded to more hydrogen atoms (less highly substituted position) gets the hydrogen. This rule is also cleverly coined as the “rich get richer” rule, where a carbon atom with more hydrogen atoms bonded to it is considered “rich”.
Are You Wondering? The Meaning of the Term Regioselectivity
Regioselectivity is when a reaction mechanism favours bond formation at a particular atom over other possible atoms. For alkene additions, we observe two types of regioselectivity – Markovnikov and Anti-Markovnikov. A Markovnikov regioselective product results from a mechanism that would result from the more stable of two possible carbocation intermediates. An Anti-Markovnikov regioselective product results from a mechanism that would result from the less stable of two possible carbocation intermediates.
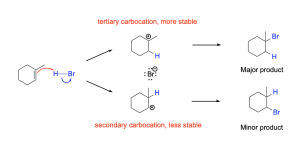
(The full solution to this problem can be found in Chapter 5.2)
Another way of naming the major and minor product of a reaction when there is Markovnikov regioselectivity is the Markovnikov and Anti-Markovnikov products.
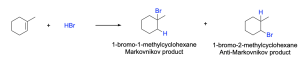
The Markovnikov product is formed through a lower energy pathway, where a more stable tertiary carbocation intermediate is formed, and the hydrogen atom is added to the carbon bonded to more hydrogens. The Anti-Markovnikov product is formed through a higher energy pathway, where a less stable secondary carbocation intermediate is formed, and the hydrogen atom is added to the carbon bonded to less hydrogens.
The energy profile diagram for the hydrohalogenation of alkenes is shown below (Figure 3.1.2.i).
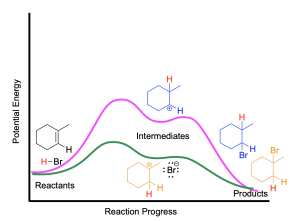
The two maxima represent the two steps of the reaction, where the first step (protonation of the alkene) is the rate-limiting step. Carbocation stability dictates which product is formed: the more highly substituted the carbocation, the more stable it will be, and the reaction pathway that involves the more stable carbocation will require less energy to overcome the activation energy barrier and proceed faster.
(The full solution to this problem can be found in Chapter 5.2)
The following video includes a worked example from a previous CHEM 1AA3 test or exam that students struggled with. Try solving it on your own before looking at the solution.
Key Takeaways
- Hydrohalogenation is a reaction using an alkene and a hydrogen halide (HX) as reactants, where the hydrogen and halogen are added to each alkene carbon
- The mechanism involves two steps, the first being rate limiting:
- Step 1: The π electrons of the double bond nucleophilically attack the proton and form a σ bond to it, creating a carbocation and a halide as intermediates
- Step 2: The halide nucleophilically attacks the carbocation, creating the product: an alkyl halide
- If the alkene is asymmetrical, then there’s two possible products: major and minor
- Because of the carbocation stability, the more substituted carbocation intermediate will be the intermediate that leads to the major product
- The major product is also called the Markovnikov product, while the anti–Markovnikov product is also the minor product
Key terms in this chapter:
Key term | Definition |
Hydrohalogenation reaction | The addition reaction of a hydrogen halide to an alkene. This produces an alkyl halide as a product. |
Any feedback or comments on this chapter? You may either email chemoer@mcmaster.ca, access this MS Form, or provide a comment in the feedback box below.
The addition reaction of a hydrogen halide to an alkene. This produces an alkyl halide as a product.