Characterization of Natural Organic Binding Media Used in Artworks through GC/MS and Py-GC/MS
Shuya Wei
Author’s Information & Abstract
Author’s Information
Shuya Wei, Institute of Cultural Heritage & History of Science and Technology University of Science and Technology Beijing; swei@ustb.edu.cn
Publishing Information
Published March 2024
Abstract
In order to preserve cultural heritage, the knowledge of the chemistry of the degradation of natural organic materials and the identification of the materials used in the objects are of main importance. The individual organic substances used as binding media or varnish in artworks were introduced. This module contributes to elucidate the chemical reactions occurring with common binding media used in objects including oils, proteinaceous materials, resins and waxes during the process of drying and ageing. The main technique used for this study is gas chromatography with mass spectrometry (GC/MS) and Pyrolysis gas chromatography with mass spectrometry (Py-GC/MS).
The basic knowledge of GC/MS, Py-GC/MS techniques and chemistry of the natural binding media are provide firstly. As case studies, the techniques of GC/MS, Py-GC/MS were applied to the investigation archaeological objects, with successful identification of organic materials involved, demonstrating how the binding media used in the cultural objects were identified, providing valuable information for understanding the objects and providing scientific support for further conservation treatment.
The techniques of GC/MS and Py-GC/MS , as well as the basic knowledge of the commonly used natural organic binding media in artworks including oils, proteinaceous materials, resins and waxes were introduced. Their analysis conditions such as sampling and sample preparation (solution and derivatisation) enabling routine GC/MS and Py-GC/MS analyses of historic binding materials have been demonstrated. The classification of the most commonly used drying oils for painting (linseed oil, walnut oil or poppy seed oil) based on the ratio of P/S is possible in the case of single binding media. However, in the presence of other binding media (such as egg, or waxes), caution must be exerted since egg and waxes also contain a considerable fraction of lipids from which palmitic and stearic acid etc. may derive.
The unambiguous identification of binders is still a challenge due to various reasons. Among these are: The deterioration of the original materials as a result of natural ageing and degradation due to environmental effects; the complexity of natural organic binding media, particularly if mixtures of different substances are used, and the small amount of sample available. The analysis of binding media mixtures will require refined methods in order to retrieve a maximum of information. So far, optimized quantitative methods still rely on individual sample preparation schemes for analysis.
Due to the simple sample preparation, high sensitivity of the Py-GC/MS with thermal assisted hydrolysis and methylation technique (THM) analysis, especially it can be used to identify multi-substance with a single analysis, which is important for samples of cultural objects (precious sample), this technique is highly recommended for binding media identification.
Introduction to Gas Chromatography and Mass Spectrometry
1. Components and Functions
GC-MS is mainly composed of gas chromatograph, mass spectrometer, mechanical pump and personal computer system (PC) (Shimadzu, 2014), which is shown in Figure 1. Gas chromatograph has a high efficiency of separating a variety of compounds and obtaining a single substance. Mass spectrometer can effectively identify an unknown compound with a high sensitivity, but it requires a single sample. Their combination enables the qualitative and quantitative analysis of complex organic materials in many fields.
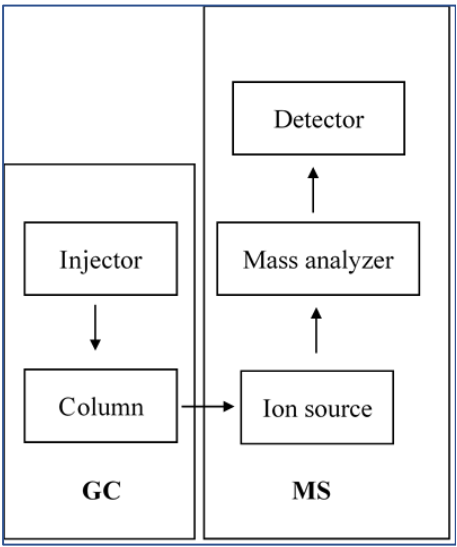
2. Gas Chromatography
Gas chromatography is a technique used to separate mixtures of gaseous chemical compounds based on differences in the compounds’ relative affinities for a solid (gas-solid chromatography) or liquid (gas-liquid chromatography) stationary phase held within a column. The main part of a gas chromatography is the column. It has a gaseous mobile phase or eluent that carries the solute or sample through the stationary phase where the separation occurs. The carrier gas (gaseous mobile phase), usually helium, nitrogen, or hydrogen, transport the sample to the stationary phase. In the terms of the intermolecular forces, an interaction occurs between the sample molecules and the stationary phase molecules. The intensity of interaction differs from strong to weak. The stronger the interaction, the longer the sample retained in the stationary phase, ensuring the successful separation of the mixture (Bruno, 2000). Figure 2 illustrate the sample (mixture) interaction with stationary phase.
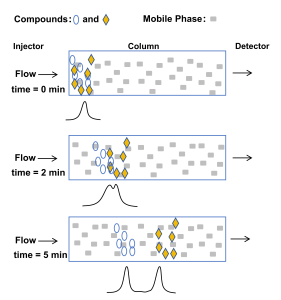
3. Mass Spectrometry
Mass spectrometry is an analytical technique that uses an instrument called a mass spectrometer to measure the mass-to-charge ratios of molecular ions. Molecules fragment within the mass spectrometer to produce a mass spectrum, which can be interpreted to determine the identity of the molecules in the sample. A mass spectrometer is commonly composed of ion source, mass analyzer and detector. Firstly, the samples molecules are ionized by ion source into gas-phase ions which are then transported to the mass analyzer in magnetic or electric field. Then the mass analyzer sorts the ions by their mass-charge ratios in the electromagnetic field. Finally, the detector converts ions into electrical signals which are shown in software in the form of spectrum (Figure 3). It should be noted that the three main parts of MS instrument work in a vacuum system. When the pressure of the instrument is high, the density of gas is high, and the probability of collision between ions is high. The collision will make the ions deviate from the normal motion orbit. Only under low pressure, the ions will have enough average free path, and there will be no collision between them. The vacuum system ensures that ions move in the normal orbit from the ion source to the detector, and ensure the good focus of the ion beam, so that high sensitivity and resolution of the MS instrument can be obtained. Mass spectrometer usually uses two-stage exhaust system. The first stage pump is usually the molecular turbine pump, which is to pump out the carrier gas from GC and remain a high vacuum state in mass spectrometer. The second stage pump is usually a mechanical pump, which can discharge the gas extracted from the first stage pump.
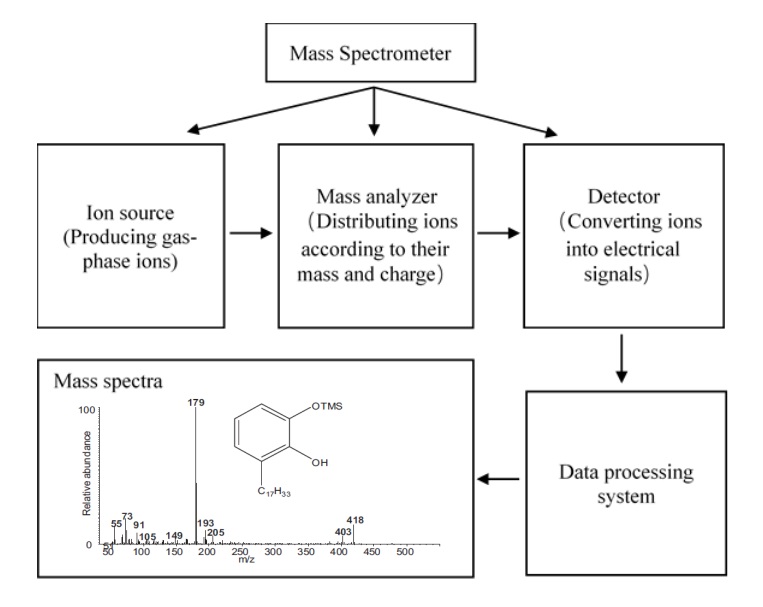
3.1 Ion Source
There are many kinds of ionization technology, including electron ionization (EI), chemical ionization (CI), electrospray ionization (ESI), matrix-assisted laser desorption/ionization (MALDI), field desorption (FD), fast atom bombardment (FAB), thermospray, desorption/ionization on silicon (DIOS), direct analysis in real time (DART), atmospheric pressure chemical ionization (ACPI), secondary ion mass spectrometry (SIMS), spark ionization and thermal ionization (TIMS) (Patel and Dutta, 2012). EI is the common ionization techniques for gases and vapors. In EI mode, the sample molecules are bombarded by electrons with certain energy (generally 70 eV), and lose their own electrons, then become molecular ions which can determine the molecular weight of the sample molecules. When the residual energy of molecular ions is greater than some chemical bonds energy, they break up and form fragment ions, which can determine the structure of the sample molecules. EI has an advantage of nonselective ionization. As long as the sample can be gasified, it can be ionized with high efficiency and sensitivity. Otherwise, the standard spectrum of EI is huge and the ratio of isotopic peaks in the spectrum reflects the distribution of natural isotopic abundances of the ions. Other ionization techniques, such as ESI and MALDI are also commonly used for their high efficiency for analyzing biomaterials (Awas and El-Aneed, 2014).
3.2 Mass Analyzer
The sector instruments of a mass analyzer include time-of-flight analyzer, quadrupole mass filter, ion traps and Fourier transform ion cyclotron resonance. There are many types of mass analyzer. Low-resolution mass spectrometers mainly include four-stage mass spectrometry and ion trap mass spectrometry, high-resolution mass spectrometers include time of flight mass spectrometry and sector field mass spectrometry, and the tandem mass spectrometer is mainly triple four-stage mass spectrometry.
3.3 Detector
The detector records the charge induced or the current produced when an ion passes by or hits a surface. In a scanning instrument, the signal produced in the detector during the course of the scan versus where the instrument is in the scan (at what m/Q) will produce a mass spectrum, a record of ions as a function of m/Q. Typically, some type of electron multiplier is used, though other detectors including Faraday cups and ion-to-photon detectors are also used. Because the number of ions leaving the mass analyzer at a particular instant is typically quite small, considerable amplification is often necessary to get a signal (Patel and Dutta, 2012).
4.0 Pyrolysis with Gas Chromatography and Mass Spectrometry (Py-GC/MS)
Pyrolysis is the transformation of a nonvolatile compound into a volatile degradation mixture by heat in the absence of oxygen. Then the volatile degradation mixture are injected into GC for separation, which subsequently are detected by a mass spectrometer, respectively. Py-GC/MS could be used to examine the composition such as polymer materials which are difficult to analyze by other techniques. Firstly, the samples are input in the pyrolizer automatically or manually, and then thermally degraded to produce smaller volatile mixtures. A flow of inlet gas, such as nitrogen or helium, flushes the mixtures into the column of gas chromatograph through the injector. Then the mixtures are separated on the column according to the differences of their polarities and the adsorption to the fixation in the column. Finally, a flow of inlet helium gas then flushes the separated mixtures into the mass spectrometer where is used to detect the mixtures (Figure 4). Py-GC/MS has become a quick, convenient and useful tool for charactering the polymer materials which are difficult to dissolve in a solvent, investigating resin deterioration, and analyzing volatile additives (Ma, Lu and Miyakoshi, 2014).
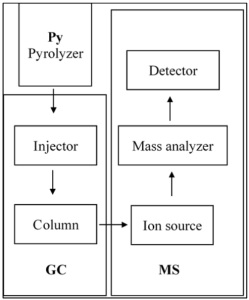
The pyrolytic process occurs when the sample is dropped into the reactor core/furnace filled with helium or nitrogen as the carrier gas. There are three main analysis modes of a pyrolyzer, including single-shot, evolved gas analysis (EGA) and double-shot. The single-shot is the simplest pyrolytic method. In the reactor core, only one heating accomplishes the thermal decomposition whose temperatures vary from 50 to 1000℃. After the thermal decomposition, the sample is cracked into smaller gas molecules and introduced into a GC instrument (Ma, Lu and Miyakoshi, 2014). It can be used to analyze all components in the sample at one time. Double-shot pyrolysis can analyze the volatile and non-volatile compounds separately. In the mode, the sample is firstly heated gradually in low temperature and emit the volatile gases which are introduced into a GC instrument. Then the remaining sample moves back to the room temperature zoom of the reaction core, waits for the reactor core to rise to high temperature and then is pyrolyzed, in similar with the single-shot pyrolysis behavior (Herrera, Matuschek and Kettrup, 2003; Quénéa, Derenne, and González-Vila, 2006).
References
Awad, Hanan, Mona M. Khamis, and Anas El-Aneed. 2015. “Mass Spectrometry, Review of the Basics: Ionization.” Applied Spectroscopy Reviews 50 (2): 158-175. https://doi.org/10.1080/05704928.2014.954046.
Bruno, Thomas J. 2000. “A Review of Capillary and Packed Column Gas Chromatographs.” Separation and Purification Methods 29 (1): 27-61. https://doi.org/10.1081/SPM-100100002.
Herrera M, Matuschek G, Kettrup A. 2003. Fast identification of polymer additives by pyrolysis-gas chromatography/mass spectrometry.” Journal of Analytical and Applied Pyrolysis, 70 (1): 35-42.
Ma, Xiao-Ming, Rong Lu, and Tetsuo Miyakoshi. 2014. “Application of Pyrolysis Gas Chromatography/Mass Spectrometry in Lacquer Research: A Review.” Polymers 6 (1): 132-144. https://doi.org/10.3390/polym6010132.
Patel, R. S., Roy M., and G. K. Dutta. 2012. “Mass Spectrometry- A review.” Veterinary World 5 (3): 185-192. DOI: 10.5455/vetworld.2012.185-192.
Quénéa K, Derenne S, González-Vila F J, et al. 2006. “Double-shot pyrolysis of the non-hydrolysable organic fraction isolated from a sandy forest soil (Landes de Gascogne, South-West France): Comparison with classical Curie point pyrolysis.” Journal of Analytical and Applied Pyrolysis, 76 (1-2): 271-279.
Shimadzu. 2014. Gas Chromatograph Mass Spectrometer GCMS-QP2010Ultra / GCMS-QP2010SE: System User’s Guide (for GCMSsolution Ver. 2.6). Kyoto: Shimadzu Corporation.
Case Study 1: The Identification of Binding Agent Used in Late Shang Dynasty Turquoise-Inlayed Bronze Objects
The study presents the results of the analysis of the binding media used in turquoise-inlay bronze artifacts in late Shang Dynasty, which were excavated in Anyang, Henan Province of China. Techniques applied include pyrolysis gas chromatography and mass spectrometry with thermal assisted hydrolysis and methylation (THM-Py-GC/MS), as well as GC/MS with derivatization reagent of MethPrep II. Marker compounds of urushi including methylated pentadecyl catechol and the oxidation products: 6-(2,3- dihydroxyphenyl) hexanoic acid; 7-(2,3-dihydroxyphenyl) heptanoic acid and 8-(2,3-dihydroxyphenyl) octanoic acid as their methylated forms were found, indicating Urushi (lacquer) was used as binding agents for the inlay. In addition, a series of fatty acids was detected with relative higher concentration of azelaic acid, which represents the presence of plant oil in the sample. Furthermore, a group of glue marker compounds and a series of long-chain fatty acids as well as a group of long-chain alcohols were detected in the sample.
Wei, Shuya, Guoding Song, and Yuling He. 2015. “The identification of binding agent used in late Shang Dynasty turquoise-inlayed bronze objects excavated in Anyang.” Journal of Archaeological Science 59: 211-218. https://doi.org/10.1016/j.jas.2015.04.021.
Case Study 2: The Study of Binding Agents Used to Inlay Turquoise onto Bronze Objects in Eastern Zhou Period
The paper presents the analysis results of the binding agents used on Turquoise-inlayed bronze artifacts in Eastern Zhou Period. The technique applied is pyrolysis-gas chromatography and mass spectrometry with thermal assisted hydrolysis and methylation (THM-Py-GC/MS). Mastic resin was identified as binding agent to inlay turquoise onto the bronze pots, based on the detection of the marker compounds of 3-oxo-olean-18-en-28-oic acid, 3-oxo-olean-12-en-28-oic acid and ursa-2, 12-dien-28-oic acid. Beeswax was determined as the binding agent used on turquoise-inlayed bronze sword according to the detection of a series of alkanes, long chain fatty acids and long chain alcohols. The results clearly demonstrate that different binding agents were used to inlay turquoise onto artifacts respectively during Eastern Zhou Period in China.
Wei, Shuya, Guoding Song, and Benxin Cui. 2016. “The identification of binding agent used to inlay turquoise onto bronze objects in Eastern Zhou Period.” Journal of Cultural Heritage 20: 671-675. https://doi.org/10.1016/j.culher.2016.01.002.
Case Study 3: Scientific Investigation of the Paint and Adhesive Materials Used in the Western Han Dynasty Polychromy Terracotta Army, Qingzhou, China
A royal tomb of early period of the Western Han dynasty (206 B.C – 8 A.D) was excavated by archaeologists in Qingzhou County, Shandong Province in 2006. Over 2000 polychromy terracotta soldiers, horses, chariots, servants etc. were unearthed from the tomb. All the terracotta figures are one quarter or one sixth as large as the livings, most of them were painted with well-designed patterns. In order to gain complete information about the materials and techniques used for the polychromy on the terracotta army, five samples from the painted areas were taken. In addition, one sample from the area to adhere one leg to the polychromy horse body was also obtained. The analytical techniques applied include XRF, FTIR, Py-GC/MS and GC/MS. Chinese purple, cinnabar, lead red and ochre were used as pigments, while animal glue was identified as binding medium and adhesive in the polychromy terracotta army in the Han Dynasty. The results definitely will provide new evidence about the materials and technologies used in Han Dynasty. Especially, the binding medium identified is different in comparison with Qin Shihuang’s terracotta army (259e210 BC).
Wei, Shuya, Qinglin Ma, and Manfred Schriner. 2012. “Scientific investigation of the paint and adhesive materials used in the Western Han dynasty polychromy terracotta army, Qingzhou, China.” Journal of Archaeological Science 39 (5): 1628-1633. https://doi.org/10.1016/j.jas.2012.01.011.
Supplemental Information: Introduction to the Natural Organic Binding Media Used in Artworks and their Analysis
1. Introduction
Paints used for decorative purpose can be traced back to as early as 40,000 years ago, when the prehistoric men decorated their caves with paintings and sketches. In ancient Egypt (5000 BC) paintings were produced with gum arabic, gelatine, egg white and beeswax as binding media, whereas the Greeks and Romans mostly used size for decoration of their walls and statues (Mills and White 1987). Egg media were common binding media of paintings before the 15th century, especially in Italy. They were gradually replaced by vegetable oils afterwards, because oils (linseed, poppy and walnut oil) made the paint more transparent. These oils are still used today for painted works of art. Apparently those organic materials play a very important role in the history of art, so it is essential to clearly identify them and to better understand their degradation mechanism to protect the cultural heritage. However, their complex chemical composition, their natural variation of contents, especially their alteration due to environmental influences during ageing as well as the limited amount of sample material usually available represent a great challenge for modern analytical chemistry.
A painting generally consists of several layers: the support (canvas, wood), the ground layer or priming (for instance gypsum and animal glue), one or more paint layers where the pigment is dispersed into an organic binder to obtain the coloured and film-forming paint capable of adhering to the ground layer, eventually an outer layer of varnish. Materials commonly used as paint media include oils (linseed oil, walnut oil, poppy seed oil), animal glues, waxes and resins. Apart from its use as a paint medium and adhesive, animal glue was mixed with gypsum for the preparation of the priming in easel paintings. The resins and waxes were used as varnish and surface coatings, as additives to paints (e.g. glazes, lacquers), as part of the pigment formulation (copper resinates) and mordant (adhesive for, e.g., gold leaf). Furthermore, resins may have been introduced by restoration procedures, both on the front as varnish or with other balsams (e.g. copaiba balsam) for regeneration purposes and at the reverse of the painting as wax/resin lining paste for repairing and strengthening of the support.
Traditional oil paints were made by grinding pigment in oil in the ratio to obtain a workable paint. Other materials could be added, such as thinner or siccatives to modify the flow characteristics and the drying properties. Once the paints applied on or in a painting, oxidative conditions are expected to result in the formation of a number of oxidation products. The changes in chemical and physical properties of oil paints can be divided into four categories: changes occur during the preparation of the paint; changes occur in the period between the application of paint and the “dry” stage; changes lead to the ageing of the paint; and changes which lead to the degradation of the paint. Numerous factors like temperature, moisture, light and reactive species in the atmosphere or materials that have been added to the painting influence the stability of a painting. Yellowing, crazing, embrittlement of varnishes, fading, (dis)coloration and destruction of the support are all unwanted defects in paintings. To better understand the degradation of binding media used in paintings is essential for conservation strategy making. The identification of materials used in works of art provides art historians, archaeologists or ethnographers with important information in order to gain a better knowledge of the techniques used by the artists.
The methods and techniques used for the identification of the materials used in paintings have been intensively studied in the past decades. Generally speaking, the m aterials used in painting can be classified into two big groups; inorganic (inorganic pigments) and organic materials (organic pigments, dyes, binding media and varnishes). To identify inorganic pigments is relatively simple due to their stability. However, the chemical characterisation of organic materials requires the development of more sophisticated identification procedures. Techniques suitable for this specific field of chemistry include thermal analysis, chromatographic and spectrometric methods. An advantage of the latter compared to chromatographic techniques is their non-destructive nature for the specimen, whereas the sample is consumed by the separation techniques. Due to the relatively good detection limits of infrared and Raman techniques, they are well suited for analysing minute sample amounts. Although spectrometric techniques have the advantage of only little sample preparation, spectra of mixtures of binding media, which have normally been used by artists, are very difficult to interpret and require preceding separation techniques for a better identification. Therefore, chromatography has proven to be one of the most useful approaches in analysing organic binding media. Methods commonly applied in this field include High Performance Liquid Chromatography (HPLC), Gas Chromatography and Mass spectrometry (GC/MS) and Pyrolysis Gas Chromatography and Mass spectrometry (Py-GC/MS) (Mills and White 1987; Van den Berg et al. 2000; Colombini et al. 1999; Asperger, Engewald, and Fabian 2001; Cappitelli, Learner, and Chiantore 2002; Lazzari and Chiantore 1999; Gimeno-Adelantado et al. 2002; Shilling 2003; Mateo-Castro et al. 2001; Scalarone, Lazzari, and Chiantore 2003).
A major problem for the identification of natural binding media is caused by drying and deterioration processes. Furthermore, the pigments used in artworks, and especially the use of mixtures of different binding media, varnishes etc. prevent the straightforward identification. For example, in the case of mastic and dammar, it is easy to distinguish between fresh samples but after extensive ageing, a reliable distinction becomes more difficult. Changes in organic materials mainly result from auto-oxidation, usually initiated by thermal, photochemical or hydrolytic reactions. Biodeterioration by moulds and bacteria represent another aspect. The binding media include oils, proteinaceous materials, resins and waxes, which are discussed individually in the following.
2. Oils as Binding Media
Oils are classified as drying oils, semi-drying oils and non-drying oils. Drying oils dry to form solid films on exposure to air; semi-drying oils form tacky films; and non-drying oils do not form highly viscous material upon exposure to air. The most important drying oils are walnut, poppy seed and linseed oil, which are normally used as binding media of paintings. Linseed oil, the mostly used drying oil, is obtained from flax, Linum usitatissimum. Linseed oil can be processed to obtain oils with different properties. In general, this was done to increase the viscosity of the oil (pre-polymerisation) and to shorten the drying time. Unfortunately, the exact conditions of the more traditional heat treatments are often not known, other than that oxygen was excluded or introduced intentionally, no certain temperature was given. The types of oil at present are known as blown-, boiled-, and stand oil. The main characteristic of the blown oil is that no driers are added to the oil. The oil is mildly heated to a temperature in the range of 40ºC to 150ºC; at the meantime blowing air into it until there is a pronounced rise in the viscosity. Boiled linseed oil refers to the oil modified by the addition of metal salts of organic acids, apart from the deliberate introduction of air. Linseed stand oil is produced by heating the linseed oil in a closed, inert vessel under the exclusion of oxygen to higher temperatures to increase its viscosity.
Once the oil has been produced it has to be purified in order to prevent drying problems later on. A number of different recipes to purify oils have been documented in the past. The effect of certain metal containing materials on the drying rate of the oil was already known at least since the 15th century, such as by using zinc, copper or bronze during bleaching.
2.1 The Identification of Oils
Different vegetable oils have distinguished properties. Chemically, vegetable oils are complex mixtures of triglycerides of long chain fatty acids. Natural drying oils are composed of triglycerides, consisting of one molecule of glycerol linked to three fatty acids (FA). The general structure of a triacylglycerol (TAG) with different fatty acids is depicted in Figure 5.
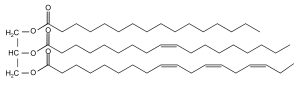
TAGs are glycerol tri-esters with three saturated/unsaturated fatty acids. A large number of possible combinations exist. An interesting result is that all TAGs of linseed oil contain at least one unsaturated fatty acid moiety. Free fatty acids are always present, with an average composition reflecting the constituents of the TAGs. These are naturally contained in the seeds or formed upon hydrolysis of the original TAG ester bonds. Apart from TAGs as the main components of the oil, other materials are present at much smaller quantities. They are found in freshly pressed oils including colouring materials like beta-carotene or chlorophyll and several sterols. Some of these can have a marked effect on the drying properties of the oil.
Oils are identified on the basis of fatty acids ratios. The ratio of palmitic acid to stearic acid (P/S) is around 1.5 for linseed oil, 3 for walnut, and 5 for poppy seed oil on a molar basis, those ratios are obtained with GC analysis after methylation of the hydrolyzed oil. The C16 and C18 fatty acids were believed to be stable marker compounds in drying oil media. The degradation products, such as suberic acid and azelaic acid can give an indication whether the oil has been pretreated or not. It should be noted, however, that other traditional paint materials e.g. egg yolk, beeswax, and animal glues contain fatty acids as well, which can lead to a wrong classification of the type of oil in case of admixtures. Schilling et al. quantified the fatty acid and glycerol content of oil paints after artificial ageing of test paints showing that the amount of C16 and C18 saturated fatty acids decreases unequally upon exposure to light and to a lesser extent upon exposure to heat. This indicates that the common practice of identification of the type of oil based merely on the P/S ratio may not be reliable. However, in a well-defined paint system, the actual evaporation of saturated fatty acids, formed upon hydrolysis of the triacylglycerols of the oil, may be much slower.
2.2 The Drying and Degradation of Oils as Binding Medium
A film of linseed oil can become touch-dry in a few days, but the drying reactions continue for many years. The drying process of linseed oil begins after a few hours of induction time, through the typical mechanism of hydrocarbon oxidation. The initial phase of oil drying consists of the unsaturated fatty acid autoxidation with the development of extensive cross-linking, together with formation of conjugated unsaturations. After an induction period, the oil absorbs large amounts of oxygen giving rise to the formation of peroxidic compounds. The oxidation will happen on the double bonds of unsaturated fatty acids such as linolic or linolenic acid, and will first lead to the formation of either hydroperoxides or cyclic peroxides. The different processes involved in autoxidation of lipid materials are very complex and depend on a variety of factors, including temperature, light, oxygen, the balance of anti- and pro-oxidative compounds, and the presence and amount of catalysts like (transition) metals etc.
3. Proteinaceous Materials as Binding Media
Whole egg and egg yolk are the most widespread media in tempera paintings. Egg yolk contains a consistent fraction of lipids. Dried whole hen egg contains about 45% protein, 41% lipids, and 2% cholesterol. Milk and casein were a valid alternative to egg tempera for artists of the past; these materials have been used in many recipes both as binders and as fixatives. Milk is an aqueous emulsion of proteins and lipids. Dried whole cow milk contains around 26% protein, 26% lipids, and 39% sugars. Casein, obtained by the acidic, enzymatic, or heating treatment of milk, is soluble in basic aqueous solutions. Ammonium caseinate has been used as a binder, mainly in mural paintings, while calcium caseinate is a strong viscous glue widely used in restoration work. Animal glues were obtained by boiling animal tissues such as bones, skin, and cartilaginous parts of animals and fish. Apart from its use as a paint medium and adhesive, animal glue was often mixed with gypsum for the preparation of the priming in easel paintings.
3.1 Structure of Protein and Amino Acids
A protein chain is formed by amino acid linked through the polypeptide bond and elimination of a water molecule, and may have somewhere in the range of 50 to 2000 amino acid residues.
3.2 Techniques for Protein Analysis
The common approach to the identification of protein media and of their degradation compounds is the use of chromatographic techniques including gas chromatography, reversed phase HPLC with pre-column derivatisation and ion exchange chromatography. Other techniques used to identify protein media including staining allow cross section examination. Fourier transform infrared spectrometry (FTIR) was used to recognize the presence of proteinaceous materials in a non-destructive manner, although it is very difficult to distinguish between egg, glue, and milk protein and the interpretation of the FTIR spectra may be extremely arduous. Differential thermal analysis (DTA) has been applied to recognize the presence of proteinaceous materials as well, but it does not allow to classify different proteins, either. HPLC with electrospray ionization time of flight mass spectrometry (ESI-TOF MS), and matrix assisted laser desorption ionization mass spectrometry (MALDI-MS) have also been used for proteinaceous paint media identification. Sometimes, binders are best identified by a combination of two or more complementary analytical techniques. The coupling of GC with MS detection allows structural information to be obtained regarding unknown or unexpected components.
Hydrolysis of the samples allows to break the polymeric network of proteins or triglycerides. The derivatisation of these components makes them more volatile in case of GC analysis. The final products of hydrolysis are a mixture of the amino acids that made up the protein. There are several ways of carrying out this reaction. The most common method to do hydrolysis is that the protein is heated with 6 M hydrochloric acid for about 24 hours at 110°C. This procedure is slow but does not require any special equipment. A newer method is microwave-assisted hydrolysis, which shortens the duration. A series of derivatisation methods have been used for proteins analysis by using GC. They include: a) transformation of amino acids into their N -trifluoroacetyl methyl esters, or into the analogous propyl or butyl esters. b) silylation of both acidic and aminic functions with formation of trimethylsilyl (TMS) derivatives or tert-butyldimethylsilyl (TBDMS) derivatives. c) derivatisation with chloroformates, such as methyl chloroformate (MCF), ethyl chlorofomate (ECF) in alcohol/water/pyridine mixtures. The reaction of chloroformate with amino acids takes place in one step, in only a few seconds and in the presence of water. It acts on both amino and carboxyl groups. The mass spectra of ethyl chloroformate derivatives undergo extensive fragmentation, which is useful for identification purposes. It is suggested to use norleucine (not occurring naturally in protein samples) as internal standard, with the aim of checking the derivatisation yield.
3.3 Drying and Degradation Processes of Proteinaceous Binding Media
During the drying process hydrated proteins of fresh binding medium loose water and their three-dimensional structures are changed leading to the liberation of functional groups and the denaturation of the molecules. Different reaction pathways will be predominant in various proteins during ageing depending on the amino acid content, initial structural variations and accessibility of chemically active side-chain groups. Sulphur-containing proteins (egg, casein) are the most labile and their cross linking and oxidation is very probable. The amino acids in the peptide chain which are most susceptible to photo-oxidation are histidine, tryptophan, tyrosine, methioine, cystine and cysteine. The relative amounts of tyrosine and lysine are often lower than those found in non-aged reference paint samples, and are similar to those found in UV aged samples, thus highlighting that photo degradation is one of the main causes of this effect
The degradation of proteinaceous materials in paint film is far from being completely understood. The possible transformations can be of physical, biological, or chemical nature. Physical changes can cause loss of adhesion and cohesion of the paint film such as swelling and shrinkage phenomena due to humidity and temperature variations. Biological changes are correlated to moisture as well, especially the growth of moulds. Chemical changes are generally due to chemical reactions induced by light, humidity, and by interaction with other chemical species. The presence of other components in the paint such as lipids or cations from pigments, may induce and catalyze the drying and degradation.
4. Resins Used in Artworks
Natural resins can be of vegetable or insect origin. Most natural resins are excretions from certain trees or shrubs. All natural resins belong to the group of terpenoids. The main components of natural resins can be classified as diterpenoids and triterpenoids, respectively. The main source of the group of diterpenoid resin is the natural order Coniferales, with several sub-families. Triterpenoid resins originate from several Angiosperm families.
The carbon skeleton of natural resins is built up from two or more of terpene units. They can be classified into monoterpenoids (a C10 skeleton, bio-synthesised from two isoprene (= C5) units, the diterpenoids (synthesized from four isoprene units, resulting in a C20 skeleton) and the tritepenoids (from six isoprene units with 30 carbon atoms). The main resins used in artworks and their sources are listed in Table 1.
Classes | Names of resins | Sources |
---|---|---|
Diterpenoids | Colophony | Pinaceae |
Sandarac | Tetraclinis articulata | |
Copal | Caesalpinioideae | |
Amber | Pinus | |
Venice turpentine | Larch (Larix deciduas) | |
Strasburg turpentine | Fir (Abies excelsa or A. pectinata) | |
Triterpenoids | Dammar | Dipterocarpaceae |
Mastic | Pistacia lentiscus L. |
Resins were used as picture varnishes in order to increase the colour saturation and gloss, and to protect the paint surface against ageing. They were also added to paint media to modify the properties of the binding media. Varnishes not only act as protective layer but also have an aesthetic function. They must be colourless, removable and their viscosity must be adequate to form homogeneous films. From the 11th century, artist applied oil varnishes by mixing natural resins like sandarac, mastic or colophony with drying oils and possibly subjected the mixture to heat during processing. The diterpene acid composition will have been greatly changed by this procedure, only the stable dehydroabietic acid would remain to indicate the original presence of a conifer, and probably a pine resin. Oils were gradually replaced in this application by solvents or spirits. Solvent varnishes made of dammar are considered the best ones due to their light stability and transparency.
Typically, varnishes become brittle and yellow during ageing, their solubility in common solvents decreases, and cracks develop on the surface. Di- and triterpenic natural resins are a mixture of different substances varying from volatile, low molecular weight- to polymeric compounds. During ageing, oxidation, polymerisation, isomerisation and cleavage reactions may happen resulting in chemical and physical changes of the varnish film. In addition, the interactions with other painting materials, especially pigments, may influence the properties of varnishes.
The compositions of fresh dammar and mastic resin have been investigated by several research groups. GC/MS is a common method for the material identification purpose. In the case of triterpenoids, a number of derivatisation methods were developed. In the past, diazomethane (CH2N2) was often used to methylate acid groups however, due to its toxicity, the use of this reagent is discontinued. This method is replaced by an alternative methylation with trimethylsilyldiazomethane (CH3)3SiCH2N2. A serious disadvantage of this method is the susceptibility of the resulting derivatives to hydrolysis by traces of water. A more often used method is based on the simultaneous formation of trimethylsilyl ethers of carboxylic and hydroxyl groups of the compounds by using bis-(trimethylsilyl) trifluoroacetamide (BSTFA).
4.1 Diterpenic Resins
The diterpenic resins commonly used as artists’ materials were colophony, Venice turpentine, copals and sandarac. Sometimes amber was also used as varnish. Sandarac and copals are fossil resins which, apart from free diterpenoids, consist of a highly polymerised fraction of polycommunic acid or communic acid copolymerised with communol. Fossil resins are hard, tough and almost insoluble in common organic solvents. Oil varnishes based on the mixture of hot linseed oil and pulverised sandarac were already used in the medieval period. From the beginning of the 15th century, the same type of varnish modified with incense or colophony, known as “vernice liquida” was used by the artists. Copals were often used by Flemish painters to prepare varnishes and oil paint media, which were appreciated because of their hardness, durability and as they were not susceptible to blooming. Most of the earliest formulations for copal oil varnishes simply included copal, oil and turpentine.
Diterpenes consist of four isoprene units. The C20 skeleton can have extra functional groups resulting in a great variety of diterpenoids which differ in the degree of unsaturation and in the number of oxygen atoms. Diterpenoids can be classified into two main groups: labdanes which are bicyclic molecules with a highly reactive unsaturated C6 side-chain; pimaranes and abietanes which are tricyclic acids (Figure 6). The abietane-type molecules, having conjugated double bonds, easily undergo oxidation reactions. The fresh resin consists almost entirely of resinous acids differing in their oxidation stability. 60% consists of abietane acids with highly reactive conjugated double bonds, 20%–25% of more stable pimarane acids and 5%–10% of acids that are more prone to oxidation (dihydroabietic and tetrahydroabietic acids). When heated, abietane-type molecules isomerise to give a mixture rich in abietic acid, whilst laevopimaric acid almost disappears. The composition of diterpenic resins and their oxidation products can be determined by GC/MS. The main compositions of Venice turpentine are same as of colophony. To differentiate Venice turpentine from colophony is by the characteristic markers of Venice turpentine, larixol and larixyl acetate. The main oxidation products normally found in turpentine and colophony are listed in the last row of Figure 6.
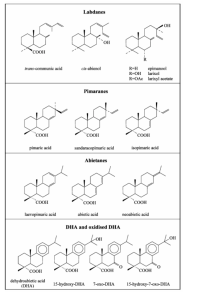
The free diterpenoids identified in copal and sandarac resins by GC/MS are sandaracopimaric acid, agathic acid and its monomethyl ester, agathalic acid and acetoxy agatholic acid. Cis-communic acid and trans-communic acid have been detected in traces in the unaged samples and they totally disappear in the aged ones. The only significant compositional difference between the two analyzed resins is due to the presence of compound methyl isopimarate in aged sandarac. It has been attributed to isopimaric acid, an isomer of sandaracopimaric acid. Oxidative drying processes have been observed by FTIR in sandarac and Manila copal too, but no oxidised derivatives of labdane diterpenoids have been identified experimentally. Methylation with trimethylsilyl (TMS)-diazomethane were successful on most diterpenoids, except for 15-hydroxy-7-oxo-dehydroabietic (DHA) acid, one of the most important markers of diterpenic resins. The best results have been obtained with trimethylsilylation using bis-(trimethylsilyl) trifluoroacetamide (BSTFA). Py–GC–MS with in situ thermally assisted hydrolysis derivatization of using hexamethyldisilazane (HMDS) was also used to analyse diterpenoid resins, while the oxidation products of colophony have been studied by HPLC/MS.
4.2 Triterpenoid Resin
Triterpenoids are usually classified according to their carbon skeleton type. The dammar resin originates from the trees of the family Dipterocarpaceae. The resin may exude naturally to some extent onto the surface of the bark, but it is generally collected by wounding the tree by means of small incisions. It consists largely of compounds of the tetracyclic dammarane skeleton series and the pentacyclic oleanane, ursane and hopane derivatives (Figure 7). The individual triterpenoid components of dammar have been reported in literature. Dammaradienone, Nor-a-amyrone, Dammaradienol, Dammarenolic acid, Oleanonic acid, Hydroxydammarenone, Oleanonic aldehyde, Ursonic acid, Ursonic aldehyde, Hydroxyhopanone. The polymer of dammar was identified as polycadinene. The oxidized compounds of dammar have been studied among others by Zumbühl et al. (Zumbühl et al. 1998) using matrix-assisted laser desorption/ionisation mass spectrometry (MALDI-MS), concluded with molecular weight information and the number of the oxygen atoms can be inserted to the molecule (e.g., oleanonic or ursonic acid) during oxidation.
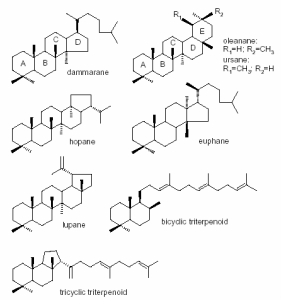
It is said that ancient Greece and Rome had great use of waxes as binding media for making paintings, statues and temple columns. Additives are often mixed with these waxy materials. Resins are used to harden and colour the material in order to improve their properties, such as using fatty materials to increase malleability and softness of waxes, using pigments and dyes to colour the materials. Beeswax is the earliest wax used in the history, but there are waxes of other origin also found to have been used in the creation of sculptures or the restoration of artworks. Waxes can be classified according to their origin as animal waxes (bees wax, wool wax, spermaceti shellac wax etc.), plant waxes (Carnarba, candelilla, Japan waxes) and mineral waxes (paraffin, Montana wax) etc. Chemically speaking, waxes are a complex mixture of n-Alkanes, long-chain alcohols and fatty acids, mono-, poly-, hydroxy-esters and triacylglycerols.
5. Waxes
It is said that ancient Greece and Rome had great use of waxes as binding media for making paintings, statues and temple columns. Additives are often mixed with these waxy materials. Resins are used to harden and colour the material in order to improve their properties, such as using fatty materials to increase malleability and softness of waxes, using pigments and dyes to colour the materials. Beeswax is the earliest wax used in the history, but there are waxes of other origin also found to have been used in the creation of sculptures or the restoration of artworks. Waxes can be classified according to their origin as animal waxes (bees wax, wool wax, spermaceti shellac wax etc.), plant waxes (Carnarba, candelilla, Japan waxes) and mineral waxes (paraffin, Montana wax) etc. Chemically speaking, waxes are a complex mixture of n-Alkanes, long-chain alcohols and fatty acids, mono-, poly-, hydroxy-esters and triacylglycerols.
5.1 Waxes from Animal Origins
Among the beehive products, beeswax was widely used until the second half of the 19th century for a large variety of purposes in the fields of technology and artworks. Beeswax is a natural product with various origins (Asiatic bees Apis dorsata, Apis floreae Apis indica, African bees Apis mellifera adansonii, and European bees Apis mellifera). Its average composition is quite constant with respect to hydrocarbons (14%), monoesters (35%), di-esters (14%), triesters (3%), hydroxymonoesters (4%), hydroxypolyesters (8%), monoacid esters (1%), acid polyesters (2%), free acids (12%), free alcohols (1%), and non-identified compounds (6%) such as flavonoids .
The composition of beeswax is often altered through time: the long-chain esters are partly hydrolyzed in palmitic acid and long-chain alcohols, and the alkanes may have sublimated either during storage in a dry and warm environment or they may have been heated in the course of the production process before use. Despite these chemical alterations, beeswax is generally characterized by its typical chromatographic pattern of n-alkanes and long-chain esters usually associated with their degradation products.
Wool wax (lanolin) is a wax obtained from the wool of sheep and is widely used in modern pharmaceutical formulations, cosmetics, and art works. It is a complex mixture of esters, di-esters and hydroxy esters of high molecular weight alcohols (aliphatic and steroidal) and high molecular weight acids. Wool wax contains also free fatty acid (FFA) and free fatty alcohol (FFAL), which are released by a slow hydrolysis mechanism.
5.2 Waxes from Plant Origins
Carnauba wax is produced by the leaves of several palm trees, such as Copernicia cerifera from the Americas, and especially in Brazil. Candelilla is produced by the leaves of various species of Euphorbia, growing in the New World, particularly in Mexico and in the South of the United States. Vegetable waxes provide very different chromatographic patterns for their total lipid extract candelilla mostly contains three n-alkanes C29, C31 (main compound) and C33. Carnauba is a more complex vegetable wax. It contains low amounts of palmitic and stearic acids, free even-numbered alcohols (C28–C34, with C32 as principal component) and a series of long-chain compounds.
5.3 Waxes from Mineral Origins
Parffine and Montan wax belong to the mineral waxes. Montan wax is a fossilized vegetable wax extracted from lignites. The chemical composition of Montan wax is very similar to some vegetable waxes (Carnauba wax) principally from the central German brown coal reserves west of the Elbe River (see Table 2).
Constituent | Average content [%] |
---|---|
Wax esters | 50-60 |
Wax acids | 20-25 |
Resin acids | 10-15 |
Polyterpenes | 3.5 |
Resin alcohols | 1.5 |
Wax alcohols | 1-1.5 |
Wax ketones | 1-1.5 |
Hydroxy wax acids | 1.0 |
Paraffins | 1.0 |
Short chain fatty acids | 0.1 |
Volatile terpenes | 0.1 |
Montan waxes of minor quality and quantity have also been mined in Australia, New Zealand, Czechoslovakia, Russia, Great Britain, China and the United States. Based on the remains of portions of plants found in the central German seams, the typical forests 30 to 40 million years ago included swamp cypresses, Sigillaria and Lepidodendron trees, and other conifers. This is thought to be the origin of the wax-containing coal.
5.4 The Analytical Techniques Used for Analysis of Waxes
Various distributions of fatty acids and alcohols from the original esters and triacylglycerols are used for distinguishing the different waxes. Gas chromatography mass spectrometry is the common technique for analytical studies of waxes. Many sample preparation alternatives have been proposed for the detailed analysis of the different chemical families of waxes. For the determination of the total content of acids in beeswax, an acid or alkaline hydrolysis of the samples followed by a methylation of the acids with diazomethane N,O-bis- (trimethylsilyl)-trifluoroacetamide (BSTFA) and N-methyl-N-tert.- butyldimethylsilyl-N- methyltrifluoroacetamide (MTBSTFA) were reported Recently ethyl choroformate (ECF) was reported as derivatization reagent to form volatile derivatives of the fatty acids, which were released from waxes. A thermally assisted hydrolysis in presence of tetramethyl ammonium hydroxide (TMAH) has also been applied in wax analysis. Using high temperature gas chromatography (HTGC) and HTGC/MS, allows to extract a maximum of information from a single analysis (Regert et al. 2003).
5.5 The Alteration of Waxes during Ageing
Although the chemical alteration of beeswax has been investigated recently by Regert et al., among all classes of organic natural binders, the degradation of waxes is currently the least investigated of all. Various alteration processes, including hydrolysis of esters and triacylglycerols, migration and crystallisation of n-alkanes and fatty acids, sublimation of n-alkanes but also oxidation and polymerisation, may occur, mostly depending on the context of preservation.
References for Supplemental Section
Asperger, Arndt, Werner Engewald, and Gerd Fabian. 2001. “Thermally assisted hydrolysis and methylation – a simple and rapid online derivatization method for the gas chromatographic analysis of natural waxes.” Journal of Analytical and Applied Pyrolysis 61: 91-109.
Bartholomé, E., E. Biekert, H. Hellmann, H. Ley, W. M. Weigert, and E. Weise, ed. 1983. Ullmanns Enzyklopädie der Technischen Chemie. Vol. 24. Weinheim: Verlag Chemie.
Cappitelli, Francesca, Tom Learner, and Oscar Chiantore. 2002. “An initial assessment of thermally assisted hydrolysis and methylation-gas chromatography/mass spectrometry for the identification of oils from dried paint films.” Journal of Analytical and Applied Pyrolysis 62 (2): 339-348.
Colombini, Maria Perla, Francesca Modugno, Marina Giacomelli, and Sandro Francesconi. 1999. “Characterisation of proteinaceous binders and drying oils in wall painting samples by gas chromatography-mass spectrometry.” Journal of Chromatography A 846 (1-2): 113-124.
Gimeno-Adelantado, J.V, R Mateo-Castro, M.T Doménech-Carbó, F Bosch-Reig, A Doménech-Carbó, J De la Cruz-Cañizares, and M.J Casas-Catalán. 2002. “Analytical study of proteinaceous binding media in works of art by gas chromatography using alkyl chloroformates as derivatising agents.” Talanta 56 (1): 71-77.
Lazzari, Massimo, and Oscar Chiantore. 1999. “Drying and oxidative degradation of linseed oil.” Polymer Degradation and Stability 65 (2): 303-313.
Mateo-Castro, R., J. V. Gimeno-Adelantado, F. Bosch-Reig, A. Doménech-Carbó, M. J. Casas-Catalán, L. Osete-Cortina, J. De la Cruz-Cañizares, and M. T. Doménech-Carbó. 2001. “Identification by GC-FID and GC-MS of amino acids, fatty and bile acids in binding media used in works of art.” Fresenius’ Journal of Analytical Chemistry 369: 642-646.
Mills, John S., and Raymond White. 1987. The Organic Chemistry of Museum Objects. London: Butterworth-Heinemann.
Regert, M., S. Colinart, L. Degrand, and O. Decavallas. 2003. “Chemical Alteration and Use of Beeswax Through Time: Accelerated Ageing Tests and Analysis of Archaeological Samples from Various Environmental Contexts.” Archaeometry 43 (4): 549-569.
Scalarone, Dominique, Massimo Lazzari, and Oscar Chiantore. 2002. “Ageing behaviour and pyrolytic characterisation of diterpenic resins used as art materials: colophony and Venice turpentine.” Journal of Analytical and Applied Pyrolysis 64 (2): 345-361.
Scalarone, Dominique, Massimo Lazzari, and Oscar Chiantore. 2003. “Ageing behaviour and analytical pyrolysis characterisation of diterpenic resins used as art materials: Manila copal and sandarac.” Journal of Analytical and Applied Pyrolysis 68-69: 115-136.
Schilling, Michael. 2003. “Workshop on Binding Media Identification in Art Objects.” LabS TECH European Infrastructure Cooperation Network. Workshop in Amsterdam, Netherlands, 24th –26th March 2003.
Van den Berg, Klaas Jan, Jaap J. Boon, Ivana Pastorova, and Leo F. M. Spetter. 2000. “Mass spectrometric methodology for the analysis of highly oxidized diterpenoid acids in Old Master paintings.” Journal of Mass Spectrometry 35 (4): 512-533.
Van der Doelen, Gisela. 1999. “Molecular studies of fresh and aged triterpenoids varnishes.” PhD thesis, University of Amsterdam.
Zumbühl, Stefan, Richard Knochenmuss, Stefan Wülfert, Frédéric Dubois, Michael J. Dale, and Renato Zenobi. 1998. “A Graphite-Assisted Laser Desorption/Ionization Study of Light-Induced Aging in Triterpene Dammar and Mastic Varnishes.” Analytical Chemistry 70 (4): 707-715.
Feedback/Errata