Chapter 1: Protein phosphorylation and its role in cell signalling
1 Protein phosphorylation and its role in cell signalling
1.1 Introduction
Proteins undergo several post-translational modifications that extend their functions and alter their interactome. Phosphorylation is one of the most common and critical regulatory mechanisms for proteins. This unit aims to provide insights to the major enzyme groups involved in protein phosphorylation and dephosphorylation.
1.2 Chapter Specific Learning Outcomes
Upon successful completion of this chapter, you will be able to:
- Define the key properties of kinases with reference to specific examples
- Define the key properties of reciprocal phosphatases with reference to specific examples
- Describe phosphorylation and dephosphorylation cycles
- Define kinomes and the role of kinases in the context of signalling
1.3 Enzymes involved in phosphorylation
1.3.1 What are protein kinases?
Kinases are a generic term for enzymes which are involved in transferring a phosphate group from one molecule (substrate) to another. In eukaryotes, conventional protein kinases introduce phosphate groups onto three key amino acids: serine (Ser), threonine (Thr) or tyrosine (Tyr).
1.3.2 List common general kinases participating in cellular signalling.
i. Tyrosine kinases
ii. Serine/Threonine kinases
The name of the kinase reflects the specific amino acids which are phosphorylated. There are also dual-specific kinases which phosphorylate Ser, Thr as well as Tyr residues. As a guiding principle, serine and threonine protein phosphorylation are associated with large changes in protein conformation, whereas tyrosine protein phosphorylation is associated with altering the cellular localization of a protein. There are approximately 518 proteins identified as kinases within the human genome (Manning et al., 2002).
1.3.3 Which functional group is targeted for phosphorylation on different amino acids?
a. Hydroxyl (OH)
b. Thiol (SH)
c. Ether (-O-)
d. Alkene(C=C)
When comparing the structures of Ser, Thr, and Tyr, note that all of these amino acids possess a hydroxyl group as part of their side-chain group (Figure 1.1). A negatively charged phosphate group can be conjugated to these amino acids, generating a phospho-Ser, phospho-Thr, or phospho-Tyr respectively.
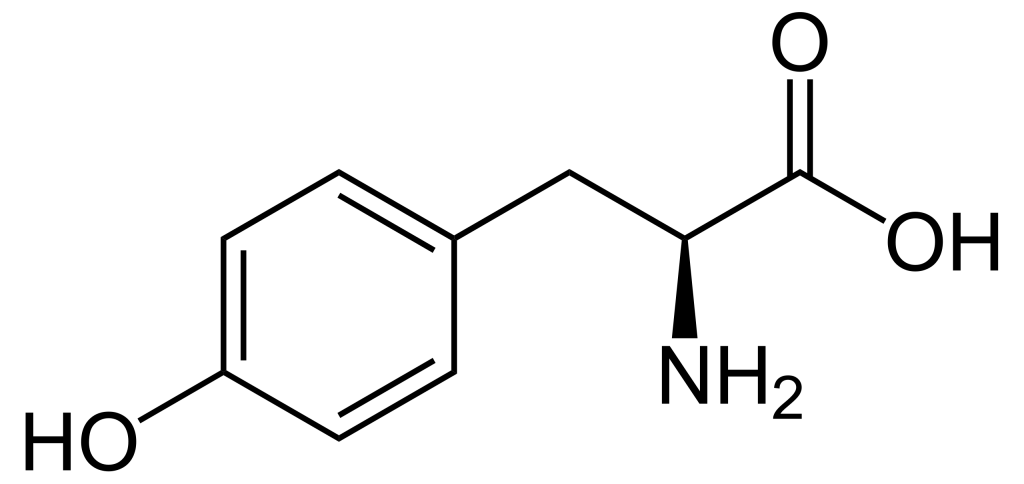
1.3.4 How does phosphorylation impact the activity of a protein?
a. Increases protein catalytic activity
b. Decreases protein catalytic activity
c. Depends on the scenario
Phosphorylation of a protein (adding a phosphate group), may increase or decrease the catalytic activity of a protein depending on the consequent structural changes that impact the protein conformation (Figure 1.2). The phosphate group introduces a divalent negative charge at the protein site (distinct from any naturally occurring amino acid) which can drastically alter the physico-chemical properties of the protein.
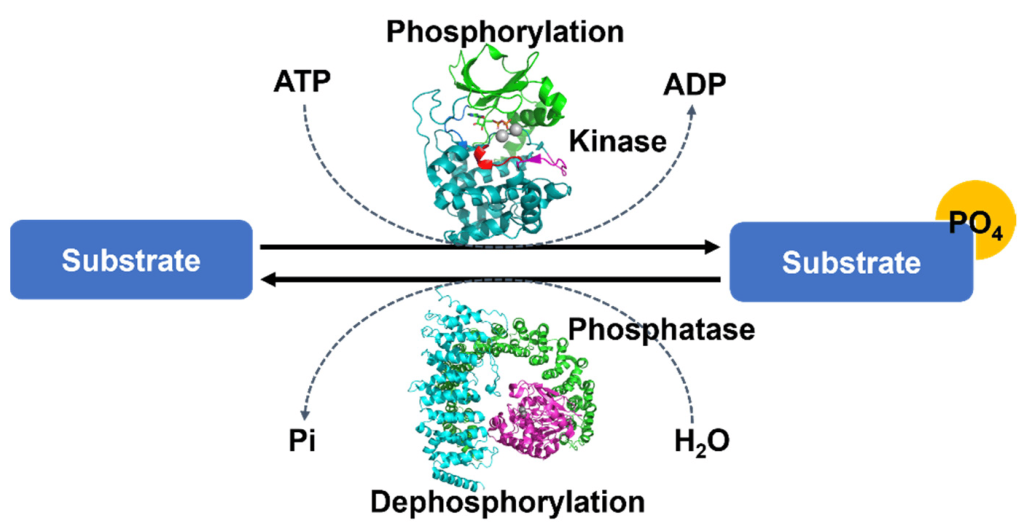
For example, the phosphorylation of a chloride ion channel protein called CFTR (cystic fibrosis transmembrane conductance regulator) occurs on a specific loop (called the R region) of the protein that normally blocks chloride ion flow through the channel (Figure 1.3). The resulting electrostatic perturbation as a result of phosphorylation of the loop enables it to engage with other regions of the protein, thereby removing it from sterically blocking pore access.
Furthermore, a subset of proteins are also prone to multi-site phosphorylation, where there are clusters of Ser or Thr available for phosphorylation. Increases in phosphorylation levels can result in a more pronounced effect. For example, the R region of CFTR contains at least ten phosphorylation sites, and progressive phosphorylation increases gating activity.
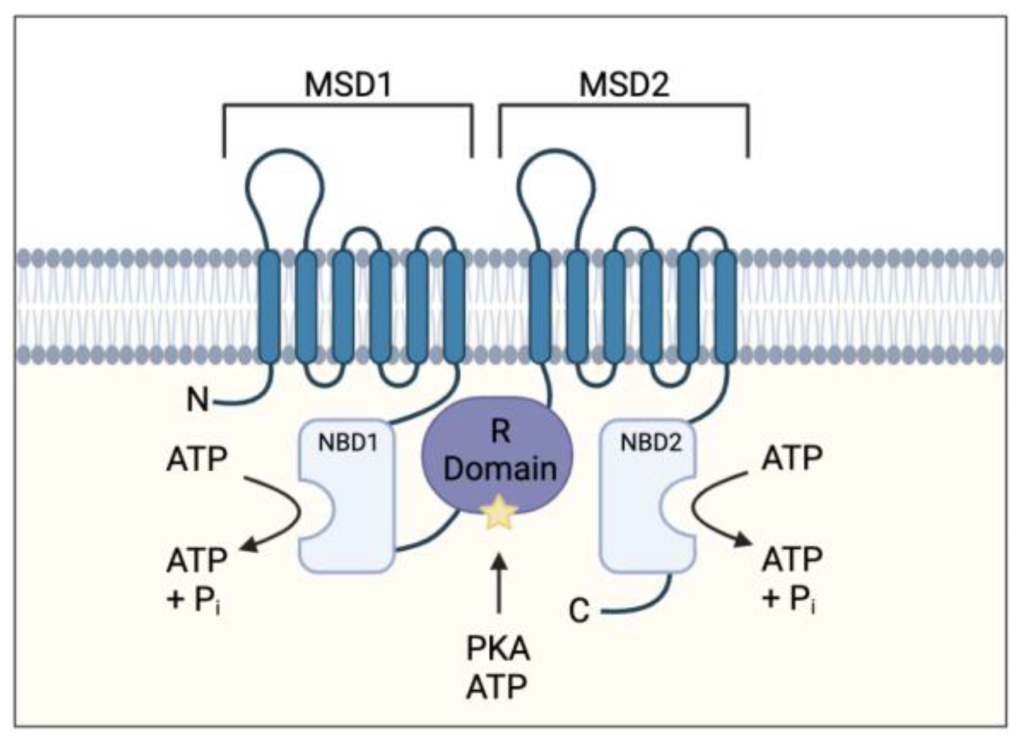
1.3.5 Briefly outline the relationship between kinases and kinomes.
There are a number of ‘-omes’ categories in biology. The -ome suffix generally refers to a subset of biomolecules from a cell or organism. This can be referred to more broadly defined subsets, such as the genome (subset of genes within a cell) or proteome (subset of proteins within a cell). There are also more niche terms such as ‘kinome’ which is encompasses all of the kinases for an organism. The human kinome is often represented in a ‘kinome tree’ which represents a phylogenetic visual representation of the evolutionary relationships between the 518 different kinases (Manning et al., 2002).
1.3.6 How does a kinase know where to phosphorylate on a protein?
Ser, Thr, and Tyr are common residues and are found in proteins. However, not all of these residues will be labelled with a phosphate group by the action of kinase. Kinases recognize a specific sequence containing a Ser/Thr/Tyr, called a ‘consensus sequence’. This peptide sequence provides a recognition motif for the kinase to bind and enables specific interactions with the kinase active site to position the appropriate Ser/Thr/Tyr into the pocket. For example, the recognition sequence of cAMP dependent protein kinase (PKA) is R-R/K-x-S-ϕ (where X is any amino acid and ϕ is a hydrophobic residue) (Kemp et al., 1977).
1.4 Enzymes involved in dephosphorylation
1.4.1 What general reaction is catalyzed by protein phosphatases?
a. Enzymes which catalyze the hydrolysis of phosphoryl groups on protein substrates
b. Enzymes which catalyze the addition of phosphoryl groups on protein substrates.
c. Enzymes which catalyze the formation of disulfide bonds.
One of the advantages of phosphorylation as a protein post-translational event, is that the phosphate group may be removed in a reversible manner through dephosphorylation steps (Figure 1.2). This provides a mechanism of transient regulation, by effectively operating as a molecular switch to turn a protein ‘on’ or ‘off’. Protein phosphatases are enzymes which remove phosphate groups present on the amino acids Ser, Thr, or Tyr resulting in a return to the original protein side chain with a hydroxyl (-OH) group. An ortho-phosphate (Pi) group is subsequently released.
1.4.2 List common general phosphatases participating in cellular signalling.
Analogous to kinases, there are two common classes of phosphatases which participate in cellular signalling pathways:
i. Ser/Thr phosphatases
ii. Tyr phosphatases
1.4.3 Define Tyrosine phosphatases.
Tyrosine phosphatases are enzymes which catalyze the removal of phosphate groups from Tyr residues of protein substrates. Tyrosine phosphatases can be further classified into either receptor-like or non-transmembrane proteins. Receptor-like tyrosine phosphatases (RPTPs) will be discussed as part of the module on enzyme-coupled receptors. There are approximately 226 protein phosphatases (Liu & Chance, 2014).
An example of a member of a non-transmembrane protein phosphatase is Src homology-2 (SH2)-domain containing protein tyrosine phosphatase-2 or SHP-2, which is expressed in multiple tissues. SHP-2 is a specific example of a cytosolic phosphatase, composed of different protein domains including two tandem SH2 domains and a catalytic phosphatase domain (Qu, 2000). SHP-2 acts by dephosphorylating multiple proteins which generally has a negative role in blocking signalling pathways.
1.4.4 Explore the SHP-2 mechanism of action in signalling networks.
In the absence of a target protein, the phosphatase domain interacts with the N-terminal SH2 domain of SHP-2. This interaction maintains the protein in a closed conformation and prevents access of substrates to the phosphatase site. Therefore, SHP-2 is unable to carry out phosphate hydrolysis reactions.
In the presence of a target (e.g. a protein with phosphorylated tyrosine), the phosphorylated target will bind SH2 domain of SHP2. This binding event triggers a conformational change, altering interactions between SH2 domain and catalytic domain. The active site becomes more accessible, enabling protein binding at the catalytic phosphatase domain and eventual removal of the phosphate group from the target protein. The products are released to reset the catalytic cycle.
1.4.5 Define Dual-specificity Phosphatases (DUSPs).
Within the tyrosine phosphatase group of proteins, there is another sub-family, referred to as DUSPs (dual-specificity phosphatases) which are phosphatases hydrolyzing phosphate groups primarily from Tyr residues. However, they also possess Ser/Thr phosphatase activity.
References
Harwood, K. H., McQuade, R. M., Jarnicki, A., & Schneider-Futschik, E. K. (2021). Anti-Inflammatory Influences of Cystic Fibrosis Transmembrane Conductance Regulator Drugs on Lung Inflammation in Cystic Fibrosis. International Journal of Molecular Sciences, 22(14). https://doi.org/10.3390/ijms22147606
Kemp, B. E., Graves, D. J., Benjamini, E., & Krebs, E. G. (1977). Role of multiple basic residues in determining the substrate specificity of cyclic AMP-dependent protein kinase. The Journal of Biological Chemistry, 252(14), 4888–4894. https://www.jbc.org/article/S0021-9258(17)40137-2/pdf
Liu, Y., & Chance, M. R. (2014). Integrating phosphoproteomics in systems biology. Computational and Structural Biotechnology Journal, 10(17), 90–97. https://doi.org/10.1016%2Fj.csbj.2014.07.003
Manning, G., Whyte, D. B., Martinez, R., Hunter, T., & Sudarsanam, S. (2002). The Protein Kinase Complement of the Human Genome. Science, 298(5600), 1912–1934. https://doi.org/10.1126/science.1075762
Qu, C. K. (2000). The SHP—2 tyrosine phosphatase:Signaling mechanisms and biological functions. Cell Research, 10(4), 279–288. https://doi.org/10.1038/sj.cr.7290055
Seok, S.-H. (2021). Structural Insights into Protein Regulation by Phosphorylation and Substrate Recognition of Protein Kinases/Phosphatases. Life, 11(9). https://doi.org/10.3390/life11090957