Chapter 4: Nuclear Receptors in Signalling Pathways
4.1 Introduction
Nuclear receptors are transcription factors which play a role in gene expression influencing processes such as metabolism, proliferation, electrolyte balance, reproduction and inflammation (Lavery & McEwan, 2005; Mazaira GI, 2018). Due to their broad implication in a number of physiological activities, nuclear receptors are also targets for therapeutics. This unit aims to describe the mechanism of action of nuclear receptors in signalling pathways. The structural and functional aspects of nuclear receptors will be described.
4.2 Chapter Specific Learning Outcomes
Upon successful completion of this chapter, you will be able to:
- Identify features of ligands for nuclear receptors
- Describe the domain structure of nuclear receptors
- Define orphan nuclear receptors
- Describe the impact of phosphorylation on nuclear receptors
- Compare and contrast nuclear receptors from other receptor proteins
4.3 Signalling molecules
4.3.1 What are some common structural features of ligands that target nuclear receptors?
a. Possess aromatic groups
b. Predominantly hydrophilic in composition
c. Predominantly hydrophobic in composition
d. Options a and b
e. Options a and c
Ligands that target nuclear receptors are distinct from ligands that target the (previously discussed) enzyme-coupled receptors or G-protein coupled receptors. This is predominantly because these ligands are relatively lipophilic and are capable of passively traversing the cell membrane and entering the cytosol to exert their effects. Ligands for enzyme-coupled receptors or GPCRs are either too large or polar and cannot cross the cell membrane.
For example, testosterone is a hormone ligand for the nuclear receptor, androgen receptor. Testosterone is comprised of a cyclopentaperhydrophenathrene ring (which is the same steroid nucleus as the common sterol, cholesterol) (Figure. 4.1). The overall structure is relatively nonpolar with the exception of two terminal oxygen atoms that cap each end of the molecule. This facilitates testosterone binding to its corresponding nuclear receptor, as the binding pocket is relatively hydrophobic with exception of the side chains of Arg752 and Thr877 making hydrogen bonding interactions with the carbonyl and hydroxyl functional groups of testosterone, respectively (de Jésus-Tran et al., 2006).
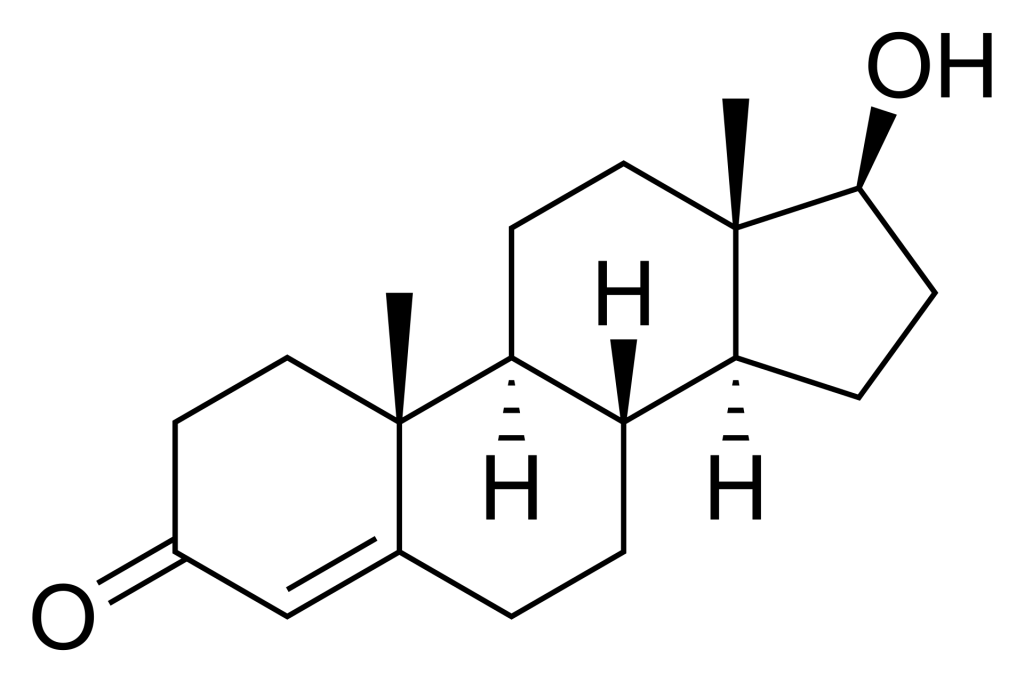
4.3.2 What is the general mechanism of action for nuclear receptors responding to ligands in a signalling pathway?
a. Nuclear receptor ligands operate in a manner similar to enzyme receptor ligands by binding in the extracellular region and exerting their effects
b. Nuclear receptor ligands cross the plasma membrane lipid bilayer, and bind receptor molecules forming a ligand-receptor complex, following entry into the nucleus
As mentioned previously, ligands that activate nuclear receptors are capable of traversing the cell membrane passively due to their lipophilic nature. Nuclear receptors are different from other receptors in that they are intracellular proteins (and not integrated into the cellular membrane). They are also known as transcription factors, because they will bind to DNA and directly facilitate transcription upon ligand binding. In fact, nuclear receptors represent the largest family of eukaryotic transcription factors (Mazaira GI, 2018).
4.3.3 What are the different types of nuclear receptors?
There are 48 nuclear receptors identified in humans (Weikum et al., 2018). Over the past 50 years, these receptors have been stratified into different categories based on historical identification, sequence similarity, ligand substrate response, dimerization, and DNA-binding elements. For example, nuclear receptors were originally classified into three different types (endocrine receptors, orphan receptors, and adopted orphan receptors), and there are also classifications based on sequence similarity that have led to six sub-families (Laudet, 1997).
Conventionally, the simplest classification lists two types of nuclear receptors – called Type I and Type II receptors. Type I receptors are located in the cytoplasm, and will translocate to the nucleus following hormone binding. Androgen receptors, progesterone receptors, and glucocorticoid receptors are examples of Type I receptors. Type II receptors are found in the nucleus and are bound to DNA even in the absence of ligand. Peroxisome proliferator-activated receptors (PPAR) and retinoic acid receptor (RAR) are examples of Type II receptors.
4.3.4 Describe the canonical domain structure of nuclear receptors.
Varying nomenclature has been employed for labelling nuclear receptors. In the most generic case, all nuclear receptors contain a region for DNA-binding and a region of ligand-binding. Based on the sequence, nuclear receptors are also labelled in regions labelled A – F (Figure 4.2). These regions contain specific domains that are important for activity. The A/B region is found at the N-terminus and is largely unstructured and contains the Activation Function-1 (AF-1). The C region contains a DNA-binding domain (DBD) which is important for binding specific sequences of DNA and initiating transcription. The D region serves as a connecting loop between the DBD and the E/F region and is sometimes called the hinge region. The E/F region is located the C-terminus and harbours the ligand-binding domain as well as the Activation Function-2 (AF-2) motif.
The DBDs enable for nuclear receptors to bind DNA at specific regions called response elements. The DBD also contains two zinc finger motifs. In addition to the different domains, nuclear receptors possess different NLSs identified in the D domain (hinge region) that facilitate cellular localization to the nucleus (Weikum et al., 2018).
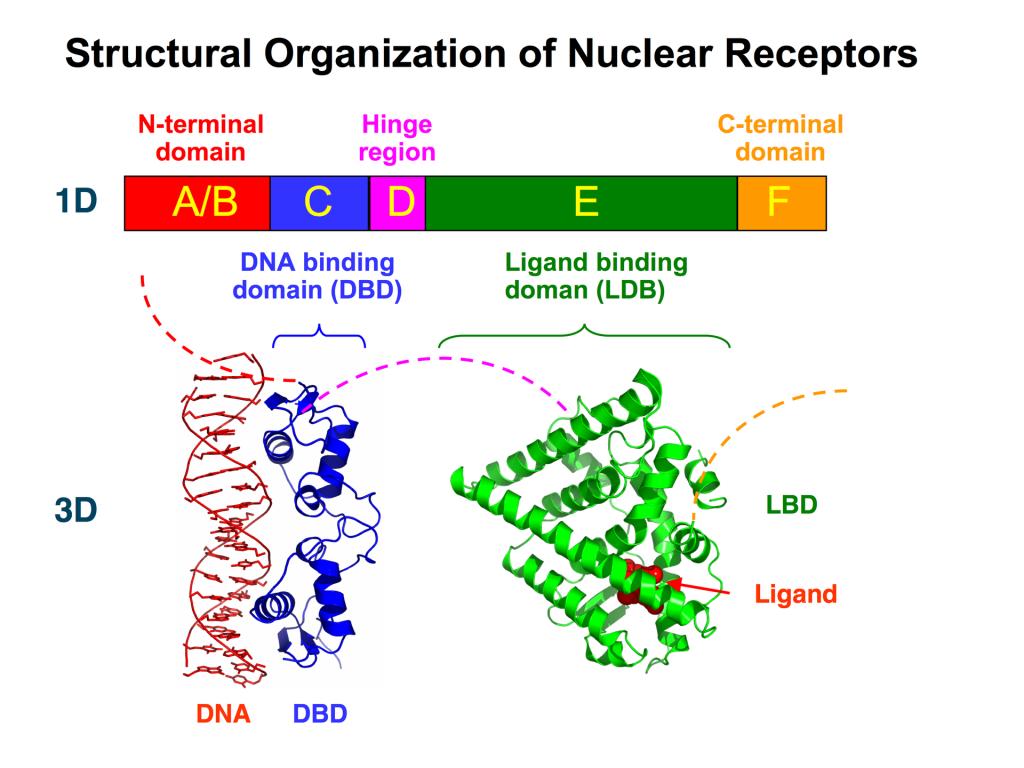
4.3.5 Where does the ligand bind nuclear receptors?
The ligands bind at the C-terminus of nuclear receptors at the E/F region or the ligand-binding domain (LBD). This triggers specific conformational changes throughout the ligand binding domain. In particular the LBD is composed of 12 α-helices along with a nonpolar pocket enabling the ligand to bind (Figure 4.2). The final helix (H12) is re-positioned to create a more condensed structure and surface pocket that enables interactions with the A/B region as well as other co-regulatory proteins. This also promotes dimerization of the nuclear receptor further reinforcing receptor activation.
4.3.6 Describe the hormone binding cycle for a Type I and Type II nuclear cycle.
For Type I receptors, ligand binding occurs (at the Ligand Binding Domain) in the cytosol following hormone diffusion across the cell membrane. The binding event triggers conformational changes that lead to dissociation of stabilizing proteins such as heat shock proteins which also enables dimerization in the cytosol. Dimerization is facilitated by both the DBD and the LBD. The AF-1 and AF-2 sites bind to other proteins that are recruited and help activate the nuclear receptor (for example SRC/p160 – p160 Steroid Receptor Coactivator family). The complex situated in the interior of the nucleus binds to hormone response elements to initiate transcription (Figure 4.3) (Feng & He, 2019).
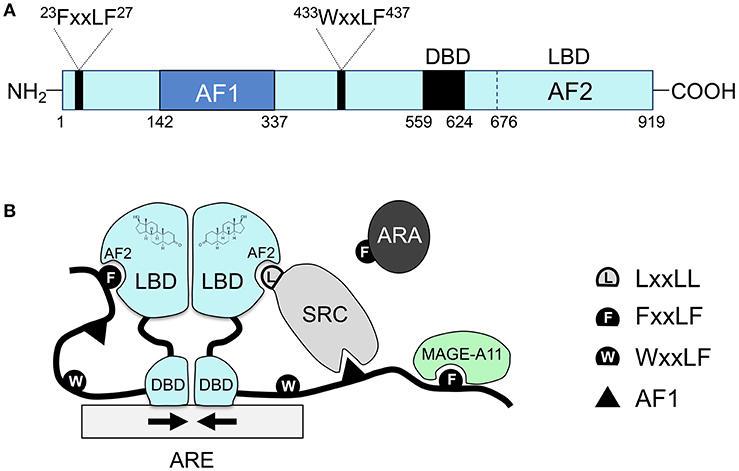
Type II receptors follow a similar pathway, except that the nuclear receptor is always bound to DNA and is usually in complex with a co-repressor protein (Sever & Glass, 2013). Binding of the ligand (e.g. hormone) liberates the release of the co-repressor, and assemblage of a co-activator and RNA polymerase which eventually leads to transcription of target proteins.
4.3.7 Which substrate binding has a greater affinity for a receptor?
Consider a hypothetical situation, where a nuclear receptor has different Kd (dissociation constant) values for different ligands.
a. Kd = 0.5 nM
b. Kd = 5 nM
c. Kd = 50 nM
The reaction of a protein (or receptor) with a ligand is given by the equation: P + L ↔ P-L.
The association constant (Ka) can be quantitatively expressed as the concentration of the products divided by the concentration of the substrates. However, the units of this reaction are M-1 which are challenging to intuitively compare. As such, dissociations constants are commonly used to describe different reactions and provide a value that can be readily interpreted. The value of Kd represents the concentration required for half of the receptor/protein to be saturated. Therefore a lower Kd represents a more potent compound, since a lower concentration would be required engage the target. Importantly, the dissociation constant is the inverse of the association constant. Therefore, in the above example, substrate with a Kd = 0.5 nM represents the highest affinity. Importantly, different substrates/ligands can have different Kd values for different receptors. For example, estriol (an estrogen analog) has about half the Kd for estrogen receptor alpha compared to estrogen receptor beta (Dahlman-Wright et al., 2003).
4.3.8 Which cytoskeletal elements enable transport of ligand bound nuclear receptors to the nucleus?
a. Microtubules
b. Microfilaments
c. Intermediate filaments
Cytosolic ligand-nuclear receptor complexes are transported to the nuclear pores using microtubules as the main transport pathway (Gomperts et al., 2009).
4.3.9 What are orphan nuclear receptors?
We have described different types of nuclear receptors. In the majority of these scenarios, both the ligand and receptor have been defined (for example, the steroid cortisol binds the glucocorticoid receptor). However, there are nuclear receptors where the ligand remains unknown. These nuclear receptors are characterized as orphan receptors yet potentially still impact transcription processes either activating or repressing it (Gomperts et al., 2009). If the ligand is discovered at a later date, these receptors are updated to “adopted orphan receptors” (Li et al., 2003). For example, the PPAR receptors (alpha, gamma, and delta) are all considered adopted orphan receptors since they were originally identified as critical proteins in cell metabolism. They have been identified to respond to a number of substrates (for example, PPARα is activated by free fatty acids), and they have important pharmacological effects in a number of diseases such as hyperlipidemia and diabetes.
4.3.10 Can phosphorylation regulate nuclear receptors?
Kinases have been shown to phosphorylate nuclear receptors and alter their activity. For example, PKA and MAPK can phosphorylate nuclear receptors, demonstrating the cross-talk between different biochemical pathways (Adams et al., 1997; Tzagarakis-Foster & Privalsky, 1998). While multiple different sites have been identified as phosphorylation targets across all of the domains of nuclear receptors, serine residues identified in the A/B domain are common targets.
4.3.11 Does phosphorylation of nuclear receptors promote or repress transcription?
The effect of phosphorylation may promote or repress transcription. In a situation where phosphorylation enables for coactivators to bind, transcription would be upregulated. However, transcription would be inhibited if phosphorylation prevents receptor dimerization (Dongsheng Chen Paul E. Pace & Ali, 1999). For example, phosphorylation of the adrenergic receptor by PKA promotes ligand-dependent and ligand-independent activation, whereas phosphorylation of the estrogen receptor alpha by PKA can reduce dimerization and DNA binding. However, phosphorylation of different sites on the estrogen receptor alpha by other kinases (such as MAPK) can promote activation. Therefore, the effects of phosphorylation of nuclear receptor activity are complex and challenging to predict.
4.3.12 Compare and contrast some of the properties of nuclear receptors from enzyme coupled receptors and GPCRs.
The are multiple different features that separate nuclear receptors from the other types of receptors. Some of the key points are listed below:
- Nuclear receptors are found in the cytosol or nucleus, whereas enzyme coupled receptors and GPCRs are found exclusively in the cell membrane.
- Nuclear receptors directly stimulate transcription and bind to DNA, whereas enzyme coupled receptors alter kinase activity, and GPCRs modulate enzymatic or transport function.
- Structurally, nuclear receptors have a monomeric structure with distinct ligand binding and DNA-binding domains that will either homo- or hetero- dimerize for activation. Enzyme coupled receptors have a single transmembrane helix embedded in the cell membrane that links a ligand binding domain to the intracellular kinase domain. GPCRs have seven transmembrane helices that link the ligand binding site to the G-protein coupled domain.
References
Adams, M., Reginato, M. J., Shao, D., Lazar, M. A., & Chatterjee, V. K. (1997). Transcriptional activation by peroxisome proliferator-activated receptor gamma is inhibited by phosphorylation at a consensus mitogen-activated protein kinase site. The Journal of Biological Chemistry, 272(8), 5128–5132. https://www.jbc.org/article/S0021-9258(19)79350-8/fulltext
Dahlman-Wright, K., Koehler, K., & Gustafsson, J.-Å. (2003). Estrogen Receptor-β Structure and Function. In H. L. Henry & A. W. Norman (Eds.), Encyclopedia of Hormones (pp. 599–608). Academic Press. https://doi.org/10.1016/B0-12-341103-3/00091-7
de Jésus-Tran, K., Côté, P.-L., Cantin, L., Blanchet, J., Labrie, F., & Breton, R. (2006). Comparison of crystal structures of human androgen receptor ligand-binding domain complexed with various agonists reveals molecular determinants responsible for binding affinity. Protein Science, 15(5), 987–999. https://doi.org/10.1110/ps.051905906
Dongsheng Chen Paul E. Pace, R. C. C., & Ali, S. (1999). Phosphorylation of Human Estrogen Receptor α by Protein Kinase A Regulates Dimerization. Molecular and Cellular Biology, 19(2), 1002–1015. https://doi.org/10.1128/MCB.19.2.1002
Feng, Q., & He, B. (2019). Androgen Receptor Signaling in the Development of Castration-Resistant Prostate Cancer. Frontiers in Oncology, 9. https://doi.org/10.3389/fonc.2019.00858
Gomperts, B. D., Kramer, I. M., & Tatham, P. E. R. (2009). Signal transduction . In Signal transduction (2nd ed.). Elsevier/Academic Press.
Laudet, V. (1997). Evolution of the nuclear receptor superfamily: early diversification from an ancestral orphan receptor. Journal of Molecular Endocrinology, 19(3), 207–226. https://doi.org/10.1677/jme.0.0190207
Lavery, D. N., & McEwan, I. J. (2005). Structure and function of steroid receptor AF1 transactivation domains: induction of active conformations. In Biochemical journal (Vol. 391, Issue Pt 3). Portland Press Ltd. https://doi.org/10.1042/bj20050872
Li, Y., Lambert, M. H., & Xu, H. E. (2003). Activation of Nuclear Receptors: A Perspective from Structural Genomics. Structure, 11(7), 741–746. https://doi.org/10.1016/S0969-2126(03)00133-3
Mazaira GI, Zgajnar NR, Lotufo CM, Daneri-Becerra C, Sivils JC, Soto OB, Cox MB, G. M. (2018). The Nuclear Receptor Field: A Historical Overview and Future Challenges. Nuclear Receptor Research. https://doi.org/10.11131/2018/101320
Sever, R., & Glass, C. K. (2013). Signaling by nuclear receptors. Cold Spring Harbor Perspectives in Biology, 5(3), a016709–a016709. https://doi.org/10.1101%2Fcshperspect.a016709
Tzagarakis-Foster, C., & Privalsky, M. L. (1998). Phosphorylation of Thyroid Hormone Receptors by Protein Kinase A Regulates DNA Recognition by Specific Inhibition of Receptor Monomer Binding. The Journal of Biological Chemistry, 273(18), 10926–10932. https://doi.org/10.1074/jbc.273.18.10926
Tzavlaki, K., & Moustakas, A. (2020). TGF-β signaling. Biomolecules (Basel, Switzerland), 10(3), 487-. https://doi.org/10.3390/biom10030487
Weikum, E. R., Liu, X., & Ortlund, E. A. (2018). The nuclear receptor superfamily: A structural perspective. In Protein Science (Vol. 27, Issue 11, pp. 1876–1892). https://doi.org/10.1002/pro.3496